ABSTRACT
The rapid iterative negative geotaxis (RING) assay is a commonly used behavioral test in Drosophila based on digital imaging of flies and requires minimal equipment and space. Larval mobility can be used to reliably determine the effect of drugs on locomotion in Drosophila larvae. The effects of Valeriana officinalis extract (VoE) and melatonin (ME) were tested in Drosophila melanogaster flies and larvae. In the RING assay, the locomotor activity of flies was reduced to 30.6, 72 and 70.4% when exposed to 10 mg/ml VoE, 8 mM ME, and a combination of 8 mM ME + 10 mg/ml VoE, respectively. The performance index (PI) was reduced to 68.9% (P = 0.0058), 33.3% (P = 0.0001), and 70% (P = 0.0013) when flies were exposed to 8 mM ME, 10 mg/ml VoE, and a combination of 8 mM ME + 10 mg/ml VoE, respectively. The number of body wall contractions of Drosophila larvae (nBWC) was reduced to 47.8, 37.7 and 38.5% when larvae were exposed to 8 mM ME, 10 mg/ml VoE, and a combination of 8 mM ME + 10 mg/ml VoE, respectively. The displacement velocity (V0) of larvae decreased significantly to 67.6, 60.1 and 55.6% when larvae were exposed to 8 mM ME, 10 mg/ml VoE, and a combination of 10 mg/ml VoE + 8 mM ME, respectively (P < 0.05). The effects of the VoE extract were not synergistic with ME as demonstrated by the RING assay, nBWC, and V0. Based on these results, valerian root extracts have demonstrated sedative effects that are better than those of melatonin in fruit flies, but did not show any increase in sedative effect when combined with melatonin.
Key words: Valeriana officinalis roots, Melatonin, Drosophila melanogaster, negative geotaxis, sedative effect, antioxidant.
Sleep disorders and anxiety are common problems that are frequently the cause for consultations with healthcare providers, and have a significant impact on an individual's' quality of life (Miyasaka et al., 2006). Insomnia is the most common sleep disorder, affecting between 6 and 12% of the adult population. Difficulties in initiating and maintaining sleep are also very common in children, affecting between 15 and 25% of this population (Slomp-Junior et al., 2010; Bakhshaei, 2017). Psychotropic medications are predominantly used to treat these pathologies (Schreiber et al., 2008). However, the search for alternative treatments, including the deliberate use of medicinal herbs, has increased substantially in recent years with the identification of natural medicines for the treatment of insomnia and anxiety (Stevinson and Ernst, 2000; Srivastava et al., 2010; Ekor, 2014; Bakhshaei, 2017).
The overuse of psychotropic medications and herbal medicines have two important consequences: the first is that use of psychotropic medications such as benzodiazepines, antidepressants, barbiturates and antipsychotics to treat insomnia and sleep disorders have many side effects such as hangover and drug addiction. Secondly, medicinal herbs are easily accessible, are believed to be devoid of side effects, and are therefore commonly used to resolve sleep disorders (Stevinson and Ernst, 2000; Brattström, 2007; Bakhshaei, 2017). Ang-Lee et al. (2001) have suggested discontinuing herbal medicinal products prior to surgery, and these authors considered valerian in their studies, as it is one of the herbal medicines which has information available for its direct pharmacological effects, as well as pharmacodynamic and pharmacokinetic interactions. Thus, it is necessary for physicians to have a clear understanding of the herbal medicinal products being used by patients and to take a detailed history before combining these herbal products with other pharmaceuticals products (Ang-Lee et al., 2001).
A variety of therapeutic effects, including hypnotic, sedative, anxiolytic, anticonvulsant, and antidepressant functions, have been determined for valerian (Wagner et al., 1980; Oshima et al., 1995; Andreatini et al., 2002; Del Valle-Mojica et al., 2011; Torres-Hernández et al., 2015). Some studies have tested the effects of valerian only, or in combination with other pharmaceuticals or herbal medicines in treating neurological diseases. Torres-Hernández and colleagues (2015) showed that a valerian extract and valerenic acid interacted synergistically with a minimally effective concentration of clonazepam to increase the latency to convulsions and improve survival. These results suggest possible new therapeutic alternatives for epileptic patients (Torres-Hernández et al., 2015). Yoo et al. (2015) showed that the inhibitory effect of the valerian extract on microglia may protect hippocampal neurons from ischemic damage.
The results from these authors support the previous observation that the valerian extract ameliorates the reduction of total thiol content in homogenates and also decreases H2O2 production in the mitochondria of flies exposed to rotenone (Sudati et al., 2009). Thus, the administration of valerian extract significantly increases neuronal survival after ischemia/reperfusion by decreasing reactive microglia and the levels of lipid peroxidation (Yoo et al., 2015). Choi et al. (2017) showed that a valerian and cascade extract mixture (Valeriana officinalis and Humulus lupulus) promotes sleep with a reduction in dark phase activity, compared to control conditions or a single administration of the extracts, in a fruit fly model. This study revealed that a Valerian/Cascade extract mixture improves sleep-related behaviors, including sleeping time, by modulating GABAergic/serotonergic signaling (Choi et al., 2017).
