ABSTRACT
Patterns of climatic variables are likely to change significantly in the coming century, with important but poorly-understood consequences for plant communities. This experiment determined how seeds of P. sylvestris collected from different elevations in two geographical regions performed when placed in simulated moisture stress conditions. Eight provenances from two regions (Norway and France) were chilled for zero and four weeks at 4±1°C and then germinated at 10 and 20°C, at water potentials of -0.3, -0.6 and -0.9 MPa. Moisture stress resulted in delayed germination and reduced germination capacity, and there was no germination at a water potential of -0.9 MPa. Provenances from high elevations were the most susceptible to moisture stress.
Key words: Moisture stress, water potentials, simulated moisture conditions, germination capacity, temperature effects.
Changes in climate are predicted to alter the geographic distributions of plant species, in part by affecting where individuals can establish from seed. Climate models predict that dry periods will become more common in Central Europe, the Western USA and East Asia (Eastaugh, 2011), and this may affect seed germination and seedling establishment. Germination begins with water uptake (imbibition), and imbibition rate is determined by the availability of water, the permeability of seed structures regulating the uptake of water, and conditions during hydration. Both factors can, separately or jointly, affect the germination capacity and germination rate (Baskin and Baskin, 1998). Each plant species appears to have its own specific range of water potential requirements, below and above which no germination occurs, and optimum water potential at which germination is fastest.
The values of water potential ranged from zero (pure water) to large negative numbers such as -100 MPa for air-dry seeds. Increasing osmotic and matric effects reduce the total water potential to lower (more negative) values. The water potential of seeds imbibed sufficiently for germination has generally been considered to be high. Accurate models are required to describe seed responses to temperature and water potential and make effective predictions of field germination. Bradford (1990) introduced the idea of hydrotime for explaining germination. Hydrothermal germination models offer a useful way of understanding why and how seed germination varies under different moisture and temperature conditions, and among different seed populations (Bloomberg et al., 2009). Germination of a given fraction of seed is prevented if water potential is below the base water potential for that fraction. Germination rate increases with an increase in the distance between base water potential and actual water potential up to the optimum water potential (Meyer and Allen, 2009).
One technique for studying the effect of water potential on germination is to simulate moisture stress conditions using solutions of variable concentrations of large molecules such as polyethylene glycol (PEG) (Falusi et al., 1983). Polyethylene glycol is an inert, water-binding polymer with a non-ionic and virtually impermeable long chain and is not toxic to plants (Amooey and Fazlollahnejad, 2014). Polyethylene glycol of high molecular weight (PEG 8000) is more appropriate than smaller molecules such as PEG 4000 for creating moisture stress, because germination capacities of seed in polyethylene glycol 8000 and in soil with the same water potential are approximately equal (Rana et al., 2017). Studies have demonstrated that significant differences exist among seed sources in germination ability under moisture stress levels. There have been few studies to determine if seeds collected from different environmental conditions, particularly from areas of high and low rainfall, demonstrate variation in moisture stress tolerance. The work described here shows the effects of moisture stress on germination of Pinus sylvestris provenances from four elevations in Norway and four in France.
Table 1 shows the geographic and climatic details for the eight provenances from two regions (Norway and France, wet and dry climates respectively) of Pinus sylvestris used in this study. The provenances were selected to represent an elevation range from two parts of the species’ range and seed was purchased from a government tree seed centre and a commercial tree seed supplier in Norway and France, respectively. Seeds of the France A3 provenance were collected from an elevation range that included the locations of the France A2 and A4 provenances.
Seed treatment: Target moisture content and chilling
At the start of experiment, all seeds to be chilled for any duration before germination testing were adjusted to a target moisture content of 30%. This is the moisture content at which seeds of P. sylvestris are optimally chilled (Afroze and O’Reilly, 2013). Target moisture content allows moist seeds to break dormancy and do not allow any radicle growth. The weight of water necessary to achieve this target moisture content was calculated using the following formula:
Where w = weight of water to add (g); s = dry weight of seed (g); m = moisture content (fresh weight basis) of seed prior to treatment; tmc = target moisture content (% fresh weight basis) (Jones and Gosling, 1994).
