ABSTRACT
Soil physical and structural degradation may influence crop productivity over time in long-term no-tillage system areas. A field study was conducted at two sites, Palmeira das Missões and Não-Me-Toque, in southern Brazil to quantify soil physical/hydraulic and structural changes in zones with different yield potentials. The sites have been managed under no-tillage system without soil disturbance for more than 10 years. Soils were classified as Oxisols (Hapludox). Each site was divided into three zones with low, medium and high yield potentials based on overlapping of yield maps obtained from harvesters with precision agriculture tools. Within each yielding zone soil samples were collected to determine bulk density, porosity and aggregate stability. In addition, water infiltration rate and initial time for starting surface runoff were measured using a sprinkler infiltrometer (Cornell Sprinkler infiltrometer). Our findings showed that soils under low-yielding zones presented higher bulk density, lower macro-aggregate stability and water infiltration rate as well as shorter time for starting surface runoff compared to high-yielding zones. Therefore, these results suggest that soil physical and structural degradations have induced crop yield losses under long-term no-tillage areas. Macro-aggregate stability (>4.76 mm) and water infiltration rate were efficient parameters for distinguishing yielding zones in Oxisols managed under long-term no-tillage system in southern Brazil.
Key words: Soil compaction, Cornell Sprinkler Infiltrometer, soil aggregation, water infiltration.
The perpetuation of conservationist tillage systems is directly related to the soil and to the adopted crop management practices, which can enable the maintenance and improvement of soil structure quality (Dumanski, 2015) and crop yield (Pittelkow et al., 2015). Thus, the success of no-tillage systems depends on the maintenance of the following physical-hydraulic conditions: i) suitable soil water and air balance for plant growth (Lanzanova et al., 2010; Jemai et al., 2013; Palm et al., 2014, Nunes et al., 2015); ii) high rate of water infiltration into the soil (Golabi et al., 2014); iii) reduction of surface runoff (Sun et al., 2015).
In contrast, intensive machinery traffic during crop management practices is one of the major drivers of deleterious impacts on soil structural quality (Raper, 2005; Drescher et al., 2011; Barik et al., 2014). Soil aggregation is globally used as an indicator of soil structural quality changes induced by land use and management practices (Vezzani and Mielniczuk, 2011; Karlen et al., 2013). Macro-aggregates play crucial role on the stabilization and protection of soil organic matter (Six et al., 2000a), which increase the soil resilience to structural degradation and water dynamics in the soil (Vezzani and Mielniczuk, 2011). Studies have shown that increases of soil aggregation is positively correlated to higher yields of corn (Song et al., 2015), soybean (Corbin et al., 2010) and other cereals (Hou et al., 2012).
The determination of soil physical-hydraulic properties such as water infiltration rate can be important for understanding the causes of variability in crop productivity. According to Jégo et al. (2015), the knowledge of the spatial variability of crop yields requires a large amount of data about soil physical properties and their relationships that influence plant development. The relationship between soil water dynamics and crop yield can provide complementary information for understanding critical levels of soil porosity, resistance to penetration and bulk density (Kılıç et al., 2004; Whalley et al., 2008; Reichert et al., 2009).
Soil water infiltration can be determined using several methods, such as: tension infiltrometers or disc permeameters, pressure infiltrometers and sparkler infiltrometers. The method of concentric cylinders (pressure infiltrometer) uses a hydraulic load on the soil surface and has been used as a standard determination of infiltration, although some authors consider that this method overestimates the real water infiltration (Cheng et al., 2011). On the other hand, van Es and Schindelbeck (2003) stated that a sprinkler infiltrometer (Cornell Sprinkler infiltrometer) allows the determination of several important hydrological features, such as, the initial time for surface runoff, infiltration rate, accumulated infiltration and water consumption which are relevant characteristics in the evaluation of soil hydro-physical quality. These authors also mention the advantages of this method compared to double concentric rings, being of low cost in acquisition; convenience in transportation (small size and low weight), rapid assessment and operated by one person, easy calibration for different rainfall intensities, and low water consumption. Despite these advantages, the high coefficients of variation observed in the soil water infiltration data (Warrick and Nielsen, 1980) have limited use in commercial areas, since an accurate assessment requires a high number of field replications. In this sense, the adoption of precision agriculture tools makes possible to identify homogeneous areas with different yielding potential (Amado et al., 2009; Santi et al., 2013), and to investigate soil physical and hydraulic property changes within each one these yielding zones (Keller et al., 2012).