V. officinalis L. is one of the most commonly used medicinal plants worldwide for the treatment of anxiety. Its roots include subterranean organs such as rhizomes and stolons, and are used for the production of herbal medicines (WHO, 1999). It is a native plant from different temperate regions of America, Europe and Asia (Hadley and Petry, 2003). It has been used as a medicinal plant for over 2000 years, since the time of ancient Greece and Rome where it was already being used as a diuretic, analgesic and spasmolytic (Blumenthal et al., 1999). The bioactive component, valerenic acid, extracted from the essential oil is always present in greater quantity in the valerian roots. Valerian is a positive GABAA receptor modulator acting in a dose-dependent manner on a site other than the GABA binding site (Yuan et al., 2004)and increases GABAA neurotransmission (Cavadas et al., 1995). This effect is verified with the demonstration that valerenic acid binds to the β3 subunit of the GABA receptor (Khom et al., 2007).
Consequently, the elimination of this receptor subunit in rats blocks the effects of valerenic acid (Benke et al., 2009). The ethanolic and aqueous extracts of the valerian root inhibit the uptake of GABA (Ortiz et al., 1999). Neamati et al. demonstrated that the hydroalcoholic extract of V. officinalis prevents the depressive behavior observed in rats sensitized with ovalbumin. These results support the traditional beliefs pertaining to the beneficial effect of V. officinalis on the nervous system (Neamati et al., 2014). Drugs such as melatonin have established uses in medicinal clinics for the treatment of some sleep disorders such as insomnia, sleep-wake cycle with different 24 h periods, prolonged sleep latency, sleep fragmentation, REM sleep behavioral disorders, among others (Kurganova and Danilov, 2015). Melatonin is an important regulator of the sleep-wake cycle, and in addition displays antioxidant, antiamyloidogenic, neurotrophic and neuroplastic activity.
It is used as a therapeutic adjunct in the treatment of neurological and degenerative diseases (such as autism, attention deficit hyperactivity disorder, etc.) that result in changes in sleep and circadian biological rhythms. In some of these studies, in addition to sleep enhancements, the antioxidant properties of this hormone were the main reason for testing this pineal hormone (Reiter, 1998; Reiter et al., 1999;Reiter et al., 2000;Reiter et al., 2003). In a recent publication, Vankirk et al. (2017), using a Drosophila model, demonstrated that injection of melatonin increases the regularity of heartbeat significantly, and it can rescue rhythmicity in flies bearing mutations that adversely affect cardiac function. The authors showed compelling evidence that melatonin’s action as an antioxidant is not the mechanism underlying improved cardiac performance and that melatonin’s action on the heart is mediated via a specific G-Protein-coupled receptor encoded by a specific gene, which is a promising candidate for melatonin receptor (VanKirk et al., 2017).
The aim of the present study was to investigate the potential synergistic action of the valerian root extract and melatonin in the RING assay using D. melanogaster. The use of D. melanogaster is widespread in genetic studies, and in recent years has been classically used as a genetic model in different human diseases including neurodegenerative diseases such as Parkinson’s, Huntington’s, spinocerebellar ataxia and Alzheimer's disease (Coulom and Birman, 2004; Choi et al., 2017). Experimental models using invertebrates such as D. melanogaster offer a wide variety of experimental tools (Jeibmann and Paulus, 2009; VanKirk et al., 2017; Choi et al., 2017). For example, they are widely used by researchers because they are relatively easy to maintain in the laboratory, have a short reproductive cycle, provide a large number of individuals per progeny and have metabolic reactions similar to those of mammals, which allows some degree of extrapolation for humans (Graf et al., 1994). In addition to these characteristics, Drosophila presents an excellent database of information on mutations, ecology and behavior (Li et al., 2013).
Plant material and preparation of V. officinalis roots extract
Commercial samples of V. officinalis roots were obtained from compounding pharmacies in the Guarapuava City, State of Paraná, Brazil, accompanied by reports of quality control analysis carried out by suppliers of the raw materials. In preparation of the extract, 40 g of V. officinalis roots were used. The samples were extracted by turbo-extraction using a processor with 1 L of distilled water and kept in an amber glass jar for 24 h. The extract was then filtered and stored in hermetically sealed amber glass bottles and refrigerated until use.
Preparation of V. officinalis Extract for DPPH reaction
V. officinalis extract (VoE) with methanol was obtained for the reaction with the radical DPPH• (2,2-diphenyl-1-picryl-hydrazyl). Samples of 10 g of V. officinalis roots were transferred to a beaker containing 100 ml of solvent (methanol), wrapped with aluminum foil and automatically agitated for 12 h using a shaker. After 12 h, the samples were vacuum filtered. After filtration, an additional 100 ml of solvent was added to the remaining sample and re-filtered. The filtrate was subjected to a rotary evaporator to evaporate the organic solvent. The extracts were stored in capped amber flasks and subsequently cooled.
DPPH Reaction
The antioxidant potential of the methanolic extract of valerian roots was determined by the reduction of the absorbance of the DPPH• (2,2-diphenyl-1-picrylhydrazyl) radical at 517 nm, with a change from violet to yellowish, proportional to the concentration of the reducing substance in the sample, (Brand-Williams et al., 1995). Final volumes of the valerian methanolic extract solution with indicated concentration (0.17 to 2 mg/ml) were adjusted to 500 ml with methanol. Later, this solution was mixed with 2.5 ml of DPPH (6 x 10-5 mol/l) and homogenized at room temperature. After 60 min of reaction (until stable absorption values were obtained), the absorbance was read at 517 nm. A control reference containing 2.5 ml of DPPH + 500 μl of methanol was also prepared. Antiradical activity (AA) was determined as %AA using the following equation: %AA = [(Absorbance of Reference – Absorbance of Sample)/(Absorbance of Reference)] x 100. The IC50 value is the concentration of sample (extract/standard) that provided 50% inhibition of the DPPH radical. A linear graph of concentration versus percentage inhibition was prepared and IC50 values were calculated. The lower the IC50 value, the higher the antioxidant capacity. A standard reference curve was prepared with ascorbic acid at concentrations of 5, 10, 20, 30 and 40 mg/ml.
