ABSTRACT
The bacterial heat shock response is characterized by the elevated expression of a number of chaperone complexes including the GroEL and the rate change of synthesis of certain proteins (total and secreted). In this work, after incubation at 45°C, total and secreted proteins profiles of stressed bacteria were found to be altered when analyzed by sodium dodecyl sulfate-polyacrylamide gel electrophoresis (SDS-PAGE). In addition, the level expression of GroEL was evaluated with Western blot. Our results show a marked increase in both GroEL expression and protein synthesis at 45°C. These modifications were manifested by the appearance and/or disappearance of bands as well as in the level of expression of certain proteins.
Key words: Lactobacillus casei, heat shock, GroEL, secreted and total proteins, sodium dodecyl sulfate-polyacrylamide gel electrophoresis (SDS-PAGE), Western blotting.
The viability of probiotic in foods depends on various factors during processing and storage. Heat is used in the process of a lot of foods, so the survival of probiotics in thermal processing is the main obstacles to food manufacturers (Mansouripour et al., 2013). Thus, paying special attention to the effect of heat shock on the survival of probiotics is necessary. For an organism to grow at high temperatures, especially as high as those of the hyperthermophiles discussed here, all cellular components, including proteins, nucleic acids and lipids, must be heat protected. Desmond et al. (2004) showed that the mechanisms involved in thermoprotection of Lactobacillus paracasei NFBC338 are probably controlled at the protein synthesis level. It has been previously reported that stress tolerance in Lactobacillus delbrueckii ssp. bulgaricus induced by a moderate heat shock was dependent on protein synthesis (Gouesbet et al., 2002) and Whitaker and Batt (1991) demonstrated the enhanced synthesis of a number of heat shock proteins, including GroEL and DnaK in a heat-adapted culture of Lactococcus lactis ssp. lactis. In addition, destabilization of macromolecules as ribosomes and RNA, and alterations of membrane fluidity were also described (Earnshaw et al., 1995; Teixera et al., 1997; Hansen et al., 2001). The heat-shock response has been studied notably in Escherichia coli (Gram negative) and Bacillus subtilis (Gram positive). Physiological studies demon-strated that lactobacilli elicit heat-shock responses similar to that of other Gram positive bacteria: L. lactis (Whitaker and Batt, 1991; Auffray et al., 1992; Kilstrup et al., 1997), Leuconostoc mesenteroides (Salotra et al., 1995), Enterococcus faecalis (Flahaut et al., 1996), Oenococcus oeni (Guzzo et al., 1997) and L. bulgaricus (Gouesbet et al., 2002). In order to understand the mechanisms of stress tolerance of lactobacilli, numerous studies have examined the physiological and genetic adaptations of these organisms during growth and survival in diverse environmental stresses (Corcoran et al., 2008; Spano and Massa, 2006; Van de Guchte et al., 2002) and postgenomic approaches have accelerated the understanding of the global (genome-wide) stress responses in lactobacilli to acid, lactate, oxidative, bile and heat stresses (Broadbent et al., 1998; Stevens, 2008; Serrano et al., 2007; Bron et al., 2006; Pieterse et al., 2005; De Angelis et al., 2004). These studies have shown that lactobacilli respond rapidly to their environ-ment by modulating expression levels of genes involved in different cellular processes, including stress response pathways, cell division, transport and cell envelope composition. However, the proteins synthesized by the stressed cells during the stationary phase were necessary for maintaining of the viability during prolonged stress (Kolter et al., 1993). These proteins enable the cell to neutralize stress to adapt or repair damages caused by stress (Hecker et al., 1996). Examination of lactobacilli heat shock responses using bi-dimensional electro-phoresis revealed variable numbers of induced proteins: 34 in E. faecalis (Flahaut et al., 1996), 17 in L. lactis (Kilstrup et al., 1997) and 40 in Streptococcus mutans (Svensater et al., 2000), among them, only six proteins were specifically up regulated by heat (Svensater et al., 2000). In L. lactis, the 12 proteins were induced by NaCl and detected on bi-dimensional electrophoresis, all belong to the heat shock stimulon (Kilstrup et al., 1997). This striking overlap between heat-shock and osmotic-stress responses may also exist in S. mutans as 21 heat-inducible proteins also belong to the osmotic stress response. Among the heat shock proteins, well conserved chaperones (DnaK, DnaJ, GrpE, GroES and GroEL) and proteases (Clp, HtrA and FtsH) have often been identified.