Although many technical papers have been published, changes on physical and hydraulic properties within different yielding zones in Brazil are still poorly documented. An on-farm study was conducted in southern Brazil, a pioneer region for the adoption of no-tillage (NT) system and precision agriculture tools. The objective of this study was to quantify soil physical/hydraulic and structural changes within zones with high-, medium- and low-yield potential. This research hypothesized that zones with higher crop yield have better soil physical/hydraulic and structural quality compared to zones of lower crop yield. Water infiltration rate assessed by sprinkler infiltrometer (Cornell Sprinkler infiltrometer) method is able to distinguish zones with different yield potentials.
Experimental field sites
The study was carried out in the Eastern Plateau of Rio Grande do Sul, Brazil at two experimental sites: i) Palmeira das Missões (PM), with area of 57.4 ha, at medium geographic coordinates, 27°53ʹS and 51º18ʹW and altitude of 600 m; and ii) Não-Me-Toque (NMT), with area of 132 ha, at coordinates 28°48ʹS and 52º77ʹW, and altitude of 550 m (Figure 1). The soils were classified as Oxisols (Hapludox) at both study sites (Santos et al., 2013). The average clay contents within each site were: i) PM - 730; 741 and 785 g kg-1; ii) NMT - 572; 616 and 518 g kg-1 within high-, medium- and low-yielding zones, respectively. The regional climate according to the Köppen´s global climatic classification is humid subtropical (Cfa), with an average annual temperature of 18.1 ºC and annual rainfall of the order of 1,900 mm.
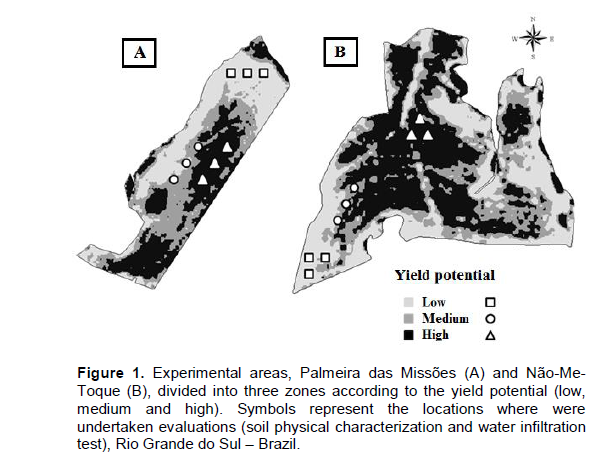
Both study sites have been managed with precision agriculture tools such as yield monitoring, systematic soil sampling and variable rate applications of fertilizers for five years and under NT system for more than ten years without interruption. In this work, we used the methodology described in Santi et al. (2013) overlapping the following sequences of crop yield maps for PM: soybean-corn-soybean-wheat-soybean-corn and NMT: corn-soybean-wheat-corn.
From the overlap of yield maps, each area were divided into three homogeneous zones according to their yield potential (high, medium and low), which was considered a low-yielding zone when showing up to 95% of the average crop yield in the total area, medium-yielding zone between 95 and 105% and high-yielding zone above 105% (Santi et al., 2013). Average grain yield of crops observed in both experimental areas were: i) PM: 2000/01 soybean (3,180 kg ha-1); 2001/02 corn (7,800 kg ha-1); 2002/03 soybean (3,240 kg ha-1); wheat 2003 (3,540 kg ha-1); 2003/04 soybean (2,220 kg ha-1) and corn 2004/05 (6,000 kg ha-1); ii) NMT: 2001/02 corn (5,640 kg ha-1); 2002/03 soybean (3,720 kg ha-1); wheat 2003 (2,700 kg ha-1) and corn 2004/05 (7,680 kg ha-1).
Sampling and determination of physical soil properties
In each study site, three representative sampling points within each yielding zone (high, medium and low yield) were selected (Figure 1). Undisturbed soil samples were taken using a metal cylinder (height 0.05 m x internal diameter 0.05 m), from the 0-0.05 and 0.05-0.10 m layers. At each zone five samples (replications) were collected, providing a total of 45 samples at each site. In addition, soil blocks of 0.05 x 0.05 m to 0.05 m depth were collected from the 0-0.05 and 0.05-0.10 m layers at each sampling point for soil aggregate stability analyses.
In the laboratory, the undisturbed soil samples were weighted (initial moisture), saturated with water and weighted again. The determination of the water content at -6 kPa (60 cm of water column) matrix potential was made on tension tables (Ball and Hunter, 1988). Afterwards, the soil samples were dried at 105oC for 48 h and weighed. Bulk density (BD) was calculated dividing the soil dry weight by volume of cylinder. The soil total porosity (TP, m3 m-3) was calculated by: TP = 1 - (BD/PD), where PD is particle density (2.65 Mg m-3). Soil macroporosity (Ma, m3 m-3) was estimated from the difference between water content in saturated soil and at -6 kPa. Soil microporosity (MiP) was estimated as the retained water content at -6 kPa soil matrix potential.