Culture of D. melanogaster
Canton-S flies were supplied by the Laboratory of Genetics and Evolution, Department of Biological Sciences, Universidade Estadual do Centro-Oeste/UNICENTRO - Paraná State - Brazil. All flies were reared at 25°C and 55% relative humidity under a 12-hour light/dark cycle in bottles with standard food medium (10% sucrose, 2% yeast, 3.3% cornmeal, 1% agar) and distributed at a density of 12 male and female parents per bottle. Flies were allowed to recover for at least 18 hours at 25°C/55% humidity before the negative geotaxis assays were performed. To avoid the probable variability in the relative sensitivity of different strains of D. melanogaster to the valerian roots extract and melatonin, all experiments were performed using the same lineage in both sexes.
RING assay
The flies had an average climbing height of ~4-5 cm in a 3-second time period (the time can be adjusted from 3 seconds to accommodate different strains or activity levels, or to define a given average height for a given strain/treatment). These flies were grown in standard food medium for D. melanogaster containing 1, 2.5, 5 and 10 mg/ml VoE, 8 mM ME or a combination of both 8 mM ME + 10 mg/ml VoE. The choice of V. officinalis aqueous extract concentrations was based on previous observations by Sudati et al. (2013) which showed that V. officinalis aqueous extracts in the range of 5 - 20 mg/ml did not cause overt signals of toxicity in flies. Coulom and Birman (2004) showed that a 5 mM concentration of the antioxidant melatonin was able to prevent both locomotor deficits and neuron degeneration in Drosophila.
Thus, melatonin in similar concentration or larger could be used to prevent oxidative stress (Coulom and Birman, 2004). For the negative control, the flies were developed in culture medium without VoE or ME. Newly emerged adult flies that emerged in the flasks containing food and extract, melatonin or the combination of both were anesthetized in CO2 and transferred to new flasks containing food and extract, melatonin or the combination of both. After 3 days for recovery from CO2 (and accumulation of steady-state drug levels), 20 flies were transferred without anesthetizing to prepared polystyrene vials (Figure 1). The vials with flies were assembled into the RING apparatus and were allowed to acclimate to the environment, undisturbed, for 15 min. During this time, the camera was placed ~1 m in front of the apparatus and a timer was set to 3.0 s. Carefully, the RING apparatus was sharply tapped on the surface of the bench three times, ensuring that the tap was hard enough to knock down all the flies to the bottom of the vials. Simultaneous with the completion of the third tap, the timer was started and after 3 s a picture was taken.


The timer was reset for 1 min and started. During this time, the camera was reset and another channel of the timer was set for three seconds. After 1 min, these steps were repeated for a total of 5 trials. The images were uploaded onto a computer and an image viewer was used to open and score the average height climbed for each vial (Nichols et al., 2012). The scores are the mean of the numbers of flies at the top (ntop) and at the bottom of vials (nbot), expressed as percentages of the total number of flies (ntot). Results are presented as the mean ± SEM of the scores obtained in 3 independent experiments. For each experiment, a performance index (PI) was calculated, defined as PI = 1/2[(ntot + ntop - nbot)/ntot] (Feany and Bender, 2000;Friggi-Grelin et al., 2003;Coulom and Birman, 2004). For the RING assay, it is imperative that the flies not be anesthetized before testing, and that fresh vials are used after a given set of trials before a new batch of flies is tested.
Determination of larval mobility
The determination of D. melanogaster larvae mobility was performed in accordance with the protocol by Nichols et al. (2012). One bottle of D. melanogaster flies (10 to 15 males + 10 to 15 females) was prepared. The flies laid eggs for 24 hours and then were removed from the bottle. The bottle was incubated for 3-4 days, or until the third instar larvae were visible. Then 100 ml of 20% sucrose was added to the flask with larvae kept at rest for 20 min. When the larvae were bloated in the middle with sucrose, they were collected using a 25-ml serological pipette with the tip cut off and placed into a mesh basket. The larvae were then rinsed three times with deionized H2O. The larvae were transported with a small brush into 5 ml beakers containing a 5% sucrose solution plus the aqueous extract of V. officinalis at concentrations of 1, 2.5, 5 and 10 mg/ml, melatonin at a concentration of 8 mM or a combination of 8 mM melatonin + 10 mg/ml V. officinalis. The larvae were exposed to the extract and melatonin for 15 min.
Subsequently, the larvae were rinsed again with deionized H2O 3 times. The larvae were then transported to a Petri dish (15 cm in diameter) containing 2% agarose gel (previously spilled and allowed to harden) and placed over a graph paper with a 0.1 cm2 grid. Larval mobility (measured by distance traveled and body wall contractions of the larvae) was then performed. The number of crossed lines by larvae was counted in 1 min, and the results expressed in centimeters traveled per min. A microscopic dissecting magnifying glass was used to quantify the peristaltic movement or body wall contractions of the larvae (1 complete anteroposterior movement = 1 contraction) for 1 min. In this type of experiment, D. melanogaster larvae crawl 3 cm/min on average and carry out approximately 40 - 50 body wall contractions in 1 min (Nichols et al., 2012).