Some of the most intensively investigated heat shock proteins include the molecular chaperones GroEL and GroES, which are highly conserved in E. coli and eukaryotic cells (Gupta, 1995). The GroEL and GroES chaperones (also known as Hsp60 and Hsp10 chaperones) have been recognized as heat shock proteins in many bacteria, including E. coli, B. subtilis (Hecker et al., 1996), Agrobacterium tumefaciens (Segal et al., 1996), Streptomyces lividans (De Leon et al., 1997), L. lactis (Kilstrup et al., 1997), Lactobacillus helveticus (Broadbent et al., 1998), Pseudomonas aeruginosa (Fujita et al., 1998) and Lactobacillus johnsonii VPI 11088 (Walker et al., 1999).
In the present work, we focused on the effect of heat stress on GroEL expression of two strains of Lactobacillus and their influence on secreted and total proteins profiles of stressed cells. The cells were analyzed by sodium dodecyl sulphate (SDS)-polyacrylamide gel electrophoresis (PAGE).
Bacterial strain and growth conditions
Two strains of Lactobacillus casei were used in this study: S1, L. casei (ATCC 393); S2, L. casei, a potential probiotic, obtained from the laboratory collection; this strain was identified by sequencing the 16S rRNA gene (BankIt1773923 BL2 KP123430). All strains were stored on De Man, Rogosa, Sharpe (MRS; LAB M, Bury, UK) broth added with 33% of sterile glycerol at -20°C. Working cultures were grown at 37°C in modified MRS broth at pH of 6.4 for 24 h, which contained 0.5% maltose, 1% peptone, 0.5% yeast extract, 1 ml Tween 80, 0.2% K2HPO4, 0.2% ammonium citrate, 0.02% MgSO4 and 0.005% MnSO4. The pH of the modified MRS was 6.4 and, unless otherwise stated, it was maintained constant by the on-line addition of 1 M NaOH. In this study, the cells were grown at 37°C on MRS for 24 h.
Stress conditions
Cells were recovered by centrifugation and re-suspended to an optical density at 600 nm of 0.5 immediately before heat shock treatment at 45, 55, and 65°C. One culture (1 ml) was maintained at 37°C as a control, and three cultures were shifted to 45, 55 and 65°C. After 10 min, heat shock was stopped by placing samples on ice for 5 min.
Determination of the lethal temperature of Lactobacillus
100 µl of a bacterial suspension was inoculated with an optical density at 595 nm of about 0.6 prepared from a bacterial culture in MRS incubated overnight. These bacterial suspensions were treated at temperatures ranging from 45 to 95°C with an interval of 10°C for 10 min. All suspensions were prepared ??at the same time; one sample of each is removed after every increase of 10°C. All experiments were performed in triplicate.
Enumeration of cells
To determine the number of cultivable cells, decimal dilutions were performed in series in sterile saline solution. A volume of 0.1 ml of each dilution was then spread on the surface of three plates of MRS at 37°C. After 24-48 h of incubation, the enumeration of CFU/g of the appropriate dilution were made. All experiments were performed in triplicate.
After incubation and enumeration, we observed temperatures for which a bacterial growth is possible. Thus, we performed the heat shock at three temperatures: 45, 55 and 65°C.