Field-moist soil was gently passed through an 8 mm sieve by breaking up the soil along natural planes of weakness, and air dried. The aggregate samples (25 g) were moistened slowly by capillarity and after 10 min, subjected to aggregate stability analysis using the standard wet-sieving method (Kemper and Chepil, 1965), in a vertical oscillator (Yoder, model MA-148 unit), at a speed of 30 oscillations per min for 10 min. The vertical oscillator consists of set of four sieves (4.76, 2.0, 1.00, and 0.21 mm mesh sizes). The aggregates were then separated into the following classes: 8.00-4.76; 4.76-2.00; 2.00-1.00; 1.00-0.21 and < 0.21 mm. The aggregates retained on each sieve were dried at ± 105 °C for 48 h for dry mass determination. After, the aggregates were chemically dispersed (NaOH 2% for 30s) for quantification of the mass of inert materials. Inert materials were subtracted from the respective aggregate size fractions.
Soil hydraulic properties
Around sampling points where undisturbed soil samples were taken, tests of water infiltration into soil were performed, with five replicates, providing a total of 45 measurements at each site. Water infiltration assessment was performed through a sprinkler infiltrometer ("Cornell Sprinkler infiltrometer"), described by van Es and Schindelbeck (2003). This infiltrometer is a portable rainfall simulator with a capacity of 20.6 liters composed of 69 drippers at the bottom, with a diameter of 0.00063 m and 0.19 m long each. This assembly is mounted on a cylinder with a diameter of 0.24 m, simulating different rain intensities by an air inlet regulation system.
To determine the water infiltration rate and the initial time for surface runoff a simulated rain of 300 mm h-1 was established for 60 min, as proposed by van Es and Schindelbeck (2003). Although the intensity is high for regional conditions, we chose to follow the original standard established by these authors. The rainfall intensity was determined from the expression:
Ri = (H1 - H2) / Ti (1)
Where: Ri = rainfall intensity (mm h-1); H1 and H2= initial and final reading (mm) of the water volume in the infiltrometer ruler, respectively; Ti= time interval (hours) between readings (we used 0.05 h in this study).
The surface runoff was determined by:
SR = [(Vt x 1000) / (45730 x t)] (2)
Where: SR= surface runoff (mm h-1), Vt= water volume collected (ml); 45730= ring area (mm2); t= time interval (hours) between the runoff collections (we used 0.05 h in this study).
The water infiltration rate was determined by:
WI = Ri – SR (3)
where: WI= water infiltration rate (mm h-1), Ri = rainfall intensity (mm h-1), SR= surface runoff (mm h-1).
At the moment of water infiltration measurements, the soil surface was fully covered by plant residues from previous winter crops and the volumetric soil water content was very similar among yield zones (that is, PM – 0.243, 0.234 and 0.241 m m-3; NMT – 0.253, 0.266 and 0.259 m m-3 at low- medium- and high-yielding zone, respectively).The equations of water infiltration rate and cumulative water infiltration were adjusted by non-linear and linear regressions, respectively, based on average values observed in each treatment, using Statistical Analysis System v.8.0 software (SAS Inc, Cary, USA). The surface runoff time was determined by intersection point between infiltration rate and surface runoff curves.
Soil physical properties
Bulk density was significantly lower under high-yielding zones compared to low-yielding zones for both sites at surface soil layer (0-0.05 m) and only for NMT at the deeper soil layer (0.05-0.10 m) (Table 1). Bulk density is widely used to investigate soil compaction and its deleterious impacts on plant growth (da Silva et al., 1994; Reynolds et al., 2009; Stolf et al., 2011; Salem et al., 2015). Within low- and medium-yielding zones bulk density reached values >1.2 Mg m-3 for both 0-0.05 and 0.05-0.10 m layers, which is considered a critical limit to suitable plant growth in clay soils (Reynolds et al., 2009). Therefore, soil compaction is likely a key factor that drives the productive potential losses in these zones. Under long-term no-tillage the absent of soil disturbance associated with intensive machinery traffic have induced critical soil compaction (Nunes et al., 2015), that leads to decreasing crop growth and yield, as reported by Pittelkow et al. (2015). Decreased productivity of the crops also decreases atmospheric CO2 uptake by the biomass (above and belowground), resulting in lower organic C inputs to soils over time, and to progressive process of soil physical degradation. Soil porosity attributes had small changes among yielding zones, though an overall non-significant tendency of higher macroporosity and total porosity in the high-yielding zones was verified (Table 1).