The moisture content of seeds was determined using the oven dry method. Once the target moisture content (30±2 %) was achieved the polythene bags containing the seeds were loosely tied to allow gas exchange but restrict water loss and transferred to a walk-in cold dark room set at 4±1°C for the required chilling duration. The seeds were inspected and gently shaken once in a week to allow uniform imbibition and air circulation (Afroze and O’Reilly, 2013).
Moisture stress treatments
Polyethylene glycol (PEG 8000) was dissolved in deionised water to make solutions with water potentials of -0.3, -0.6 and -0.9 MPa. The equation used to determine the amount of PEG to be used for a given water potential is:
Ψ = 1.29 × (PEG)2 × T – 140 × (PEG)2 − 4.0 × (PEG).
where Ψ = water potential; PEG = polyethylene glycol concentration (g l-1).
Deionised water (0.0 MPa) was used as the control. Water potentials of the solutions used in germination boxes were monitored by measuring samples of the solutions using a HR-33T Dew Point Microvoltmeter and Sample Chamber C-52 (Wescor model).
Experimental procedure and analysis
Two chilling durations (zero and four weeks) at one chilling temperature (4°C) were used in this experiment. Seeds were germinated at two temperatures in two germination chambers, one set at 10°C and the other 20°C. Each temperature had fifty seeds. Filter paper in germination boxes were moistened with 150 ml of the appropriate PEG 8000 solution or deionised water. PEG solutions at a germination temperature of 20°C were renewed weekly by pouring out the existing solution and adding 150 ml of fresh solution in every germination box. Germination boxes were inspected daily. Germinated seeds were counted and discarded immediately; this avoided any recounting of the same germinants. The final germination count was done on day twenty-one. Germination capacity and rate of germination were calculated using the following formulas:
Germination capacity: 100 × S/T
Where S is the cumulative number of germinated seeds at the end of the experiment and T is the total number of sown seeds.
Germination rate: (Σ Ni/Di)/S
Where Ni is the number of seeds that germinated on day Di. Statistical analyses were conducted at two levels. Firstly, differences in germination capacity and germination rate among seed sources and treatments (chilling temperatures and chilling durations) as factors were tested with a two-way ANOVA. None of the germination data from any of the experiments required transformation. Where differences among seed sources and / or treatments were significant (P < 0.05) a multiple comparison post hoc test was performed (Tukey test) to determine the significance of pairwise differences between means. Data analyses were conducted using Genstat (14th Edition, VSN International Ltd) and SPSS (20th Edition).
Norway: Germination capacity
Germination capacity was generally higher in seeds from low elevations, after chilling for four weeks at 4°C, at lower levels of water stress (water potentials of 0.0 and -0.3 MPa) and at 20°C. Without chilling and at 10°C, germination capacity ranged between 0 and 29%, and was highest in the lowest elevation (Norway A4) provenance. After four weeks chilling and at 10°C, germination capacity ranged between 0 and 78.5%, and highest germination capacity was in the second lowest elevation (Norway A3) provenance (Figure 1a and b). Without chilling and at 20°C, germination capacity ranged between 19 and 81.5%, and was highest in the two lower elevation (Norway A3 and A4) provenances. After four weeks chilling and at 20°C, germination capacity ranged between 30.5 and 96%, and was highest in the second lowest elevation (Norway A3) provenance (Figure 1c and d). Germination at a water potential of -0.6 MPa occurred only at 20°C (Figure 1c and d).
Germination decreased as moisture stress increased and was completely inhibited at a water potential of -0.9 MPa. Analysis of variance showed that there were significant (P <0.001) differences in germination capacity among provenances, chilling durations, moisture stress levels and germination temperatures (Table 2). There were significant interactions between all factors. The provenances were significantly different from each other, except for comparisons between Norway A3 and Norway A4 (Table 3).
Norway: Germination rate
Germination rate was generally higher in provenances from low elevations, after four weeks chilling at 4°C, at lower levels of moisture stress (water potentials of 0.0 and -0.3 MPa) and at 20°C. Without chilling and at 10°C, germination rate ranged between 0 and 0.7day-1, and was highest in the lowest elevation (Norway A4) provenance.
