ABSTRACT
Korean Hackberry (Celtis koraiensis Nakai), an ornamental, deciduous tree, lacks species resources, and low germination percentage also prevent the exploitation. Thus it becomes necessary to investage the mechanism of seed dormancy. This research explored the effect of plant hormone and seed coat (endocarp and testa) on seed germination. Endogenous hormone concentration, protein content, total soluble sugars, total starch and amino acids during germination were studied and the germination recorded with different seed coat treatments. Four seed lots were evaluated: (i) fresh; (ii) imbibed; (iii) germinated; (iv) non-germinated seeds. In seeds treated with gibberellic acid (+GA3), the rate of germination was higher than in untreated (control) seeds. Exogenous GA3 also promoted the generation of endogenous GA3, increasing the total bioactive gibberellins (GA) in the embryo during germination relative to the control. Endogenous abscisic acid (ABA) concentration decreased during germination, however, the GA/ABA ratio was consistently higher in germinated than non-germinated seeds. The GA3 treatment had a significant effect on the GA/ABA ratio. One-trans-zeatin riboside (ZR) and indole-3-acetic acid (IAA) content increased in germinated seeds. From dormancy to germination, the seeds of Korean Hackberry underwent changes in protein content, decreasing in germinated seeds, while concentrations of total soluble sugars and amino acids increased. In addition, germination was higher in seeds with the covering layers removed. The results suggest that germination of Korean Hackberry seeds is controlled by plant hormones and is also affected by the seed coat.
Key words: Abscisic acid, gibberellic acid, germination, indole-3-acetic acid, one-trans-zeatin riboside, storage substances.
Korean Hackberry (Celtis koraiensis), a deciduous tree, is naturally widespread in northern China and in Korea. The beautiful foliage makes it very desirable as an ornamental. In addition, Korean Hackberry is potentially economically important for use in the production of lubricants and soap; this wild species has great development value and possible applications. It is also potentially important in research, because it is highly drought resistant. However, low and erratic seed germination limits the availability of seedlings and seriously affects the cultivation of this tree. Seed dormancy is vital for plant survival and ensures that seeds germinate only when environmental conditions are suitable. It is an adaptive trait in seed-plant species to resist bad environments, enabling plants to survive under stressful conditions in nature (Finkelstein et al., 2008), but can be a barrier for plant exploitation. In order to understand how to break seed dormancy, we need to explore seed germination mechanism. Germination is an extremely complex process that is regulated by signaling molecules, such as hormones, enzymes and antioxidants (El-Maarouf-Bouteau and Bailly, 2008). A considerable amount of research has demonstrated that the transition between dormancy and germination is mediated by plant hormones (Koornneef and Bentsink, 2002; Kucera et al., 2005), especially dependant on the balance of hormones such as abscisic acid (ABA) and gibberellins (GA) (Koornneef and Bentsink, 2002).
Germination is not only a consequence of complex hormone interactions, but also involves changes in cell structure and storage substances. Einali and Sadeghipour (2007) have demonstrated that protein in the seeds of Juglans regia is degraded by activating hydrolases. In the seeds of Corylus avellana, the total starch content has been shown to increase (Li and Ross, 1990) probably as a result of gluconeogenesis is by products of reserve lipid hydrolysis.
Although biochemical and physiological mechanisms during germination have been studied in seeds of many species, the knowledge is deficient in certain genera of trees, such as Korean Hackberry. Hence, in order to understand the mechanisms of Korean Hackberry seed dormancy and germination, we studied the dynamic change of plant hormones and storage substances at different stages during germination in seeds with or without GA3 treatment.
Plant material
Freshly matured fruits were collected from Taishan, at an elevation 751 m, in Shandong province, China (36.23924N° 117.10623°E) during late November 2014. Seeds were removed from the pericarp by hand, and only intact seeds were used for experimental analyses. The word ‘seed’ is used to refer to the propagule with the epicarp removed (botanical seed plus its covering layers: lemma, palea, and pericarp).
Seed covering layers
To investigate the germination pattern affected by the seed covering tissues, mature seeds were dissected as follows: (1) endocarp removed - N, E; (2) endocarp and testa removed-N, E, T; (3) Intact seeds-Control. Seeds which had the endocarp removed were surface-sterilised with 1% mercuric chloride for 5 min, and 75% alcohol for 10 min, the intact seeds were sterilised with 1% mercuric chloride for 10 min, and 75% alcohol for 10 min. All the seeds were inoculated into MS culture medium (8 g.L-1sucrose, 30 g.L-1 agar) and incubated at a constant temperature of 25°C. Seeds were either left in the dark or in an alternating regime of 16 h of white light and 8 h of darkness. Each treatment included three replicates of 30 seeds each.