Statistical analysis
Numerical results were expressed as an arithmetic mean ± standard error of the mean. The differences between the groups were determined by Analysis of Variance (ANOVA) followed, when detected, by Tukey's multiple comparisons test. Statistically significant differences for the number of flies at the bottom or top of the RING assay were determined using two-way ANOVA. The analysis was done using GraphPad Prism® version 5.01 for Windows®, GraphPad Software®, San Diego, California, USA. The results were considered statistically significant when P < 0.05.
Antioxidant activity
The DPPH assay was used to determine the free radical scavenging activity of the valerian methanolic extract. DPPH is a stable free radical and accepts an electron or hydrogen radical to become a stable diamagnetic molecule (Oliveira, 2015). The free radical scavenging activity of DPPH was determined by the decrease in absorbance at 517 nm, which is induced by antioxidants such as ascorbic acid. According to the results obtained in Table 1, the methanolic extract of valerian showed an IC50 = 887.29 ± 10.55 μg/ml (y = 38.91x + 15.474; R2 = 0.9906) as compared to the ascorbic acid IC50 = 16.62 ± 0.16 μg/ml (y = 2031.1x + 16.247; R2 = 0.9912). A lower IC50 value indicates higher antioxidant potential. It was also found that the free radical-scavenging activities of the extract increased with increasing concentration (data not shown).


RING assay
In order to verify the effects of the valerian extract (VoE), melatonin (ME) and the combination of the valerian extract + melatonin (VoE + ME), the RING assay was conducted using D. melanogaster as an experimental model. Figure 2A shows the effects of VoE on the RING assay of D. melanogaster. In all tested concentrations of the extract (1 to 10 mg/ml), a reduction in fly mobility was observed. At the concentration of 10 mg/ml, the mean number of flies at the top of the vial was 6.67 ± 0.33 (35%) and at the bottom of the vial was 13.33 ± 0.33 (65%) which was statistically different (P < 0.0001) when compared to control, that showed a mean number of 18.0 ± 0.58 (90%) flies at the top and 2.0 ± 0.58 (10%) flies at the bottom of the vial. These results showed that the valerian extract significantly reduced the locomotor activity of flies that developed in medium containing valerian.
The locomotor performance of D. melanogaster showed that at a concentration of 8 mM of ME, 10 mg/ml VoE and a combination of 8 mM ME + 10 mg/ml VoE extract (Figure 2B), the performance of flies was significantly reduced in relation to the control. The data showed that a concentration of 10 mg/ml VoE was more effective in decreasing the locomotor performance of flies than a concentration of 8 mM ME (Figure 2B). In the presence of 8 mM of ME, the number of D. melanogaster flies that reached the top of the tube in the RING assay was reduced to 13 ± 0.97 (72%), whereas with a concentration of 10 mg/ml VoE, the number was reduced to 5.5 ± 0.5 (30.6%). The combined concentration of 8 mM ME + 10 mg/ml VoE reduced the performance to 12.67 ± 0.33 (70.4%) flies at the top of the tube during the test. The sedative effects of 8 mM ME was not increased when the RING assay was conducted with a combined concentration of 8 mM of ME + 10 mg/mL of VoE.
Performance Index (PI) was calculated to confirm the effect observed when flies were exposed to melatonin, valerian root extract, and the combination of both. Figure 3A shows the PI calculated for both untreated and valerian root extract-treated flies. In all tested concentrations of valerian root extract, the PI was reduced in relation to the control. At different dose of 1, 2.5, 5 and 10 mg/ml of VoE, there was a reduction in PI to 0.7 ± 0.03 (77.8%), 0.52 ± 0.04 (57.8%), 0.50 ± 0.029 (55.5%) and 0.33 ± 0.017 (36.7%), respectively. The control PI value was 0.9 ± 0.029. The locomotor activity of the flies was decreased by the V. officinalis extract and melatonin. Figure 3B shows that a concentration of 8 mM melatonin decreased the Performance Index (PI) to 0.62 ± 0.044 (68.9%) (P = 0.0058). When flies were exposed to a combination of 8 mM ME + 10 mg/ml VoE, the PI was decreased to 0.63 ± 0.0167 (70%) (P = 0.0013). However, at a concentration of 10 mg/ml VoE, the inhibition of PI was more pronounced and PI was reduced to 0.3 ± 0.029 (33.3%) (P = 0.0001) compared to the control.