After four weeks chilling and at 10°C, germination rate ranged between 0 and 1.8 day-1, and was highest in the second lowest elevation (Norway A3) provenance (Figure 2a and b). Without chilling and at 20°C, germination rate ranged between 0.3 and 1.9 day-1, and was highest in the lower elevation (Norway A3 and A4) provenances. After four weeks chilling and at 20°C, germination rate ranged between 0.7 and 2.2 day-1, and was highest in the second lowest elevation (Norway A3) provenance (Figure 2c and d). Table 4 shows the results of analysis of variance of germination rate. Germination rate was significantly (P < 0.001) different among provenances, chilling durations, moisture stress levels and germination temperatures. There were significant interactions between all factors except between provenance and chilling and between provenance and temperature (Table 4). The provenances were significantly different from each other, except for comparisons between Norway A3 and A4 (Table 5).
Norway: Cumulative germination
There was no germination of any provenances in the -0.6 MPa moisture stress treatment (Figure 3). Germination curves differed chiefly in the time at which germination started. Without chilling, germination started at 19-20 days in the control (0.0 MPa) moisture stress treatment and at day 21 in the -0.3 MPa treatment (Figure 3a and b). After four weeks chilling, germination started at 11 to 13 days in the control (0.0 MPa) moisture stress treatment and at 15-18 days in the -0.3 MPa treatment (Figure 3c and d). The difference in the start of germination between moisture stress levels was greatest for the provenance from the lowest elevation (Norway A4).
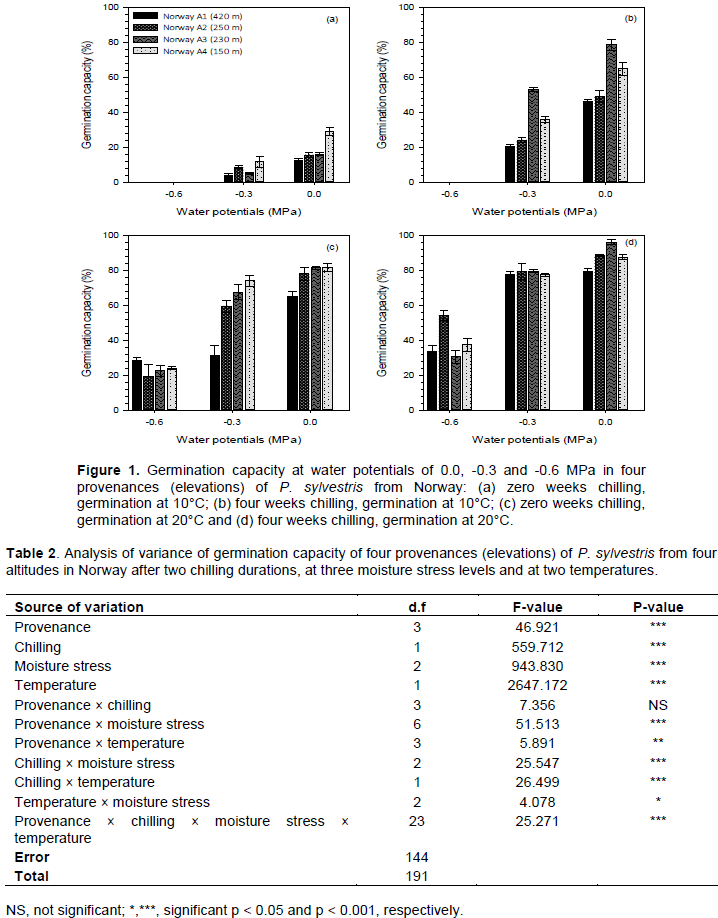
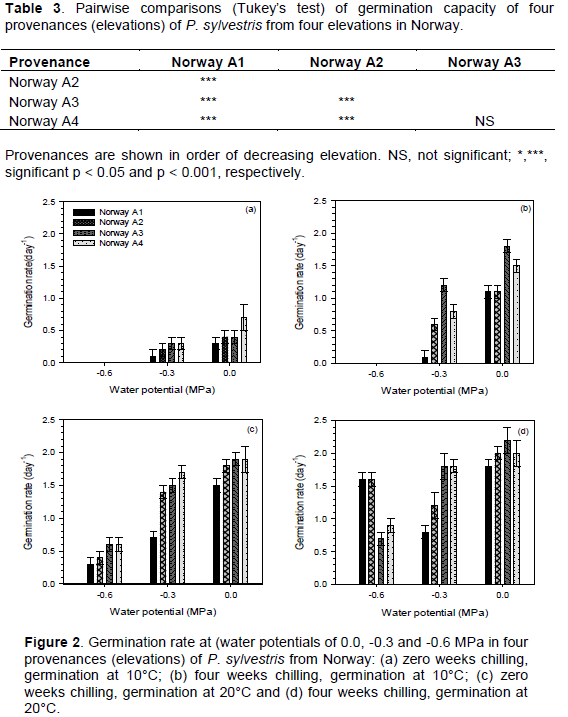
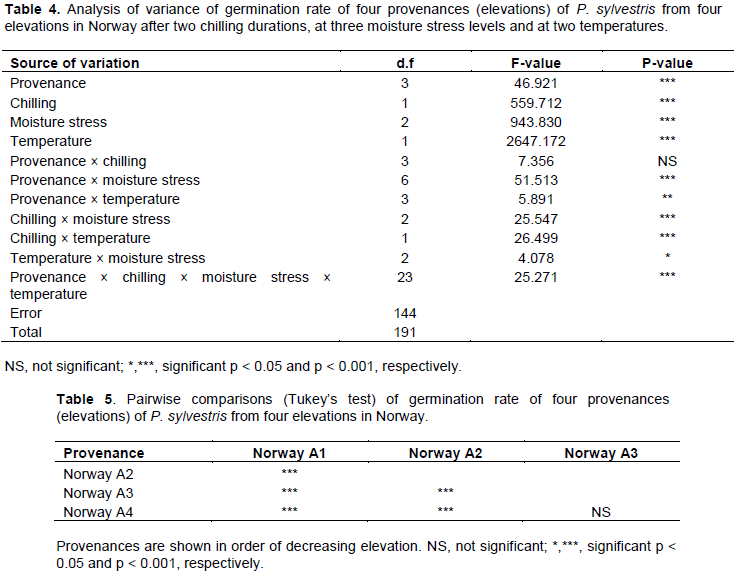
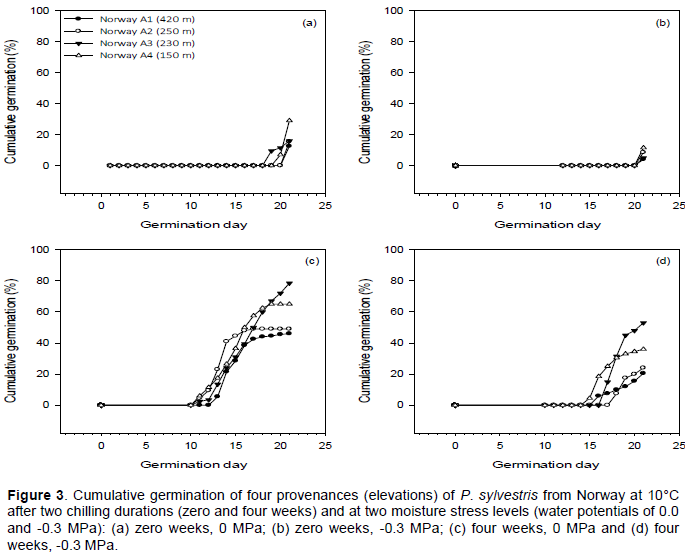
Figure 4 shows the cumulative germination of Norwegian provenances at 20°C after two chilling durations and at three moisture stress levels (water potentials of 0.0, -0.3 and -0.6 MPa). Without chilling, germination started at 5 to 7 days in the control (0.0 MPa) moisture stress level treatment, at 7 to 8 days in the -0.3 MPa treatment and at 16 to 18 days in the -0.6 MPa treatment (Figure 4a to c). After four weeks chilling, germination started at 3 to 4 days in the control, 4 to 6 days in the -0.3 MPa treatment and at 6 to 10 days in the -0.6 MPa treatment (Figure 4d and f). The difference in the start of germination between moisture stress treatments was greatest for the provenance from the lowest elevation (Norway A4).