Germination
The seeds were rinsed for 30 min with distilled water, surface-sterilised with 3% potassium permanganate (KMnO4) for 10 min, and mixed with sand (also sterilised with 0.5% KMnO4 at 60% moisture content in polyethylene mesh bags. The seeds were stored for 5 months at 4°C. All seeds were observed and the germination rate recorded every month. The appearance of the radicle was the criterion adopted for the onset of germination (Ribeiro et al., 2011). The percentage was calculated as the number of germinated seeds/viable seeds ×100, as described by Conversa et al., (2010).
Analyses of plant hormones and storage products in fresh, imbibed, germinated and non-germinated seeds
The aim was to determine the dynamics of endogenous hormones and storage substances during germination in seeds with and without GA3 treatment. Four physiological phases were classified for biochemical analyses: fresh seeds, F (stored at room temperature without imbibition); imbibed seeds, I (stratification about 2 months); non-germinated seeds, NG (undamaged intact seeds after stratification about 5 months without appearance of the radicle); germinated seeds, G (the appearance of the radicle as criterion). To determine the influence of GA3 on germination, the control seeds, without GA3 treatment, were soaked in distilled water for 48 h at room temperature. The seeds treated with GA3 were submerged in 400 mg/L GA3 for 48 h. The seeds were first removed, by hand, from pericarp. Three replicates of 200 seeds were used for each treatment, with every sample frozen in liquid nitrogen and stored at -80°C prior to use.
(i) Seed hormones
The levels of ABA, GA, ZR and IAA were determined using gas chromatographye-mass spectrometry-selected ion monitoring (GC-MS-SIM) (Chen, 2015).
(ii) Storage substances
Total soluble sugars, starch, protein and amino acids were extracted from mature seeds, randomly selected and quantified using ultraviolet spectrophotometry. Total soluble sugars and starch were measured at a wavelength of 620 nm, protein at 595 nm, and the amino acid content at a wavelength of 340 nm. Three replicates were used for each sample. All data analyses were carried out using the procedure of SAS.
Gibberellin effects on Korean Hackberry seed germination
The effect of GA3 on germination was clear, resulting in a two-fold increase in the percentage germination (Figure 1). The difference between treatments was discernible after 50 days incubation. Germination of GA3-treated seeds was significantly higher than the control (F=45.00 P<0.01) after 50 days incubation. Through 5 months cold stratification, the GA3-treated seeds germinated to 18.6%, significantly higher than the control (F=39.20 P<0.01). From days 50 to 80, the increase in the germination percentage after GA3 application was evident. From days80 to 150, the effect of GA3 was not significant (F=10.47; P>0.05). The change in variation of the control was similar to that of the GA3 treatment.
Seed covering layers
In order to investigate whether a possible interaction exists between physiological embryo dormancy and seed coat (endocarp and testa) dormancy, the Korean Hackberry seeds were physically altered and germinated in MS culture medium. The results showed that there is indeed a difference in the seed germination depending on the treatment of the seed coat. There were no significant differences in any treatments after 5 days cultivation. Seeds without endocarp (N, E and N, E, T) had significantly higher germination rates than the control after 15 days incubation, but there was no difference between N, E and N, E, T seeds. Total germination increased by 70% points in N, E seeds and by 75%
points in N, E, T seeds (Table 1).
Changes in endogenous hormone
Through 5 months cold stratification, there were major changes in the hormone levels in the seed embryo. Total ABA content decreased from the F to the G phase in both treatments. Except for the imbibition phases, there were significant differences between control and GA3 treatments (Figure 2), although the total GA concen-trations increased gradually in both. The addition of GA3 significantly affected the endogenous GA concentration, the level increasing from 3.2ng. g-1 FW to 9.1ng. g-1 FW (P<0.01; Figure 2). These results indicate that the GA/ABA ratio in the G phase is significantly higher than in any other phase. There were also significant differences in the GA/ABA ratio between the control and the GA3 treated seeds (P<0.01; Figure 2).