Total protein extraction
Total proteins of two strains of Lactobacilli were prepared according to the method described previously (Sabri et al., 2000). Cultures of 24 h at 37°C were centrifuged (7000 ×g for 10 min at 4°C) and the cells were then washed three times in 40 ml of sterile saline solution (0.9% NaCl) and resuspended in 5 ml of sterile saline solution. Cells were disrupted by lysosyme solution (10 mg/ml) and incubated at 37°C for 30 min. 30 μl of loading buffer was added to the solution and the pellet was incubated in a water bath at 100°C for 5 min. Supernatant was collected and further centrifuged at 100,000 ×g for 40 min at 4°C. The concentration of the total proteins in the final preparation was determined using the Bradford assay (Bradford, 1976).
Secreted protein extraction
Cultures of 24 h at 37°C were centrifuged (7000 ×g for 20 min) and subsequently filtered through a 0.45-µm pore-size filter. Proteins from the cell-free culture supernatants were then precipitated by addition of 10% (vol/vol) trichloroacetic acid and recovered by centrifugation at 7000 ×g for 20 min. Pellets were resuspended in 4 ml of phosphate-buffered saline (PBS), and protein were precipitated by addition of 20 ml of cold acetone. After centrifugation at 7000 ×g for 20 min, the pellets were washed once with cold acetone, air-dried and re-suspended in 25 µl of PBS. The concentration of the secreted proteins in the final preparation was determined using the Bradford assay (Bradford, 1976).
Sample preparation and SDS-PAGE
Protein pellet were denatured with sample containing β-mercaptoethanol (Sigma, Chemical Co., St Louis, MO, USA). These protein samples were subjected to SDS-PAGE analysis using a Mini Protean 3 gel Unit (BIORAD; Richmond, CA) on 15% (w/v) polyacrylamide gels (Laemmli, 1970). Wide range protein markers (from 212 to 6.5 kDa) were used as molecular weight standards (High-Range Rainbow; Amersham, Little Chalfont, Buckinghamshire, UK). Proteins were visualized by Biosafe colloidal Coomassie blue (Sigma, Chemical Co., St Louis, MO, USA) according to the manufacturer's instructions.
Western blotting
20 µg of total proteins were analyzed by SDS PAGE as reported above and blotted onto a nitrocellulose membrane (Hybond ECL, Amersham), using the Mini Trans-Blot equipment (BioRad Inc.), at 90 mA, 4°C, for 16 h in transfert Buffer. The membrane was incubated in TBST buffer (20 mM Tris base, 137 mM NaCl, 0.15% Tween 20) + nonfat dry milk 4% for 1 h at room temperature, with orbital shaking, then incubated in rabbit polyclonal anti-GroEL antibodies (Abcam) diluted 1:10000 in blocking buffer, at room temperature for 1 h. After three washes with TBST, the membrane was incubated with a 1:10000 dilution of the secondary antibody conjugated with horseradish peroxidase (GE Healthcare), in blocking buffer at room temperature for 1 h. After three washes with TBST, the bound antibodies were revealed with revelation solution (tris HCl pH 9, 0.1 M MgCl2, 1 M Nacl, NBT, BCIP).
Statistical analysis
The statistical analysis was performed on SPSS v.17.0 statistics software. The statistical differences and significance were assessed by ANOVA test. P < 0.05 was considered significant.
Effect of temperature on survival of Lactobacillus
The survival of L. casei at different temperatures was investigated. Our results show that the two strains used remained cultivable up to 65°C. The viability was studied for an initial population of about 108 CFU/g. It was shown that both strains can survive at 75°C for 10 min (Figure 1). Remarkabely, at 45°C, we observed an increase of the optical density of the culture (Figure 1).