France: Germination capacity
Germination capacity was generally higher in the provenances from lower elevations after chilling for four weeks at 4°C, at lower moisture stress levels (water potentials of 0.0 and -0.3 MPa) and at 20°C. Without chilling and at 10°C germination capacity ranged between 0 and 41%, and was highest in the lower elevation (France A3 and A4) provenances. After four weeks chilling and at 10°C, germination capacity ranged between 0 and 87.5%, and was also highest in the provenances from the lower elevation (France A3 and A4) (Figure 5a and b). Without chilling and at 20°C, germination capacity ranged between 0 and 94%, and was highest in the lower elevation (France A3 and A4) provenances. After four weeks chilling and at 20°C germination capacity ranged between 19.5 and 97.5%, and was again highest in the lower elevation (France A3 and A4) provenances (Figure 5c and d).
Germination at a water potential of -0.6 MPa only occurred at 20°C and there was very little germination without chilling. Germination decreased as moisture stress increased and was completely inhibited at a water potential of -0.9 MPa. Analysis of variance showed that there were significant (P < 0.001) differences in germination capacity among provenances, chilling durations, moisture stress levels, and germination tem-peratures (Table 6). There were significant interactions between all factors except between provenance and chilling and between provenance and temperature (Table 6).
The provenance from the highest elevation (France A1) was significantly different from the other three provenances, which were not significantly different from each other except between France A2 and France A4 (Table 7).
France: Germination rate
Germination rate was generally higher in provenances from lower elevations, after four weeks chilling at 4°C, at lower levels of moisture stress (water potentials of 0.0 and -0.3 MPa) and at 20°C. Without chilling and at 10°C germination rate ranged between 0 and 0.8 day-1, and was highest in the lower elevation (France A2, A3 and A4) provenances. After four weeks chilling and at 10°C germination rate ranged between 0.0 and 1.9 day-1, and was highest in the provenances from lower elevations (France A3 and A4) (Figure 6a and b). Without chilling and at 20°C, germination rate ranged between 0.0 and 2.1 day-1, and was highest in the provenances from the lower elevations (France A3 and A4). After four weeks chilling and at 20°C germination rate ranged between 0.4 and 2.2 day-1, and was also highest in the provenances from the lower elevations and the lowest elevation (France A3 and A4) provenances (Figure 6c and d). Germination rate was significantly (P < 0.001) different among provenances, chilling durations, moisture stress levels and germination temperatures (Table 8).
Results of pairwise comparisons between provenances are shown in Table 9. The provenance from the highest elevation (France A1) was significantly different from the other three provenances, which were not significantly different from each other except between France A2 and A4.
France: Cumulative germination
Without chilling, germination started at 16-18 days in the control (0.0 MPa) moisture stress level treatment and at 19-21 days in the -0.3 MPa treatment. The provenance from the highest elevation (France A1) did not germinate at all in the -0.3 MPa treatment (Figure 7a and b). After four weeks of chilling germination started at 7 to 10 days in the control treatment and at 12 to 16 days in the -0.3 MPa treatment (Figure 7c and d). The difference in the start of germination between moisture stress treatments was greatest for the provenance from the lowest elevation (France A4).
Cumulative germination of four provenances from France at 20°C after two chilling durations (zero and four weeks) and at three water stress levels (water potentials of 0.0, -0.3 and -0.6 MPa) is shown in Figure 8. Without chilling, germination started at 4 to 5 days in the control (0.0 MPa) treatment and at 6 to 9 days in the -0.3 MPa treatment (Figure 8a and b). Only the France A2 provenance germinated in the -0.6 MPa treatment, starting on day 11 (Figure 8c). After four weeks chilling, germination started at three days in the control, at 4-5 days in the -0.3 MPa treatment and at ten days in the -0.6 MPa treatment (Figure 8d to f). There were no obvious differences in response to moisture stress levels among provenances.