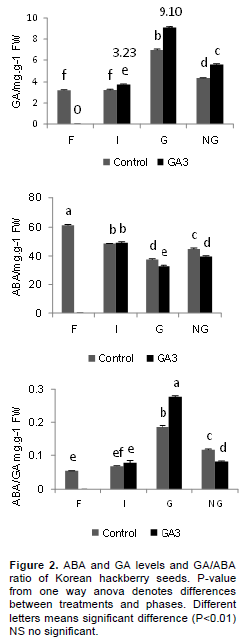
During germination, ZR and IAA were also detected in the Korean hackberry seeds. The ZR levels increased from F to G in both the control and with GA3 treatment, but there were no significant differences between them (Figure 3). The IAA concentration was higher in germinated seeds. The seeds treated with GA3 attained maximum levels of 59.71 ng.g-1 FW, and the IAA content differed significantly between the control and GA3 treatment (P<0.01;Figure 3). Major changes in the IAA/ABA ratio in the Korean hackberry seeds also showed the degree of breaking dormancy, with significant differences from F to G in both treatments. However, the differences between the control and GA3 treatment were only significant in the G phase.
Changes in storage substances
The concentration of total soluble sugar and starch in fresh seeds was 1.40 mg. (g DW)-1 and 1.28 mg.(g DW)-1, respectively. The content of sugar increased on germination, and after 5 months cold stratified, there were significant differences in the content of sugar in non-germinated seeds (Table 2; P<0.01). The concen-tration in GA3-treated seeds was higher than the control, but not significantly, except in the NG phase. One of the most remarkable things about this data was that the sugar concentration in non-germinated seeds was higher than in germinated seeds, especially on +GA3 treatment. We found that the content of starch also increased from the F to G phases, with a similar trend and no significant difference between the control and GA3 treatment (Table 2). For analyses of the protein, the concentration in fresh seeds was highest, with significant differences between the control and GA3 treatment. The results for the total amino acid content were the reverse, increasing from the F to the G phase. However, the amino acid decreased during imbibition. The values in F, I and NG (control and +GA3) were similar, being lower than in germinated seeds, and +GA3 treatment did not significantly affect the concentration of amino acid (Table 3).
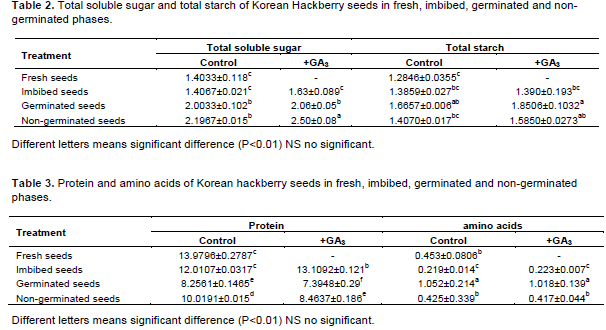
Widely used to increase germination in certain species, GA3 treatment has been shown to significantly promote germination in macaw palm seed (Ribeiro et al., 2011), with +GA3 treatment, seeds reaching maximum germination in four weeks, compared to control seeds at 18 weeks (Bicalho et al., 2015). However, there is no information on the effects of adding exogenous hormones on Korean Hackberry seeds germination. Here we found that Korean Hackberry seeds treated with GA3 performed better than the control, with a significantly higher germination percentage. These findings support that exogenous GA3 may be able to help break dormancy in Korean Hackberry seeds, even though GA3 treatment did not fully promote germination, and the criterion suitable for sowing (>30%) was not reached (Figure 1). Whether a higher concentration of exogenous gibberellic acid may increase germination requires further study. Moreover, environmental cues also strongly influence seed germination. The transition of seeds from dormancy to germination is mainly mediated by the balance between two competing hormones, gibberellins and abscisic acid (Steinbach et al., 1997; Debeaujon and Koornneef, 2000; White et al., 2000; Denise et al., 2014). ABA can inhibit seed germination by affecting the cell cycle and synthesis of cell wall hydrolases (Miransari and Smithc, 2013). The germination rate of switchgrass seeds has been found to be reduced in solutions containing ABA (Duclos et al., 2014), and the embryos of germinated seeds of macaw palm have been found to have lower ABA concentration than in non-germinated seeds (Bicalho et al., 2015). In our research, major differences were found in the ABA concentration in all phases, in line with published research, with a lower ABA concentration detected in G seeds and a decrease of ABA during imbibition (Ali-Rachedi et al., 2004). During the imbibition phase, storage products are mobilized, and the cell wall is loosened by mannanases and mannosidases (Bewley et al., 2013). This impairs ABA concentration and leads to the decrease of ABA during imbibition. In addition, we found the ABA concentration was significantly lower in germinated than non-germinated seeds after 5 months cold stratification with and without the addition of GA3. As stated by Barreto et al. (2014), the remaining ABA content in non-germinated seeds is related to dormancy maintenance and could protect seeds against biotic stress during storage. Our results confirmed that ABA could inhibit seed germination in Korean Hackberry seeds.