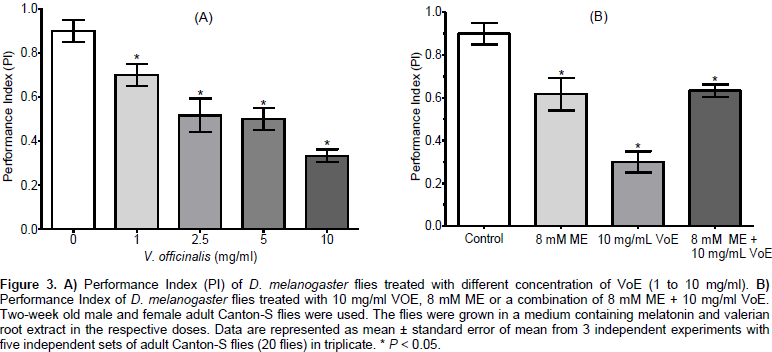
Determination of larval mobility
Figure 4A shows the number of body wall contractions (nBWC) of the 3rd instar larvae of D. melanogaster in the absence (control) and presence of VoE. In this experiment, the 3rd instar larvae were exposed to VoE or ME for 15 min before the mobility test. The number of body wall contractions nBWC of the larvae was 59.67 ± 1.67 in the control group. The exposure of the larvae to the different concentration of 2.5, 5 and 10 mg/ml VoE showed a reduction of nBWC to 46 ± 2.51 (77.1%), 30 ± 3.46 (50.3%) and 22.5 ± 1.52 (37.7%), respectively, when compared to the control. Figure 4B shows the nBWC of D. melanogaster larvae in the absence (control) and in the presence of 8 mM ME or 10 mg/ml VoE or a combination of 8 mM ME + 10 mg/ml VoE. The data showed that nBWC was reduced to 28.5 ± 1.5 (47.8%) when larvae were exposed to 8 mM ME, to 22.5 ± 1.5 (37.7%) when larvae were exposed to 10 mg/ml VoE, and to 23 ± 2.38 (38.5%) when larvae were exposed to both 8 mM ME + 10 mg/ml VoE.

No significant difference was observed between larvae exposed to 8 mM ME and 10 mg/ml VoE (P = 0.2203), between larvae exposed to 8 mM ME and a combination of 8 mM ME + 10 mg/ml VoE (P = 0.376), or between larvae exposed to 10 mg/ml VoE and 8 mM ME + 10 mg/ml VoE (P = 0.898). nBWC for larvae exposed to 10 mg/ml of VoE was not statistically different to that observed when larvae were exposed to 8 mM ME, or to a combination of 8 mM of ME + 10 mg/ml of VoE (P > 0.05). Figure 5A shows that the control 3rd instar larvae showed a mean value of 3.33 ± 0.33 cm/min (100%) for displacement velocity (V0), whereas 3rd instar larvae treated with 2.5, 5 and 10 mg/ml VoE reduced the mean value of V0 to 2.0 ± 0.20 cm/min (60.1%), 1.75 ± 0.32 cm/min (52.6%) and 2.0 ± 0.20 cm/min (60.1%), respectively. In Figure 5B, the mean values of V0 were 2.25 ± 0.14 cm/min (67.6%), 2.0 ± 0.20 cm/min (60.1%) and 1.85 ± 0.36 cm/min (55.6%) for the 3rd instar larvae treated with 8 mM ME, 10 mg/ml VoE or a combination of both 8 mM ME + 10 mg/ml VoE respectively. All treatments were statistically different (P < 0.05) from the control (Figure 5B). The data show that effects of ME x VoE, VoE x ME+VoE, and ME x ME+VoE were not statistically significant (P = 0.3559, P = 0.7328, and P = 0.3488, respectively). The data supports the notion that valerian improves sleep and induces sedative effects when compared to control, but does not present synergistic effects after association with melatonin.
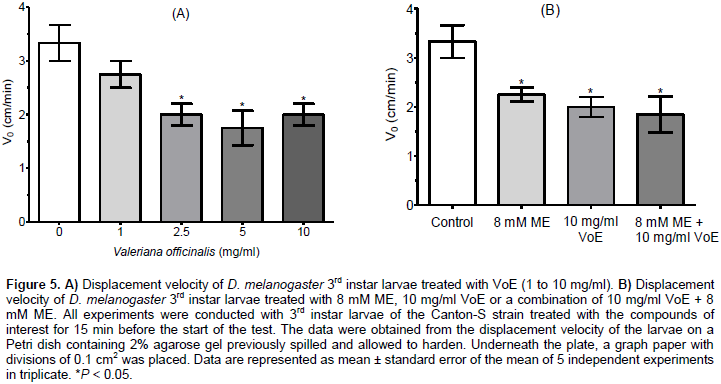
The present work was initiated because recent studies with rigorous designs reported no significant benefits of valerian treatment on sleep outcomes (Coxeter et al., 2003; Brattström, 2007; Taibi et al., 2007). Although pharmacological treatments have been suggested for anxiety and sleep disorders, the demand for natural product-targeting studies has increased owing to side effects caused by the interaction between plant extracts and drugs. Therefore, this work presented the potential of combining valerian and melatonin as a sedative agent in a fruit fly model of climbing and locomotor activity. Sudati et al. (2013) demonstrated that the aqueous extract from valerian roots was effective in reducing motor impairments and some oxidative stress parameters mediated by rotenone (an inhibitor of complex I in the mitochondrial respiratory chain).
It is widely accepted that the effects mediated by rotenone encompass oxidative stress linked with mitochondrial dysfunction and reactive oxygen species (ROS) overproduction (Cicchetti et al., 2009). However, our data showed that the methanolic extract of valerian roots showed low antioxidant activity (IC50 887.29 ± 10.55 mg/ml) for the radical DPPH as compared with the antioxidant activity of ascorbic acid (IC50 16.62 ± 0.16 mg/ml). We used the RING assay to evaluate the effects of the valerian roots extract, melatonin and a combination of both valerian root extract and melatonin in Drosophila. In the RING assay, it was observed that valerian decreased the mobility of flies when compared to the control. We did not observe a synergistic effect when valerian extract was combined with melatonin. Melatonin or valerian extract decreased the mobility of flies, and valerian extract (10 mg/ml) showed a higher sedative effect compared to melatonin (Figure 2).