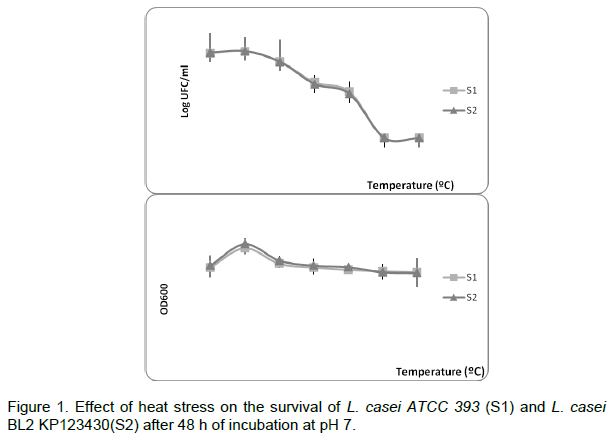
Analysis of total proteins
Total protein of Lactobacilli strains before and after heat shock were analyzed by SDS-PAGE. They displayed different profiles before and after exposure of heat shock. Before heat stress, the strains S1 and S2 of L. casei had the same total protein profile. Five clear bands were detected in each profile at 27, 35, 38 43, 90 kDa (Figure 2). After heat stress, some modifications were observed in total protein profile in both strains. However, at 45 and 55ºC, the expression of band corresponding to protein of 35 kDa was significantly decreased. Interestingly, bands corresponding to molecular weights of 38 and 43 kDa were significantly slightly increased at 55°C (Figure 2). At 65°C, the protein synthesis was completely stopped; all bands were not visible after heat shock to this temperature for 10 min.
Analysis of secreted proteins profiles
Secreted protein of Lactobacilli strains displayed different profiles before and after exposure to heat shock (Figure 3). Lactobacillus presents five or six major proteins in their profiles. After heat stress, both strains have a different extracellular protein profile, whereas, bands corresponding to protein of molecular weight of 65 kDa were not visible at 55 and 65°C for S2. In comparison, the intensity of this band for S1 was increased in intensity after heat stress. In addition, we have observed the level intense of one band corresponding to molecular weight of 35 kDa for S2. Interestingly, bands corresponding to molecular weights of 25 and 27 kDa approximately were slightly increased at 55 and 65°C.
GroEL expression
As shown in the Figure 4, the expression of protein was greater at 45°C (column S1i and S2i) and the higher levels were found in both strains at 55°C. However, the proteins were not visible at 65°C (Figure 4).
The results show that Gram positive bacteria, such as Lactobacilli, are able to survive heat shock up to 75°C. In addition, we found that L. casei cells remained cultivable at temperature of 65°C, which demonstrates that bacteria are able to withstand such adverse environments (Van de Guchte et al., 2002). It has been shown that organisms respond to environmental stress by modifying the rate of synthesis of certain proteins (Hennequin et al., 2001). In our study, we have shown that the heat shock induced alterations of secreted and total proteins and induced elevated GroEL expression. The marked increase on both GroEL expression and protein synthesis may suggest that Lactobacillus species are able to grow and survive under suboptimal conditions during food and beverage fermentations. Desmond et al. (2004) revealed by 2D-PAGE that the chaperone protein GroEL was among the most strongly expressed proteins in the cell under heat adaptation conditions; indeed, densitometry analyses indicated an approximately 50-fold increase in cells that were pre-adapted to heat shock. Kim et al. (2001) demonstrated that the transcriptional activity of both chaperones is increased dramatically in response to heat shock and was increased to a lesser extent by ethanol stress. Other studies have shown that culture of log-phase Lactobacillus cultures were subjected to heat stress, a 15-fold increase in GroEL synthesis was observed when compared with only 1.5-fold increase in protein synthesis in stationary phase cultures (Prasad et al., 2003).