The plant hormone gibberellins are necessary for seed germination. Yamaguchi has showed that gibberellins stimulate the production of α-amylase, resulting in seed germination (Yamaguchi, 2008) and Ribeiro et al. (2014) suggested that GA increase has a central role in germination, with the absence of GA synthesis contributing to dormancy (Ribeiro et al., 2014). In our experiment, the concentration of GA significantly increased in germinated seeds, suggesting that GA does affect germination in Korean Hackberry seeds. In addition the highest concentration was observed in +GA3 treatment, therefore indicating that addition of exogenous hormones could stimulate the production of endogenous hormones.
Recently, it has been showed that control of seed dormancy may depend on the ratio of GA/ABA rather than the individual amounts of these two plant growth regulators. Bicalho et al. (2015) found that germinated seeds had no significant GA increase, but ABA concentration was significantly lower in embryos of germinated seeds compared to non-germinated seeds, resulting in an increased GA/ABA ratio. Chen et al. (2015) also found that the decrease in the ABA/GA ratio during cold stratification for 12 weeks correlated with dormancy break. In this study, the higher GA concentration and lower ABA concentration of germinated seeds clearly resulted in an increased GA/ABA ratio in the control and treated seeds.
ZR and IAA also play a role in seed germination. ZR are active in all stages of germination and may affect the activities of meristematic cells in roots and shoots. Chiwocha et al. (2005) found that increases in cytokinins (CK) concentration were associated with completion of germination and post-germination events. Bicalho et al. (2015) also confirmed that CK and auxins are directly involved in growth events and strongly participate in post-germination development. And the function of CK in cell cycle progression is reflected by changes of the levels of individual CK forms, especially of the most active on, ZR (Dobrev et al., 2002). Here we found that the ZR concentration increased in germinated seeds and was significantly different to other phases. This data suggests that the accumulation of ZR could prove effective in speeding up germination. In addition, GA3 application increased the content of GA and IAA in germinated seeds, but there was no change in ZR content.
Many plant physiologists state that auxin is not a necessary hormone for seed germination. However, auxin-related genesare expressed in the seed radicle tip during and after seed germination ( Miransari and Smithc, 2013). We also found that IAA concentration increased in the G phase, confirming previous research. Liu et al. (2007) has also suggested that IAA could inhibit the ABA pathway through the microRNA60. In this research, the change in concentration of IAA was totally reversed: the IAA/ABA ratio increased in germinated seeds.
In addition to changes in hormones, there are also changes in the storage products. It has been showed that, during cold stratification, the number of protein bodies in fresh seeds is reduced and the proteins cleaved to oligopeptides and free amino acids by active proteolytic enzymes (Chen et al., 2015). In an experiment with M. domestica seeds, proteins were hydrolyzed by proteolysis, and there was an increase in the quantity of free amino acids with an increase in seed germinability (Dawidowicz-Grzegorzewska, 1989). Our data indicates that the protein decreased and total free amino acids increased markedly in germinated seeds. However, the free amino acids decreased during imbibition phase: the amino acids were most likely used to synthesize more protein to break dormancy. The ABA concentration also decreased in imbibed seeds, this change probably increases storage product breakdown. The role of protein in Korean Hackberry seeds is not well known, but as stated by Staszak and Pawlowski (2014), when seed dormancy is established, proteins in seeds are involved in carbon metabolism, energy production and antioxidant processes.
The soluble sugars provide energy directly to support early seedling growth. Normally, the total soluble sugar content increases in line with seed germination, and includes fructose, sucrose, glucose and maltose. Horbowicz and Obendorf (1994) found that the levels of fructose, sucrose, glucose and maltose decreased during germination, and may be involved in supply for cell division, but the levels of raffinose and trehalose did not change. In contrast, Chen et al. (2015) found that the total soluble sugars increased in germinated seeds of A. morrisonense, but the amount of sucrose significantly decreased after the radicle emerged. In our experiment, after 5 months cold stratification, the sugar content was higher in non-germinated seeds than in the germinated seeds. This is probably because soluble sugar was catabolized when the radicle appeared, acting as the direct source of energy for the germinating seeds. In the seeds of Korean Hackberry, those non-reducing sugars probably could not make up the balance of total soluble sugar concentration. The total amounts of starch increased, but there were no significant differences in fresh, imbibed, non-germinated and germinated seeds (Table 2), giving no obvious correlation between them.