When 8 mM of melatonin + 10 mg/ml of valerian root extract were combined in the food of flies for the RING assay, the effect observed was similar to what was observed when only 8 mM of melatonin was added to the food of flies (Flies in the top of vial P = 0.7244). The sedative effect of valerian and melatonin on the locomotor activity of D. melanogaster flies was identified (Figures 2 and 3). The effect of the valerian extract (10 mg/ml) was higher than the effect of melatonin (8 mM) on locomotor activity of flies, when compared to the control flies (Control x ME(top) P = 0.0178; Control x VoE(top) P = 0.0006). The combination of valerian extract (10 mg/ml) and melatonin (8 mM) did not have a synergistic effect on the locomotor activity of flies compared to ME (8 mM) [ME x ME+VoE(top) P = 0.7244]. However, VoE alone was more effective in promoting the sedative effect compared to ME alone [VoE x ME(top) P = 0.0215]. This was also the case when VoE alone was compared to ME + VoE [VoE x ME+VoE(top) P = 0.0011]. Thus, this result provides evidence that a valerian and melatonin combination does not exert a pronounced sedative effect in Drosophila. Additionally, valerian promotes a more pronounced sedative effect than melatonin in the fly fruit model.
The number of body wall contractions (nBWC) (Figure 4) was reduced to approximately one third (37.7%) of that observed in control flies when exposed to 10 mg/ml of valerian roots extract (Control x VoE P = 0.0006) and to 47.8% when exposed to 8 mM melatonin (Control x ME P = 0.0001). A combination of valerian roots extract (10 mg/ml) and melatonin (8 mM) did not provid a synergistic effect in the number of body wall contractions (nBWC reduced to 38.5%) but it was statistically different in the control flies (Control × VoE+ME, P < 0.0001). The effect of melatonin (8 mM) compared to the effect of valerian roots extract (10 mg/ml) was not significant (ME × VoE P = 0.2203). Similarly, the effect of melatonin compared to the effect of a combination of melatonin + valerian (ME x ME+VoE P = 0.3766), as well as the effect of valerian compared to the effect of the melatonin + valerian combination (VoE x ME+VoE P = 0.8987) on the nBWC were also not significant. The velocity of displacement (V0 cm/min) was reduced significantly when larvae were exposed to 10 mg/ml of valerian roots extract (Figure 5) (Control x VoE, P = 0.0153), 8 mM of melatonin (Control x ME, P = 0.0212), and a combination of 8 mM ME + 10 mg/ml VoE (Control x ME+VoE, P = 0.0347), compared to control larvae.
V0 of melatonin-treated larvae was not significantly different from Vâ‚’ of larvae treated with 10 mg/ml of valerian root extract (ME x VoE, P = 0.3559). Similarly, V0 of melatonin-treated larvae was not significantly different from Vâ‚’ larvae treated with a combination of valerian and melatonin (ME x ME+VoE, P = 0.3488). The effects of 10 mg/ml of valerian roots extract were not significantly different from the effects of a combination of valerian and melatonin (VoE x ME+VoE, P = 0.7328). These tests (RING, nBWC and V0) were conducted at a temperature of ~22 °C as recommended by Nichols et al. (2012). The larval crawling assay, as a test of locomotion, is more applicable if expression or abolition of a gene causes lethality in pupal or adult stages. However, the tests (nBWC and V0) were performed in our study because it was possible to observe subtle differences in the behavior of treated and untreated Drosophila larvae and flies with valerian extract at concentrations of 1 and 2.5 mg/ml compared to the control group of Drosophila larvae or flies.
Valerian roots are generally used as a dietary supplement and have been clinically proven to support better sleep and mood with long-term use. Several studies have shown that it promotes a feeling of calmness, reduces anxiety, and provides relief from muscle spasms, migraine headaches, and insomnia. However, effects such as those mentioned above may occur after prolonged use of valerian. The valerian roots have been used for over 2,000 years. Therefore, it is erroneously recognized as a safe, natural supplement with few side effects. On the other hand, a valerian root overdose is uncommon. However, herbal supplements should always be taken under the supervision of a knowledgeable and licensed heath care provider. Although not the case in this study, adverse effects can occur in certain situations when it is inappropriately combined with other drugs or taken in excess quantities. In summary, the results of the present study show that the V. officinalis extract is efficient in promoting sedative effects in D. melanogaster, however, a combination of melatonin and valerian roots extract does not appear to promote a synergistic effect in the melatonin action or to intensify the sedative effect. Therefore, additional studies on the pharmacokinetics and pharmacodynamics of the combination of valerian and melatonin should be performed.
The authors have not declared any conflict of interests.
The authors acknowledge Fundação Araucária for the fellowship provided to one of the authors. The authors gratefully acknowledge Professors Rogério P. Mateus and Luciana P. B. Machado from Lab of Genetic and Evolution (UNICENTRO) that generously provided the line of D. melanogaster used in this research and the support of Gabriela R. Salomón from UNICENTRO for the teaching of fruit fly handling skills.
REFERENCES
Andreatini R, Sartori VA, Seabra MLV, Leite JR (2002). Effect of valepotriates (valerian extract) in generalized anxiety disorder: a randomized placebo-controlled pilot study. Phytother. Res. 16(7):650-654.