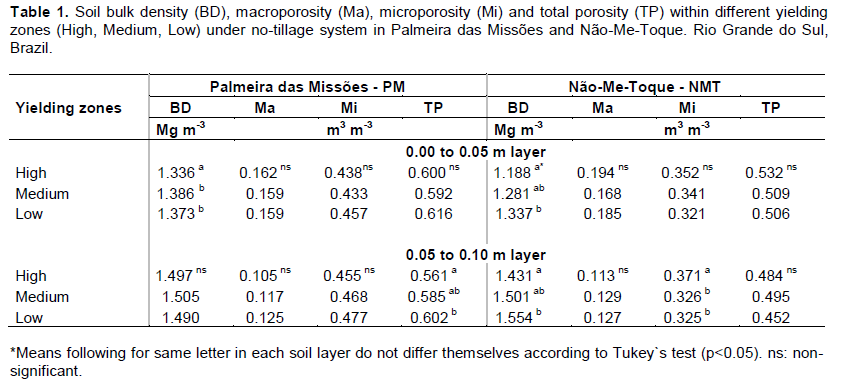
Overall, macro-aggregation stability was high regardless of the yielding zone for both sites (Figure 2). These results are typically reported in studies conducted in weathered Brazilian soils (Madari et al., 2005; Moraes et al., 2014; Cherubin et al., 2015), being associated primarily with mineralogical composition dominated by Fe and Al oxides and 1:1 minerals present in these soils (Silva and Mielniczuk, 1997; Six et al., 2000b). Despite that, large macro-aggregate fractions (>4.76 mm) were highly sensitive indicators to detect zones with different yield potentials, agreeing to reported values by O’Brien and Jastrow (2013). Soil macro-aggregates protect physically some organic matter fractions, resulting in pools with longer turnover times (Six et al., 2000a) that mediate soil physical processes related to water and air dynamics and providing resistance against soil erosion. In contrast, smaller aggregates fractions (<2 mm), which are units with lower structural complexity, increased under medium- and low-yielding zones. Therefore, significantly greater macro-aggregate values (Figure 2) and mean weight diameter values (Figure 3) under high-yielding zones for both sites indicate that soil physical and structural degradation have been negatively impacted on the productive potential of crops. Our findings suggest that improved soil structural quality provides better conditions for plant growth (roots and shoots) and consequently higher grain yield. Therefore, these areas have greater annual inputs of organic C into the soil, which acts as cementing agents throughout soil aggregation processes (Tisdall and Oades, 1982), and plants with larger and deeper root systems, which release exudates that have a cementing effect on soil particles, beyond the physical action in microaggregate formation via compressing action of growing roots and in the entanglement of soil particles to form and stabilize macro-aggregates (Tisdall and Oades, 1982; Bronick and Lal, 2005). In addition, greater biomass production led to higher amount of residues on soil surface, improving soil resistance to degradation (Wegner et al., 2015).
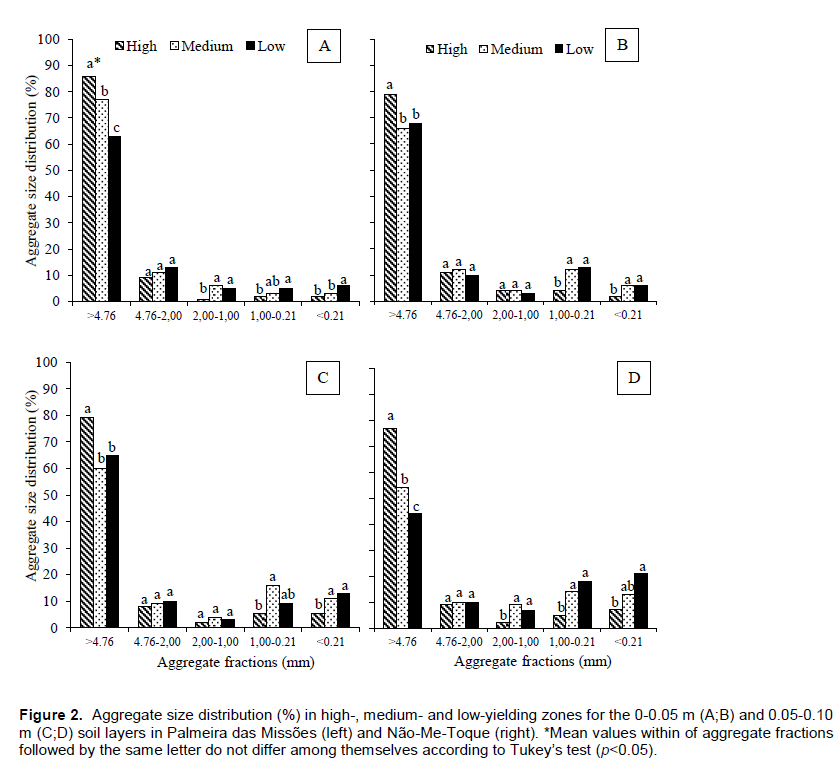
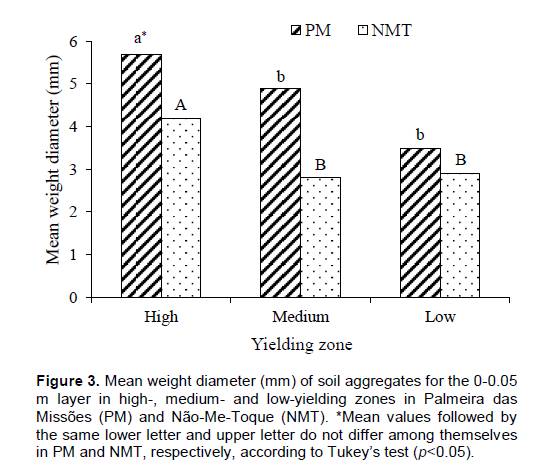
Water infiltration into the soil
The water infiltration rate and cumulative water infiltration within yielding zones at PM and NMT are shown in Figure 4. A significant decrease in water infiltration rates from high- to medium- and low-yielding zones was verified. Previously, Amado et al. (2009) also reported that zones of low yield potential had lower macroporosity and lower water availability for plants. Girardello et al. (2011) mentioned that zones of low yield potential had lower physical quality, expressed by higher soil density and lower total porosity, respectively, at 0.15-0.20 m depth, conditioning low water