The greatest response relative to the seed coat treatment occurred in the seeds without endocarp (N, E and N, E, E) (Table 1). These results showed that endocarp could be decreasing germination. The effect of seed coat on germination has been studied in other species. Neil and Horgan (1987) have found the bracts of switchgrass can hinder seed germination by interfering with ABA oxidation through oxygen deprivation, and this was confirmed by Duclos et al., (2014). Similar results have been found in barley seeds (Bradford et al., 2008), and the hull of seeds have been found to regulate oxygen availability to the embryo, and also influence the synthesis of and sensitivity to ABA and GA, altering the balance of hormones and their potential action to either promote or retard germination (Finch-Savage and Leubner-Metzger, 2006). In our work, the seeds without any covering layers also had a higher germination rate. The seed endocarp creates a physical barrier to radicle protrusion and acts as a block to inhibit oxygen or moisture (Kucera et al. 2005). Further research is needed to identify whether Korean Hackberry seeds have a seed-coat dormancy mechanism as in yellow cedar.
Transition between dormancy and germination is mediated by hormones, being stimulated by ABA and GA, with the possible involvement of IAA and ZR. To some extent, exogenous hormones can stimulate seed germination rate, and increase the ratio of GA/ABA, even though the mechanism is not clear in Korean Hackberry seeds. In addition, the storage products in Korean Hackberry seeds are affected and may be directly involved in dormancy release. Seeds without any covering layers also germinated better than controls, a result which also implies interaction with plant hormones. Our research increases understanding of the dynamic changes in endogenous hormones, storage products and the influence of seed coat on germination of Korean Hackberry seeds, and can contribute to investigating the dormancy mechanism and development of an effective method to break dormancy.
The authors have not declared any conflict of interests.
This work was supported by the Tengtou Garden corporation Ningbo 315100, P. R. China and the Shandong agricultural seeds engineering major issue under No. 20106.
REFERENCES
Ali-Rachedi S, Bouinot D, Wagner MH, Bonnet M, Sotta B, Grappin P, Jullien M (2004). Changes in endogenous abscisic acid levels during dormancy release and maintenance of mature seeds: studies with the Cape Verde Islands ecotype, the dormant model of Arabidopsis thaliana. Planta 219:479-488.
Crossref
|
|
Bradford KJ, Benech-Arnold R, Côme D, Corbineau F (2008). Quantifying the sensitivity of barley seed germination to oxygen, abscisic acid and gibberellin using a population-based threshold model. J. Exp. Bot. 59:335-347.
Crossref
|
|
|
Bewley D, Bradford KJ, Hilhorst HWM, Nonogaki H (2013). Seeds-Physiology of development, germination and dormancy, 3rd edition. Springer, New York, USA. P 392.
|
|
|
Barreto LC, Garcia QS, Morales M, Muller M, Munne-Bosch S (2014). Vitamin E and defence-related phytohormones are reliable markers of embryo growth in macaw palm fruits exposed to various storage conditions. Plant Cell Tissue Organ Cult. 118:203-213.
Crossref
|
|
|
Bicalho EM, Pinto-Marijuan M, Muller M, Morales M, Muller M, Munne-Bosch S, Garcia QS (2015). Control of macaw palm seed germination by gibberellin/abscisic acid balance. Plant Biol. 17(2015):990-996.
Crossref
|
|
|
Chiwocha SD, Cutler AJ, Abrams SR, Ambrose SJ, Yang J, Ross AR, Kermode AR (2005). The etr1-2mutation in Arabidopsis thaliana affects the abscisic acid, auxin, cytokinin and gibberellin metabolic pathways during maintenance of seed dormancy, moist-chilling and germination. Plant J. 42:35-48.
Crossref
|
|
|
Conversa G, Lazzizera C, Elia A (2010). Effects of after-ripening, stratification and GA3 on dormancy release and on germination of wild asparagus (Asparagus acutifolius L.) seeds. Sci. Hortic. 125:196-202.
Crossref
|
|
|
Chen SY, Chou SH, Tsai CC, Hsu WY, Baskin CC, Baskin JM, Chien CT, Long L, Huang K (2015). Effects of moist cold stratification on germination, plant growth regulators, metabolites and embryo ultrastructure in seeds of Acer morrisonense (Sapindaceae). Plant Physiol. Biochem. 94(2015):165-173.