The results reported here clearly demonstrate both variation in germination behaviour among provenances from different elevations and the effect of moisture stress on germination of seeds. Seeds from lower elevations had a higher germination capacity and rate than those from higher elevations, as observed for seeds of some high-elevation woody species studied by Meyer and Monsen (1991). Seeds from low elevations may germinate more rapidly and have a higher germination capacity because they are less dormant than seeds from high elevations (Pratap and Sharma, 2010). However, according to Fernández-Pascual et al. (2013), seeds from high elevations may germinate better and faster because of their reduced dormancy compared with seeds from low
elevations, as is the case for Calluna vulgaris, Erica cinerea and Erica vagans. Additionally, Vera (1997) argued that seeds from higher elevations might receive more chill units than seeds from lower elevations due to the decrease in temperature as elevation increases, resulting in faster germination and higher germination capacity. Vera’s (1997) contentions are not in agreement with findings of this experiment, in which higher-elevation provenances showed delayed and poorer germination than lower-elevation provenances. These results agree with the findings of Falusi et al. (1983), who reported a decrease in germination capacity of Pinus brutia seed with increases in moisture stress. Falusi et al. (1983) further observed that in Pinus halepensis an increase in moisture stress to -0.2 MPa lowered germination capacity considerably, while at a water potential of -0.8 MPa germination was reduced to approximately 25%. Ne’eman et al. (1999) showed that germination of Rhus coriaria was reduced by 80% at a water potential of -0.26 MPa. In studies on Pinus nigra by Topacoglu et al., (2016), increasing water stress also reduced germination, while Zhu et al. (2006) reported that increasing water stress decreased germination rate in Pinus sylvestris. Gholami et al. (2010) obtained similar results for four wild almond species and concluded that PEG prevents water uptake by seed tissues. According to Ahmadloo et al. (2011), the major reason for reduced and delayed germination under conditions of moisture stress is an increase in the length of the lag phase between imbibition and radicle emergence, since the increase in seed water
content proceeds slowly during this period. However, at 20°C and a water potential of -0.3 MPa, most (> 70%) of the seeds in the current experiment germinated, showing that seeds can germinate when subjected to some moisture stress.
Research by Mataruga et al. (2010) on five provenances of Pinus nigra showed that seeds coming from harsher environments took up lower amounts of water than those coming from environments that are more suitable for the species, suggesting local adaptation and the development of well-adapted land races. However, during the germination stages high-elevation provenances are vulnerable to changes in the physical environment, and selection by soil moisture may be important. High-elevation provenances typically germinate soon after snowmelt in the year after seed production (Körner, 2013). Following germination early in the growing season, seedlings are more likely to experience favourable soil moisture levels and temperatures, and a growing season long enough to allow accumulation of reserves before the onset of winter. Intraspecific differences in moisture stress tolerance may be associated with environmental differences among sites where populations are found, and are often interpreted in terms of responses to ecological factors (Tilki and Huseyin, 2007). The growing season is shorter and temperatures are lower at high elevations than at low elevations. Faster germination may facilitate the emergence, establishment, growth and survival of seedlings at high elevations (Mondoni et al., 2012). Higher germination at 20°C than at 10°C favours germination late in spring, when temperatures are higher and there is a lower probability of late-season frosts that could damage new seedlings. Pre-chilled seeds from low-elevation provenances do not show much difference in germination between 20 and 10°C, suggesting that seeds from lower-elevation provenances could germinate at lower temperatures, resulting in earlier seedling establishment and allowing the development of a root system appropriate for surviving summer drought conditions. Earlier germination over a wider range of environmental conditions may confer a greater chance of successful establishment of natural regeneration. Conversely, slow germination could help seeds avoid short periods favorable for germination within a long period unsuitable for seedling growth. Additionally, seeds that do not germinate in the presence of water stress can help the population to spread germination over the year.