Crossref
|
|
Ang-Lee MK, Moss J, Yuan CS (2001). Herbal medicines and perioperative care. JAMA 286(2):208-216.
Crossref
|
|
|
Bakhshaei S (2017). Phyto-pharmacological effect of nine medicinal plants as a tradicional treatment on depression. J. Appl. Pharm. 9(3):1-5.
|
|
|
Benke D, Barberis A, Kopp S, Altmann KH, Schubiger M, Vogt KE, Möhler H (2009). GABAA receptors as in vivo substrate for the anxiolytic action of valerenic acid, a major constituent of valerian root extracts. Neuropharmacology 56(1):174-181.
Crossref
|
|
|
Blumenthal M, Busse W, Golbderg A, Gruenwald J (1999). The Complete German Commission E Monographs: Therapeutic Guide to Herbal Medicines. Integrative Medicine Communication. Available at:
View
|
|
|
Brand-Williams W, Cuvelier ME, Berset C (1995). Use of a free radical method to evaluate antioxidant activity. Food Sci. Technol. 28(1):25-30.
Crossref
|
|
|
Brattström A (2007). Scientific evidence for a fixed extract combination (Ze 91019) from Valerian and hops traditionally used as a sleep-inducing aid. Wiener Medizinische Wochenschrift 157(13-14):367-370.
Crossref
|
|
|
Cavadas C, Araujo I, Cotrim MD, Amaral T, Cunha AP, Macedo T, Ribeiro CF (1995). In vitro study on the interaction of Valeriana officinalis L. extracts and their amino acids on GABAA receptor in rat brain. Arzneimittelforschung 45(7):753-755.
|
|
|
Choi H-S, Ko BS, Kim HD, Hong K-B, Suh HJ (2017). Effect of Valerian/Hop mixture on sleep-related behaviors in Drosophila melanogaster. Biol. Pharm. Bull. 40(7):1101-1110.
Crossref
|
|
|
Cicchetti F, Drouin-Ouellet J, Gross RE (2009). Environmental toxins and Parkinson's disease: what have we learned from pesticide-induced animal models? Trends Pharmacol. Sci. 30(9):475-483.
Crossref
|
|
|
Coulom H, Birman S (2004). Chronic exposure to rotenone models sporadic Parkinson's disease in Drosophila melanogaster. J. Neurosci. 24(48):10993-10998.
Crossref
|
|
|
Coxeter PD, Schluter PJ, Eastwood HL, Nikles CJ, Glasziou PP (2003). Valerian does not appear to reduce symptoms for patients with chronic insomnia in general practice using a series of randomized n-of-1 trials. Comp. Ther. Med. 11(4):215-222.
Crossref
|
|
|
Kurganova YM, Danilov AB (2015). Melatonin in chronic pain syndromes. Zhurnal Nevrologii Psikhiatrii Imeni Korsakova 115(10 Pt 2):47-54.
|
|
|
Del Valle-Mojica LM, Cordero-Hernández JM, González-Medina G, Ramos-Vélez I, Berríos-Cartagena N, Torres-Hernández BA, Ortíz JG (2011). Aqueous and ethanolic Valeriana officinalis extracts change the binding of ligands to glutamate receptors. Evid. Based Complement. Altern. Med. 2011:891819.
Crossref
|
|
|
Ekor M (2014). The growing use of herbal medicines: Issues relating to adverse reactions and challenges in monitoring safety. Front. Neurol. 4:1-10.
Crossref
|
|
|
Feany MB, Bender WW (2000). A Drosophila model of Parkinson's disease. Nature 404(6776):394-398.
Crossref
|
|
|
Friggi-Grelin F, Coulom M, Meller M, Gomez D, Hirsh J, Birman S (2003). Targeted gene expression in Drosophila dopaminergic cells using regulatory sequences from tyrosine hydroxylase. J. Neurobiol. 64(4):618-627.
Crossref
|
|
|
Graf U, Moraga AA, Castro R, Carrillo, ED (1994). Genotoxicity testing of different types of beverages in the Drosophila wing somatic mutation and recombination test. Food Chem. Toxicol. 32(5):423-430.
Crossref
|
|
|
Hadley S, Petry JJ (2003). Valerian. Am. Fam. Phys. 67(8):1755-1758.
|
|
|
Jeibmann A, Paulus W (2009). Drosophila melanogaster as a model organism of brain diseases. Int. J. Mol. Sci. 10(2):407-440.
Crossref
|
|
|
Khom S, Baburin I, Timin E, Hohaus A, Trauner G, Kopp B, Hering S (2007). Valerenic acid potentiates and inhibits GABAA receptors: Molecular mechanism and subunit specificity. Neuropharmacology 53(1):178–187.
Crossref
|
|
|
Li Y, Zhou Z, Zhang X, Tong H, Li P, Zhang ZC, Jia Z, Xie W, Han J (2013). Drosophila neuroligin 4 regulates sleep through modulating GABA transmission. J. Neurosci. 33(39):15545-15554.
Crossref
|
|
|
Miyasaka LS, Atallah AN, Soares BGO (2006). Valerian for anxiety disorders. Cochrane Database Syst. Rev. 4:CD004515.