Crossref
|
|
|
Dawidowicz-Grzegorzewska A (1989). Degradation of protein and lipid bodies during dormancy removal in apple seeds. J. Plant Physiol. 135:43-51.
Crossref
|
|
|
Debeaujon I, Koornneef M (2000). Gibberellin requirement for Arabidopsis seed germination is determined both by testa characteristics and embryonic abscisic acid. Plant Physiol. 122:415-424.
Crossref
|
|
|
Dobrev P, Motyka V, Gaudinová A, (2002). Transient accumulation of cis- and trans-zeatin type cytokinin sand its relation to cytokinin oxidase activity during cell cycleof synchronized tobacco BY-2 cells. Plant Physiol. 40:333-337.
|
|
|
Duclos DV, Altobello CO, Taylor AG (2014). Investigating seed dormancy in switchgrass (Panicum virgatum L.): Elucidating the effect of temperature regimes and plant hormones on embryo dormancy. Ind. Crops Prod. 58(2014):148-159.
Crossref
|
|
|
Einali AR, Sadeghipour HR (2007). Alleviation of dormancy in walnut kernels bymoist chilling is independent from storage protein mobilization. Tree Physiol. 27:519-525.
Crossref
|
|
|
El-Maarouf-Bouteau H, Bailly C (2008). Oxidative signaling in seed germination and dormancy. Plant Signal. Behav. 3:175-182.
Crossref
|
|
|
Finch-Savage WE, Leubner-Metzger G, (2006). Seed dormancy and the control of germination. New Phytol. 171:501-523.
Crossref
|
|
|
Finkelstein R, Reeves W, Ariizumi T, Steber C (2008). Molecular aspects of seed dormancy. Plant Biol. 59:387-415.
Crossref
|
|
|
Horbowicz M, Obendorf RL (1994). Seed desiccation tolerance and storability: dependence on atulence-producing oligosaccharides and cyclitols-review and survey. Seed Sci. Res. 4:385-405.
Crossref
|
|
|
Koornneef M, Bentsink LH (2002). Seed dormancy and germination. Curr. Opin. Plant Biol. 5:33-36.
Crossref
|
|
|
Kucera B, Cohn MA, Leubner-Metzger G (2005). Plant hormone interactions during seed dormancy release and germination. Seed Sci. Res. 15:281-307.
Crossref
|
|
|
Li L, Ross JD (1990). Starch synthesis during dormancy breakage in oil seed of Corylus avellana. Ann. Bot. 66: 507-512.
|
|
|
Liu PP, Montgomery TA, Fahlgren N, Kasschau KD, Nonogaki H, Carrington JC (2007). Repression of AUXIN RESPONSE FACTOR10 by microRNA160 is critical for seed germination and post-germination stages. Plant J. 52:133-146.
Crossref
|
|
|
Miransari M, Smithc DL (2013). Plant hormones and seed germination. Environ. Exp. Bot. 99(2014):110-121.
|
|
|
Neil SJ, Horgan R (1987). Abscisic acid and related compounds. In Rivier L., Crozier, A. (Eds.). The Principles and Practice of Plant Hormone Analysis. Academic Press, London. pp 111-167.
|
|
|
Ribeiro LM, Souza PP, Rodrigues JR, Oliveira TGS, Garcia QS (2011). Overcoming dormancy in macaw palm diaspores, a ropical species with potential for use as biofuel. Seed Sci. Technol. 39:303-317.
Crossref
|
|
|
Ribeiro LM, Garcia QS, Muller M, Munne-Bosch S (2014) Tissue-specific hormonal profiling during dormancy release in macaw palm seeds. Physiologia Plantarum 153:627-642.
Crossref
|
|
|
Steinbach HS, Benech-Arnold RL, Sánchez RA (1997). Hormonal regulation of dormancy in developing Sorghum seeds. Plant Physiol. 113:149-154.
|
|
|
Staszak AM, Pawłowski TA (2014). Proteomic analysis of embryogenesis and the acquisition of seed dormancy in Norway maple (Acer platanoides L.). Int. J. Mol. Sci. 15:10868-10891.
Crossref
|
|
|
White CN, Proebsting WM, Hedden P, Rivin CJ (2000). Gibberellins and seed development in maize. I. Evidence that gibberellin/abscisic acid balance governs germination versus maturation pathways. Plant Physiol. 122:1081-1088.
Crossref
|
|
|
Yamaguchi S (2008). Gibberellin metabolism and its regulation. Ann. Rev. Plant Biol. 59:225-251.
Crossref
|
|