Numerous studies have shown that germination patterns reflect the seasonal and climatic environments which occur naturally during the germination period (Dwyer and Erickson, 2016). The higher germination capacity shown by provenances of P. sylvestris from low elevations seems to be adaptive. At low elevations winters are relatively mild, with short periods of snow cover. Hence, the main risk of seedling death is from moisture stress as summer progresses. Seeds of all provenances, whether chilled or not, reached a very low germination capacity when incubated at 10°C, possibly because this temperature is close to base temperature and the thermal time accumulated by the end of the incubation period was not enough to permit the germination of most of the provenances. Additionally, the low germination capacity may suggest that seeds had not completely broken their primary dormancy. Reduction in germination with increased moisture stress suggests that moisture levels in the seeds were below the base water potential, which may lead to degradation and inactivation of essential hydrolytic and other groups of enzymes (Wilson, 1971). These results are consistent with suggestions that when water potential is reduced, germination will be delayed or prevented depending on the extent of its reduction (Boydak et al., 2003). The results revealed some differences, which were not tested statistically, between seeds from Norway and France. Germination of both Norwegian and French seeds tended to decrease with an increase in elevation of provenance. At the highest moisture stress level at which germination occurred (-0.6 MPa), Norwegian provenances were more tolerant than French provenances. These findings suggest that in drier climates the chances of seedling survival are increased by delaying germination until suitable moisture conditions are present for seedling establishment. They also suggest that germination of France provenances is more sensitive to declining moisture levels than germination of Norwegian provenances. Germination success of provenances from higher elevations in France was significantly reduced at high moisture stress levels. This is concerning, as climate change predictions are for drier conditions at higher elevation locations in France in the future, suggesting that natural regeneration may be at risk. However, according to Holtmeier and Broll (2007), pine species are migrating to higher elevations and pine recruitment around old seed trees appears to be promoted by climatic warming. The importance of water, temperature and their interaction on P. sylvestris germination is clear. Low germination at 10°C suggests that relatively high temperatures may be critical for success of germination during periods of low water potential, for example late spring and early summer. This experiment has demonstrated that P. sylvestris provenances from wet and dry regions (Norway and France) can germinate at various temperature and moisture stress levels, but that a temperature of 20°C and water potentials below -0.9 MPa (for Norwegian provenances) and -0.6 MPa (for provenances from France) are needed to reach high levels of germination. The low elevation provenances (Norway A3 and A4, France A3 and A4) were the most moisture-stress-tolerant provenances. The highest-elevation provenances Norway A1 and France A1 were the least tolerant of moisture stress. Seeds from low elevations which have high tolerance of moisture stress play an important role in the regeneration of this species in a future with decreased amounts of precipitation. In order to refine the selection of provenances offering the best potential under these conditions, it would be useful to continue investigations on the tolerance of moisture stress to the seedling stage, since establishment also depends on the ecological conditions prevailing at the time of seedling emergence.
Germination is one of the most critical periods in the life cycle of plants. Under water stress, low water potential is a determining factor inhibiting seed germination. Water stress delays the beginning of germination of P. sylvestris seeds in relation to the control treatment. Provenances from high elevations were the most susceptible to moisture stress. These results provide useful data that will facilitate the development of strategies for the selection of provenances that are more tolerant towards water stress in a changing climate.
The author has not declared any conflict of interest.
REFERENCES
Afroze F, O'Reilly C (2013). Breaking seed dormancy in European rowan seeds and its implications for regeneration. New Forests 44:547-557
Crossref
|
|
Ahmadloo F, Tabari M, Behtari B (2011). Effect of drought stress on the germination parameters of Cupressus seeds. International Journal of Forest, Soil and Erosion 1(1):11-17.
|
|
|
Amooey AA, Fazlollahnejad M (2014). Study of Surface Tension of Binary Mixtures of Poly (Ethylene Glycol) in Water and Poly (Propylene Glycol) in Ethanol and its Modeling Using Neural Network. Iranian Journal of Chemical Engineering 11(1):19-29.
|
|
|
Baskin CC, Baskin JM (1998). Ecology of seed dormancy and germination in grasses. In. Cheplick GP (Eds.), Population biology of grasses. Cambridge: Cambridge University Press pp. 30-83.
Crossref
|
|
|
Bloomberg M, Sedcole JR, Mason EG, Buchan G (2009). Hydrothermal time germination models for radiata pine (Pinus radiata). Seed Science Research 19(3):171-182.
Crossref
|
|
|
Boydak M, Huyesin D, Tilki F, Calikoglu M (2003). Effects of moisture stress on germination in six provenances of Pinus brutia seeds from different bioclimatic zones in Turkey. Turkish Journal of Agriculture and Forestry 27(2):91-97.
|
|
|
Bradford KJ (1990). A water relations analysis of seed germination rates. Plant Physiology 94(2):840-849.