infiltration into the soil. These results indicate the distinction of current environmental offer between different yielding zones, confirming that in areas with a historical of high productivity have the highest water infiltration rates. Similarly, Keller et al. (2012) measured significantly lower field-saturated hydraulic conductivity in low-yielding zones than in high- and medium-yielding zones. Thus, water infiltration into soil is established as an important indicator of soil physical quality (Alves et al., 2007; Amado et al., 2009; Reichert et al., 2009).
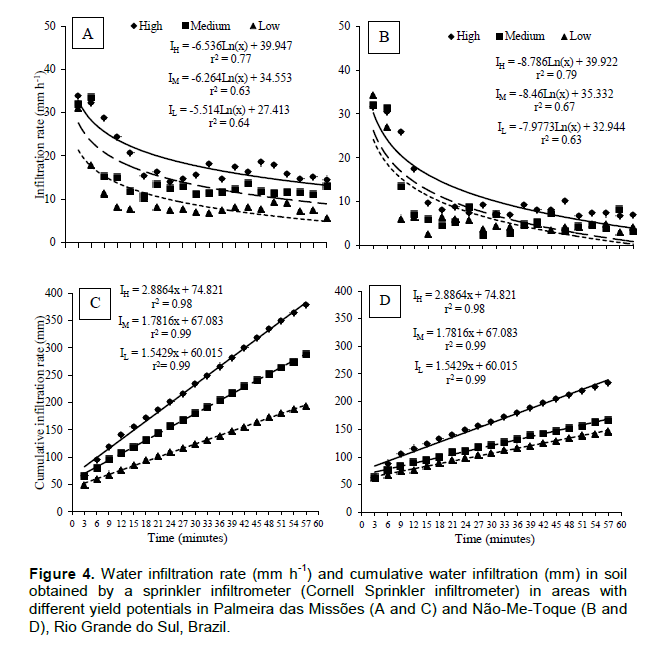
The coefficients of determination of the fitted regressions obtained in the two sites were > 0.60. These values are classified as satisfactory for water infiltration data, due to high spatial and temporal variability, assumed by the interrelationships involving intrinsic and extrinsic soil factors, which are undergo differentiated changes in time and space (Warrick and Nielsen, 1980; Tavares-Filho et al., 2006). According to Vieira et al. (2010) water infiltration in soil is usually quite variable due to variability of integrating texture, soil structure, biological activity, plant growth and uniformity of soil management practices.
In general, similar water infiltration rates were verified in PM and NMT, however, greater differences among yielding zones were observed in PM. The cumulative infiltration was higher in PM than in NMT, and again, greater differences among yielding zones was observed in PM. Both water infiltration rate and cumulative water infiltration values were higher within high-yielding zones than those quantified within medium- and low-yielding zones. In regard to sites, cumulative water infiltrations were lower in NMT compared to PM. This result may be associated with higher soil compaction in NMT area, at the time of assessment. According to Drescher et al. (2011), compacted soils have several hydrophysical changes with consequent damage to crop production capacity. The water infiltration is one of the phenomena that reflect hydrophysical soil conditions, because the structural quality leads to a pore size distribution favorable to root growth and water infiltration (Alves et al., 2007).
Surface runoff water
The initial time for surface runoff for each yielding zone at PM and NMT is shown in Figure 5. At PM, a simulated rainfall of 300 mm h-1 induced surface runoff after 35, 19 and 9 min within high-, medium- and low- yielding zones, respectively. At NMT, the surface runoff stated after 16, 10 and 8 min. These results suggest that under high-yielding zones water partition initially favors infiltration and reduces losses by runoff. These results gave more evidences that high-yielding zones have a greater soil physical structural quality and consequently improved soil quality, influencing positively crop yield (Amado et al., 2009).