Crossref
|
|
|
Neamati A, Chaman F, Hosseini M, Boskabady MH (2014). The effects of Valeriana officinalis L. hydro-alcoholic extract on depression like behavior in ovalbumin sensitized rats. J. Pharm. Bioallied Sci. 6(2):97-103.
Crossref
|
|
|
Nichols CD, Becnel J, Pandey UB (2012). Methods to assay drosophila behavior. J. Visual. Exp. 61:e3795.
|
|
|
Oliveira GLS (2015). Determinação da capacidade antioxidante de produtos naturais in vitro pelo método do dpph: Estudo de revisão. Rev. Bras. Plantas Med. 17(1):36-44.
Crossref
|
|
|
Ortiz JG, Nieves-Natal J, Chavez P (1999). Effects of Valeriana officinalis extracts on [3H] flunitrazepam binding, synaptosomal [3H] GABA uptake, and hippocampal [3H] GABA release. Neurochem. Res. 24(11):1373-1378.
Crossref
|
|
|
Oshima Y, Matsuoka S, Ohizumi Y (1995). Antidepressant principles of Valeriana fauriei roots. Chem. Pharm. Bull. 43(1):169-170.
Crossref
|
|
|
Reiter RJ (1998). Oxidative damage in the central nervous system: Protection by melatonin. Prog. Neurobiol. 56(3):359-384.
Crossref
|
|
|
Reiter RJ, Tan DX, Cabrera J, D'Arpa D, Sainz RM, Mayo JC, Ramos S (1999). The oxidant/antioxidant network: role of melatonin. Biol. Signals Recep. 8(1-2):56-63.
Crossref
|
|
|
Reiter RJ, Tan DX, Mayo JC, Sainz RM, Leon J, Czarnocki Z (2003). Melatonin as an antioxidant: Biochemical mechanisms and pathophysiological implications in humans. Acta Biochim. Pol. 50(4):1129-1146.
|
|
|
Reiter RJ, Tan DX, Osuna C, Gitto E (2000). Actions of melatonin in the reduction of oxidative stress. A review. J. Biomed. Sci. 7(6):444-458.
Crossref
|
|
|
Schreiber S, Peles E, Adelson M (2008). Association between improvement in depression, reduced benzodiazepine (BDZ) abuse, and increased psychotropic medication use in methadone maintenance treatment (MMT) patients. Drug Alcohol Dep. 92(1-3):79-85.
Crossref
|
|
|
Slomp-Junior H, Seniski G, Cunha C, Audi EA, Andreatini R (2010). The combination of Passiflora alata and Valeriana officinalis on memory tasks in mice: Comparison with diazepam. Braz. Arch. Biol. Technol. 53(6):1343-1350.
Crossref
|
|
|
Srivastava JK, Shankar E, Gupta S (2010). Chamomile: A herbal medicine of the past with a bright future (review). Mol. Med. Reports 3(6):895-901.
|
|
|
Stevinson C, Ernst E (2000). Valerian for insomnia: a systematic review of randomized clinical trials. Sleep Med. 1(2):91-99.
Crossref
|
|
|
Sudati JH, Fachinetto R, Pereira RP, Boligon AA, Athayde ML, Soares FA, Barbosa NBV, Rocha JBT (2009). In vitro antioxidant activity of Valeriana officinalis against different neurotoxic agents. Neurochem. Res. 34(8):1372-1379.
Crossref
|
|
|
Sudati JH, Vieira, FA, Pavin, SS, Dias, GR, Seeger RL, Golombieski, R, Athayde ML, Soares FA, Rocha JB, Barbosa NV (2013). Valeriana officinalis attenuates the rotenone-induced toxicity in Drosophila melanogaster. Neurotoxicol. 37:118-126.
Crossref
|
|
|
Taibi DM, Landis CA, Petry H, Vitiello MV (2007). A systematic review of valerian as a sleep aid: Safe but not effective. Sleep Med. Rev. 11(3):209-230.
Crossref
|
|
|
Torres-Hernández BA, Del Valle-Mojica LM, Ortíz JG (2015). Valerenic acid and Valeriana officinalis extracts delay onset of Pentylenetetrazole (PTZ)-Induced seizures in adult Danio rerio (Zebrafish). BMC Complement. Altern. Med. 15:1-10.
Crossref
|
|
|
VanKirk T, Powers E, Dowse HB (2017). Melatonin increases the regularity of cardiac rhythmicity in the Drosophila heart in both wild-type and strains bearing pathogenic mutations. J. Comp. Physiol. B 187(1):63-78.
Crossref
|
|
|
Wagner H, Jurcic K, Schaette R (1980). Comparative studies on the sedative action of valeriana extracts, valepotriates and their degradation products. Planta Med. 38:358-365.
Crossref
|
|
|
World Health Organization (WHO) (1999). WHO monographs on selected medicinal plants.
|
|
|
Yoo DY, Jung HY, Nam SM, Kim JW, Choi JH, Kwak YG, Yoo M, Lee S, Yoon YS, Hwang IK (2015). Valeriana officinalis extracts ameliorate neuronal damage by suppressing lipid peroxidation in the gerbil hippocampus following transient cerebral ischemia. J. Med. Food 18(6):642-647.
Crossref
|
|
|
Yuan CS, Mehendale S, Xiao Y, Aung HH, Xie JT, Ang-Lee MK (2004). The gamma-aminobutyric acidergic effects of valerian and valerenic acid on rat brainstem neuronal activity. Anesth. Analg. 98(2):353-358.
Crossref
|
|