These chaperones play a key role in the maturation of synthesized proteins and are pivotal in the degradation or refolding of denatured proteins (Rechinger et al., 2000). Thus, these heat-shock proteins can also be induced by multiple stresses, such as acid, heat, bile salts, high pressure stress and so on (De Angelis et al., 2004). Several studies have been done in the elucidation of the major chaperones belonging to the Hsp60 (GroEL) and Hsp70 (DnaK) families because protein folding has been recognized as one of the central problems in biology (Narberhaus, 2002). The study of GroESL-overproducing L. lactis and L. paracasei NFBC 338 demonstrated that technologically sensitive cultures can be potentially manipulated to become more robust for survival under harsh conditions, such as food product development and gastrointestinal transit (Desmond et al., 2004). The main function of these HSPs appeared to be the prevention of the accumulation of unfolded protein intermediates during periods of stress (Veinger et al., 1998). Although, the HSPs are always constitutively expressed, the rate of synthesis is significantly enhanced under stress (Ang et al., 1991).
The production of the HSPs is the origin of the beneficial effect of heat stress in the conservation of Lactococcus. Indeed, the physiological and biochemical riposte of the unicellular organisms to all occasional thermal elevation is universal. Some works have been done on L. lactis (Boutibonnes et al., 1995) which shows that the temporary exhibition of the cells to a temperature of 40-45°C provoked a disturbance followed by an adjustment of the metabolism. Thus, production of the majority of the usual proteins in stalled synthesis of HSP is unregulated. It has been demonstrated for E. coli, that a thermal treatment of 8 h has a beneficial effect on the cryodessiccation and the lyophilization (Joe et al., 2000).
In conclusion, our data suggest that overexpression of stress-induced proteins has the potential to improve the performance of lactobacilli strains. In particular, the GroES/EL chaperone complex can be exploited to prepare Lactobacillus for industrial processes. Indeed, the innate probiotic characteristics of the strain, such as adherence to the host cell wall and acid tolerance during gastric transit, may also be improved by the overexpression of GroESL.
The authors did not declare any conflict of interest.
The authors are grateful to Pr. Gaspar Perez Martinez [Laboratory of Lactic Acid Bacteria and Probiotics of the Institute of Agro-chemistry and Food Technology (Spanish National Research Council] for sequencing the 16S rRNA gene of L. casei.
REFERENCES
Ang D, Liberek K, Skowyra D, Zylicz M, Georgopoulos C (1991). Biological role and regulation of the universally conserved heat shock proteins. J. Biol. Chem. 266:24233-24236.
|
|
Auffray Y, Gansel X, Thammavongs B, Boutibonnes P (1992). Heat-shock induced protein synthesis in Lactococcus lactis subsp. lactis. Curr. Microbiol. 24:281-284.
Crossref
|
|
|
Boutibonnes P, Bison V, Thammavongs B, Hartke A, Panoff J, Benachour A, Auffray Y (1995). Induction of thermo tolerance by chemical agents in Lactococcus lactis subsp. lactis IL1408. Int. J. Food Microb. 25:83-94.
Crossref
|
|
|
Bradford MM (1976). A Rapid and sensitive method for the quantitation of microgram quantities of protein utilizing the principle of protein-dye binding. Anal. Biochem. 72: 248-254.
Crossref
|
|
|
Broadbent JR, Orberg CJ, Wei L (1998). Characterization of the Lactobacillus helveticus groELS operon. Res. Microbiol. 149:247-253.
Crossref
|
|
|
Bron PA, Molenaar D, de Vos WM, Kleerebezem M (2006). DNA micro-array-based identiï¬cation of bile-responsive genes in Lactobacillus plantarum. J. Appl. Microbiol. 100:728-738.
Crossref
|
|
|
Corcoran BM, Stanton C, Fitzgerald G, Ross RP (2008). Life under stress: the probiotic stress response and how it may be manipulated. Curr. Pharm. Des. 14:1382-1399.
Crossref
|
|
|
De Angelis M, Di cagno R, Huet C, Crecchio C, Fox PF, Gobetti M (2004): Heat shock response in Lactobacillus plantarum. Appl. Environ. Microbiol. 70:1336-1346.
Crossref
|
|
|
De Leon P, Marco S, Isiegas C, Marina A, Carrascosa JL, Mellado RP (1997). Streptomyces lividans groES, groEL1 and groEL2 genes. Microbiology 143:3563-3571.