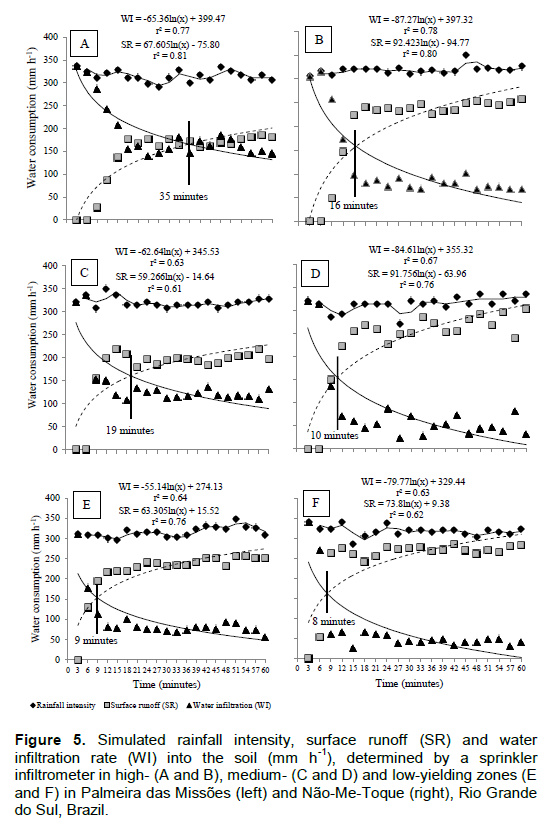
Based on our findings, we suggest that management strategies including cover crop with tap root system, crop rotation, or even mechanical chiseling should be adopted by farmers, especially under medium- and low-yielding zones, to improve soil physical and structural qualities, and consequently, to increase water infiltration rate, water storage and reduce surface runoff, as consistently reported in the literature (Lanzanova et al., 2010; Jemai et al., 2013; Sun et al., 2015).
This field study showed that soils under low-yielding zones presented higher bulk density, lower macro-aggregate stability and water infiltration rate as well as shorter time for starting surface runoff, compared to higher-yielding zones. Therefore, these findings suggest that soil physical and structural degradation have induced crop yield losses over time under long-term no-tillage areas.
Macro-aggregate stability (>4.76 mm) and water infiltration rate estimated by a sprinkler infiltrometer were efficient parameters for distinguishing yielding zones in Oxisols managed under long-term no-tillage system.
The authors have not declared any conflict of interests.
REFERENCES
Alves MC, Suzuki LGAS, Suzuki LEAS (2007). Densidade do solo e infiltração de água como indicadores da qualidade física de um Latossolo vermelho distrófico em recuperação. Rev. Bras. Cienc. Solo 31(4):617-625.
Crossref
|
|
Amado TJC, Pes LZ, Lemainski CL, Schenato RB (2009). Atributos químicos e físicos de Latossolos e sua relação com os rendimentos de milho e feijão irrigados. Rev. Bras. Cienc. Solo 33(4):831-843.
Crossref
|
|
|
Ball BC, Hunter R (1988). The determination of water release characteristics of soil cores at low suctions. Geoderma 43(2-3):195-212.
Crossref
|
|
|
Barik K, Aksakal EL, Islam KR, Sari S, Angin I (2014). Spatial variability in soil compaction properties associated with field traffic operations. Catena 120:122-133.
Crossref
|
|
|
Bronick CL, Lal R (2005). Soil structure and management: a review. Geoderma 124(1-2):3-22.
Crossref
|
|
|
Cheng Q, Chen Xi, Chen X, Zhang Z, Ling M (2011). Water infiltration underneath single-ring permeameters and hydraulic conductivity determination. J. Hydrol. 398(1-2):135-143.
Crossref
|
|
|
Cherubin MR, Karlen DL, Franco ALC, Cerri CEP, Tormena CA, Cerri CC (2015). A Soil Management Assessment Framework (SMAF) Evaluation of Brazilian Sugarcane Expansion on Soil Quality. Soil Sci. Soc. Am. J. 80(1):215-226.
Crossref
|
|
|
Corbin AT, Thelen KD, Robertson GP, Leep RH (2010). Influence of cropping systems on soil aggregate and weed seedbank dynamics during the organic transition Period. Agron. J. 102(6):1632-1640.
Crossref
|
|
|
da Silva AP, Kay BD, Perfect E (1994). Characterization of the least limiting water range. Soil Sci. Soc. Am. J. 58:1775-1781.