Crossref
|
|
|
Dwyer JM, Erickson TE (2016). Warmer seed environments increase germination fractions in Australian winter annual plant species. Ecological Society of America 7(10):e01497
Crossref
|
|
|
Eastaugh CS (2011). Green philosophies in the face of climate change. Journal of Ecosystems and Management 11(3):34-44.
|
|
|
Falusi M, Calamassi R, Tocci A (1983). Sensitivity of seed germination and seedling root growth to moisture stress in four provenances of Pinus halepensis. Silvae Genetica 32(1/2):4-9.
|
|
|
Fernández-Pascual E, Jiménez-Alfaro B, Caujapé-Castells J, Jaén-Molina R, Diaz T (2013). A local dormancy cline is related to the seed maturation environment population genetic composition and climate. Annals of Botany 112(5):937-945.
Crossref
|
|
|
Gholami M, Rahemi M, Kholdebarin B (2010). Effect of drought stress induced by polyethylene Glycol on Seed Germination of four wild almond species. Australian Journal of Basic and Applied Sciences 4(5):785-791.
|
|
|
Holtmeier FK, Broll G (2007). Treeline advance driving processes and adverse factors. Landscape Online 1(3):1-33.
Crossref
|
|
|
Jones SK, Gosling PG (1994.) Target moisture content prechill overcomes the dormancy of temperate conifer seeds. New Forestry 8(4):309-321.
|
|
|
Körner C (2013). Alpine Plant Life: Functional Plant Ecology of High Mountain Ecosystems. Switzerland. Springer pp. 12-54.
|
|
|
Mataruga M, Haase DL, Isajev V (2010). Dynamics of seed imbibition and germination of Austrian pine (Pinus nigra) from extreme habitat conditions within five Balkan provenances. New Forestry 40(2):229-242.
Crossref
|
|
|
Meyer SE, Allen PS (2009). Predicting seed dormancy loss and germination timing for Bromus tectorum in a semi-arid environment using hydrothermal time models. Seed Science Research 19(4):225-239.
Crossref
|
|
|
Meyer SE, Monsen SB (1991). Habitat-correlated variation in mountain big sagebrush (Artemisia tridentata) seed germination patterns. Ecology 72(2):739-742.
Crossref
|
|
|
Mondoni A, Rossi G, Orsenigo S, Probert RJ (2012). Climate warming could shift the timing of seed germination in alpine plants. Annals of Botany 110(1):155-164.
Crossref
|
|
|
Ne'eman G, Henig-Sever N, Eshel A (1999). Regulation of the germination of Rhuscoriaria, a post-fire pioneer, by heat, ash, pH, water potential and ethylene. Journal of Plant Physiology 106(1):47-52.
Crossref
|
|
|
Pratap V, Sharma YK (2010). Impact of osmotic stress on seed germination and seedling growth in black gram (Phaseolus mungo). Journal of Environmental Biology 31(5):721-726.
|
|
|
Rana MS, Hasa MA, Bahadur MM, Islam MR (2017). Effect of Polyethylene Glycol Induced Water Stress on Germination and Seedling Growth of Wheat (Triticum aestivum). A Scientific Journal of Krishi Foundation 15(1):81-91.
Crossref
|
|
|
Tilki F, Huseyin D (2007). Seed germination of three provenances of Pinus brutica as influenced by stratification, temperature and moisture stress. Journal of Environmental Biology 28(1):133-136.
|
|
|
Topacoglu O, Sevik H, Akkuzu E (2016). Effects of water stress on germination of Pinus nigra arnold seeds. Pakistan Journal of Botany 48(2):447-453.
|
|
|
Vera ML (1997). Effects of altitude and seed size on germination and seedling survival of heathland plants in north Spain. Plant Ecology 133(1):101-106.
Crossref
|
|
|
Wilson AM (1971). Amylase synthesis and stability in crested wheat grass seeds at low moisture stress. Journal of Plant Physiology 48:541-546.
Crossref
|
|
|
Zhu J, Kang H, Tan H, Xu M (2006). Effects of drought stresses induced by polyethylene glycol on germination of Pinus sylvestris seeds from natural and plantation forests on sandy land. Journal of Forest Research 11(5):319-328.
Crossref
|
|