Crossref
|
|
|
Desmond C, Fitzgerald GF, Stanton C, Ross RP (2004). Improved stress tolerance of GroESL-overproducing Lactococcus lactis and probiotic Lactobacillus paracasei NFBC 338. Appl. Environ. Microbiol. 70:5929-5936.
Crossref
|
|
|
Earnshaw RG (1995). Understanding physical inactivation processes: combined preservation opportunities using heat, ultrasound and pressure. Int. J. Food Microbiol. 28:197-219.
Crossref
|
|
|
Flahaut S, Hartke A, Giard JC, Benachour A, Boutibonnes P, Auffray Y (1996). Relationship between stress response toward bile salts, acid and heat treatment in Enterococcus faecalis. FEMS Microbiol. Lett. 138:49-54.
Crossref
|
|
|
Fujita M, Amemura A, Aramaki H (1998). Transcription of the groESL operon in Pseudomonas aeruginosa PAO1. FEMS Microbiol. Lett. 163:237-242.
Crossref
|
|
|
Gouesbet G, Jan G, Boyaval P (2002). Two-dimensional electrophoresis study of Lactobacillus delbrueckii subsp. Bulgaricus thermotolerance. Appl. Environ. Microbiol. 68:1055-1063.
Crossref
|
|
|
Gupta RS (1995). Evolution of the chaperon in families (Hsp60, Hsp10 and Tcp-1) of proteins and origin of eukaryotic cells. Mol. Microbiol. 15:1-11.
Crossref
|
|
|
Guzzo J, Delmas F, Pierre F, Jobin MP, Samyn B, Van Beeumen J, Cavin JF, Divies C (1997). A small heat shock protein from Leuconostoc oenos induced by multiple stresses and during stationary growth phase. Lett. Appl. Microbiol. 24:393-396.
Crossref
|
|
|
Hansen MC, Nielsen AK, Molin S, Hammer K, Kilstrup M, Palmer JrRJ, Udsen C, White DC (2001). Changes in rRNA levels during stress invalidates results from mRNA blotting: fluorescence in situ rRNA hybridization permits renormalization for estimation of cellular mRNA levels. J. Bacteriol. 183:4747-4751.
Crossref
|
|
|
Hecker M, Schumann,W, Volker U (1996). Heat-shock and general response in Bacillus subtilis. Mol. Microbiol. 19:417-428.
Crossref
|
|
|
Hennequin C, Porcheray F, Waligora-Dupriet AJ, Collignon A, Barc M, Bourlioux P, Karjalainen T (2001). GroEL (Hsp60) of Clostridium difficile is involved in cell adherence. Microbiol. 147:87-96.
|
|
|
Joe MK, Parck SM, Lee YS, Hwang DC, Hong CB (2000). High temperature stress resistance of E. Coli induced by a tobacco class I low molecular weight heat-shock proteins. Moll. Cells 10:519-524.
Crossref
|
|
|
Kilstrup M, Jakobsen S, Hammer K, Vogensen FK (1997). Induction of heat shock proteins Dnak, GroEL, and GroES by salt stress in Lactococcus lactis. Appl. Environ. Microbiol. 63:1826-1837.
PMid:9143115 PMCid:PMC168475
|
|
|
Kim WS, Perl L, Park JH, Tandianus JE, Dunn NW (2001). Assessment of stress response of the probiotic Lactobacillus acidophilus. Curr. Microbiol. 43:346-350.
Crossref
|
|
|
Kolter R, Siegele DA, Tormo A (1993).The stationary phase of the bacterial life cycle. Annu. Rev. Microbiol. 47:855-874.
Crossref
|
|
|
Mansouripour S, Esfaudiari Z, Natelghi L (2013). The effect of heat process on the survival and increased viability of probiotic by microcapsulation : a review. Ann. Biolog. Res. 4:83-87.
|
|
|
Narberhaus F (2002). Alpha-crystallin-type heat shock proteins: socializing minichaperones in the context of a multichaperone network. Microbiol. Mol. Biol. Rev. (66): 64-93.