Crossref
|
|
|
Drescher MS, Eltz FLF, Denardin JE, Faganello A (2011). Persistência do efeito de intervenções mecânicas para a descompactação de solos sob plantio direto. Rev. Bras. Cienc. Solo 35(5):1713-1722.
Crossref
|
|
|
Dumanski J (2015). Envolving concepts and opportunities in soil conservation. Int. Soil Water Conserv. Res. 3(1):1-14.
Crossref
|
|
|
Girardello VC, Amado TJC, Nicoloso, RS, Hörbe TAN, Ferreira AO, Tabaldi FM, Lanzanova ME (2011). Alterações nos atributos físicos de um Latossolo vermelho sob plantio direto induzidas por diferentes tipos de escarificadores e o rendimento da soja. Rev. Bras. Cienc. Solo 35(6):2115-2126.
Crossref
|
|
|
Golabi MH, El-Swaify AS, Iyekar C (2014). Experiment of "no-tillage" farming system on the volcanic soils of tropical islands of Micronesia. Int. Soil Water Conserv. Res. 2(2):30-39.
Crossref
|
|
|
Hou X, Li R, Jia Z, Han Q, Wang W, Yang B (2012). Effects of rotational tillage practices on soil properties, winter wheat yields and water-use efficiency in semi-arid areas of north-west China. Field Crops Res. 129:7-13.
Crossref
|
|
|
Jégo G, Pattey E, Mesbah SM, Liu J, Duchesne I (2015). Impact of the spatial resolution of climatic data and soil physical properties on regional corn yield predictions using the STICS crop model. Int. J. Appl. Earth Obs. Geoinf. 41:11-12.
Crossref
|
|
|
Jemai I, Aissa NB, Guirat SB, Ben-Hammouda M, Gallali T (2013). Impact of three and seven years of no-tillage on the soil water storage, in the plant root zone, under a dry sub humid Tunisian climate. Soil Till. Res. 126:26-33.
Crossref
|
|
|
Karlen DL, Cambardella CA, Kovar JL, Colvin TS (2013). Soil quality response to long-term tillage and crop rotation practices. Soil Till. Res. 133:54-64.
Crossref
|
|
|
Keller T, Sutter JA, Nissen K, Rydberg T (2012). Using field measurement of saturated soil hydraulic conductivity to detect low-yielding zones in three Swedish fields. Soil Till. Res. 124:68-77.
Crossref
|
|
|
Kemper WD, Chepil WS (1965). Size distribution of aggregates. In: Black CA, Evans DD, White JL (Eds.). Methods of soil analysis. Pat 1. Madison, Am. Soc. Agron. pp. 499-509.
|
|
|
Kılıç K, Özgöz E, Akbas F (2004). Assessment of spatial variability in penetration resistance as related to some soil physical properties of two fluvents in Turkey. Soil Till. Res. 76(1):1-11.
Crossref
|
|
|
Lanzanova ME, Eltz FLF, Nicoloso RS, Amado TJC, Reinert DJ, Rocha MR (2010). Atributos físicos de um Argissolo em sistemas de culturas de longa duração sob semeadura direta. Rev. Bras. Cienc. Solo 34(4):1333-1342.
Crossref
|
|
|
Madari B, Machado PLOA, Torres E, Andrade AG, Valencia LIO (2005). No tillage and crop rotation effects on soil aggregation and organic carbon in a Rhodic Ferralsol from southern Brazil. Soil Till. Res. 80(1-2):185-200.
Crossref
|
|
|
Moraes MT, Silva VR, Cherubin MR, Carlesso R, Debiasi H, Levian R (2014). Changes in a Rhodic Hapludox under no-tillage and urban waste compost in the northwest of Rio Grande do Sul, Brazil. Rev. Bras. Cienc. Solo 38(4):1327-1336.
Crossref
|
|
|
Nunes MR, Denardin JE, Pauletto EA, Faganello A, Pinto LFS (2015). Effect of soil chiseling on soil structure and root growth for a clayey soil under no-tillage. Geoderma 260:149-155.
Crossref
|
|
|
O'Brien SL, Jastrow JD (2013). Physical and chemical protection in hierarchical soil aggregates regulates soil carbon and nitrogen recovery in restored perennial grasslands. Soil Biol. Biochem. 61:1-13.
Crossref
|
|
|
Palm C, Blanco-Canqui H, DeClerck F, Gatere L, Grace P (2014). Conservation agriculture and ecosystem services: An overview. Agric. Ecosyst. Environ. 187:87-105.
Crossref
|
|
|
Pittelkow CM, Liang X, Linquist BA, Groenigen KJ, Lee J, Lundy ME, Gestel N, Six J, Venterea RT, Kessel C (2015). Productivity limits and potentials of the principles of conservation agriculture. Nature 517:365-368.