Crossref
|
|
|
Pieterse B, Leer RJ, Schuren FH, Van der Werf MJ (2005). Unravelling the multiple effects of lactic acid stress on Lactobacillus plantarum by transcription proï¬ling. Microbiology 151:3881-3894.
Crossref
|
|
|
Prasad J, McJarrow P, Gopal P (2003). Heat and osmotic stress responses of probiotic Lactobacillus rhamnosus HN001 (DR20) in relation to viability after drying. Appl. Environ. Microbiol. 69:917-925.
Crossref
|
|
|
Rechinger KB, Siegumfeldt H, Svendsen I, Jakobsen M (2000). "Early" protein synthesis of Lactobacillus delbrueckii ssp. bulgaricus in milk revealed by [35S]methionine labeling and two-dimensional gel electrophoresis. Electrophoresis 21:2660-2669.
Crossref
|
|
|
Sabri MY, Zamri-Saad M, Mutalib AR, Israf DA, Muniandy N (2000). Efficacy of an outer membrane protein of Pasteurella haemolytica A2, A7 or A9-enriched vaccine against intratracheal challenge exposure in sheep. Vet. Microbiol. 73: 13-23.
Crossref
|
|
|
Salotra P, Singh DK, Seal KP, Krishna N, Jaffe H, Bhatnagar R (1995). Expression of DnaK and GroEL homologs in Leuconostoc esenteroides in response to heat shock, cold shock or chemical stress. FEMS Microbiol. Lett. 131:57-62.
Crossref
|
|
|
Segal G, Ron EZ (1996). Regulation and organization of the groE and dnaK operons in eubacteria. FEMS Microbiol. Lett. 138:1-10.
Crossref
|
|
|
Serrano LM, Molenaar D, Wels M, Teusink B, De Vos WM, Smid EJ (2007). Thioredoxin reductase is a key factor in the oxidative stress response of Lactobacillus plantarum WCFS1. Microb. Cell Fact. 10:6-29.
Crossref
|
|
|
Spano G, Massa S (2006). Environmental stress response in wine lactic acid bacteria: beyond Bacillus subtilis. Crit. Rev. Microbiol. 32:77-86.
Crossref
|
|
|
Stevens MJA (2008). Transcriptome response of Lactobacillus plantarum to global regulator deï¬ciency, stress and other environmental conditions. The- sis. Wageningen University, Wageningen, The Netherlands.
|
|
|
Svensater G, Sjogreen B, Hamilton IR (2000). Multiple stress responses in Streptococcus mutans and the induction of general and stress-specific proteins. Microbiology 146: 107-117.
|
|
|
Teixera P, Castro H, Mohacsi-Farkas C, Kirby R (1997). Identification of sites of injury in Lactobacillus bulgaricus during heat stress. J. Appl. Microbiol. 83: 219-226.
Crossref
|
|
|
Van de Guchte M, Serror P, Chervaux C, Smokvina T, Ehrlich SD, Maguin E (2002). Stress responses in lactic acid bacteria. Antonie Leeuwenhoek 82:187-216.
Crossref
|
|
|
Veinger L, Diamant S, Buchner J, Goloubinoff P (1998). The small heat shock protein IbpB from Escherichia coli stabilizes stress-denatured proteins for subsequent refolding by a multichaperone network. J. Biol. Chem. 273:11032-11037.
Crossref
|
|
|
Walker DC, Girgis HS, Klaenhammer TR (1999). The groESL chaperone operon of Lactobacillus johnsonii. Appl. Environ. Microbiol. 65: 3033-3041.
|
|
|
Whitaker RD, Batt CA (1991). Characterization of the heat shock response in Lactococcus lactis subsp. lactis. Appl. Environ. Microbiol. 57:1408-1412.
|
|