Crossref
|
|
|
Raper RL (2005). Agricultural traffic impacts on soil. J. Terramechanics 42(3-4):259-280.
Crossref
|
|
|
Reichert JM, Suzuki LEAS, Reinert DJ, Horn R, Håkansson I (2009). Reference bulk density and critical degree-of-compactness for no-till crop production in subtropical highly weathered soils. Soil Till. Res. 102(2):242-254.
Crossref
|
|
|
Reynolds WD, Drury CF, Tan CS, Fox CA, Yang XM (2009). Use of indicators and pore volume-function characteristics to quantify soil physical quality. Geoderma 152(3-4):252-263.
Crossref
|
|
|
Salem HM, Valero C, Mu-oz MA, Gil-Rodríguez M (2015). Effect of integrated reservoir tillage for in-situ rainwater harvesting and other tillage practices on soil physical properties. Soil Till. Res. 151:50-60.
Crossref
|
|
|
Santi AL, Amado TJC, Eitelwein MT, Cherubin MR, Silva RF, Da Ros CO (2013). Definição de zonas de produtividade em áreas manejadas com agricultura de precisão. Rev. Bras. Cienc. Agrar. 8(3)510-515.
Crossref
|
|
|
Santos HG, Jacomine PKT, Anjos LHC, Oliveira VA, Oliveira JB, Coelho MR, Lumbreras JF, Cunha TJF (2013). Sistema brasileiro de classificação de solos. 3 ed. rev. ampl. Brasília - DF: Embrapa. 353 pp.
|
|
|
Silva IF, Mielniczuk J (1997). Ação do sistema radicular de plantas na formação e estabilização de agregados do solo. Rev. Bras. Cienc. Solo 21:113-117.
|
|
|
Six J, Elliott ET, Paustian K (2000b). Soil structure and soil organic matter: II. A normalized stability index and the effect of mineralogy. Soil Sci. Soc. Am. J. 64(3):1042-1049.
Crossref
|
|
|
Six J, Paustian K, Elliott ET, Combrink C (2000a). Soil Structure and Organic Matter I. Distribution of Aggregate-Size Classes and Aggregate-Associated Carbon. Soil Sci. Soc. Am. J. 64(2):681-689.
Crossref
|
|
|
Song Z, Gao H, Zhu P, Peng C, Deng A, Zheng C, Mannaf MA, Islam MN, Zhang W (2015). Organic amendments increase corn yield by enhancing soil resilience to climate change. Crop J. 3(2):110-117.
Crossref
|
|
|
Stolf R, Thurler AM, Bacchi OOS, Reichardt K (2011). Method to estimate soil macroporosity and microporosity based on sand content and bulk density. Rev. Bras. Cienc. Solo 35(2):447-459.
Crossref
|
|
|
Sun Y, Zeng Y, Shi Q, Pan X, Huang S (2015). No-tillage controls on runoff: A meta-analysis. Soil Till. Res. 153:1-6.
Crossref
|
|
|
Tavares-Filho J, Fonseca ICB, Ribon AA, Barbosa GMC (2006). Efeito da escarificação na condutividade hidráulica saturada de um Latossolo vermelho sob plantio direto. Cienc. Rural 36(4):996-999.
Crossref
|
|
|
Tisdall JM, Oades JM (1982). Organic matter and water stable aggregates in soils. J. Soil Sci. 33(2):141-163.
Crossref
|
|
|
van Es HM, Schindelbeck R (2003). Field procedures and data analysis for the Cornell Sprinkle Infiltrometer. Cornell University, Department of Crop and Soil Sciences. 8 p.
|
|
|
Vezzani FM, Mielniczuk J (2011). Agregação e estoque de carbono em Argissolo submetido a diferentes práticas de manejo agrícola. Rev. Bras. Cienc. Solo 35(1):213-223.
Crossref
|
|
|
Vieira SR, Brancalião SR, Grego CR, Martins ALM (2010). Variabilidade espacial de atributos físicos de um Argissolo vermelho-amarelo cultivado com leguminosas consorciada com a seringueira. Bragantia 69(2):423-432.
Crossref
|
|
|
Warrick AW, Nielsen DR (1980). Spatial variability of soil physical properties in the field. In: Hillel, D. Applications of soil physics. New York: Academic Press pp. 319-344.
Crossref
|
|
|
Wegner BR, Kumar S, Osborne SL, Schumacher TE, Vahyala IE, Eynarde A (2015). Soil response to corn residue removal and cover crops in eastern South Dakota. Soil Sci. Soc. Am. J. 79(4):1179-1187.
Crossref
|
|
|
Whalley WR, Watts CW, Gregory AS, Mooney SJ, Clark LJ, Whitmore AP (2008). The effect of soil strength on yield of wheat. Plant Soil 306(1):237-247.
Crossref
|
|