In Tunisia, more particularly in semiarid and arid areas, the drought accentuated by the surface evaporation of water lead to gradual increase in salinity of the soil which a major hurdal in development of vegetation. In these highly salted ecosystems, some plants are growing naturally; however, various species show different tolerance levels to salinity during their development. Seed germination is the stage which is most susceptible to this abiotic constraint. The aim of this study is to investigate the influence of increasing NaCl concentrations from 0 to 200 mM on the germination behavior of some halophytic plants species (Acacia cyanophylla, Acacia ampliceps, Medicago arborea, Hedysarum carnosum, Casuarina glauca and Ceratonia siliqua) whose seeds, were collected from Tunisian stands. The germination is evaluated through the daily and the cumulated rates of germination, the corrected seed germination rate and the recovery rate. The effect of salt stress revealed that the elevation of NaCl concentration induces a reduction of germination capacity as good as germination speed. In this setting, 200 mM concentrations of NaCl constitute a physiological limit of germination for all studied species. However, the interspecific variability is relatively important. According to the principal component analysis (PCA), seeds of C. glauca were the most salt tolerant, followed by M. arborea. Seeds of A. ampliceps were the least salt tolerant. The increase of the recovery rate with high salinity supposed that the latter has an osmotic reversible effect and not a toxic irreversible one on the studied species seeds.
Under natural conditions, plants are subjected to several types of environmental stress (biotic, abiotic). The impacts on crop productivity may be determined by one and/or the interaction of these components (Dong et al.
2015). Salinity in the soil is one of the non-living environmental stresses that affect crop production especially in arid and semiarid regions. Plants can develop strategies to grow in saline environments, but after successful seed germination, which is a key stage for plant establishment (Sattar et al., 2010). Salt stress is one of the most serious environmental factors limiting the germination of seeds and the growth of plants due to affecting the water and mineral status of the seeds and/or plants that leads to osmotic stress and an excess of sodium ions. Increase in salinity levels causes a reduction in seed germination percentage and delay germination process initiation, while salt stress can also cause complete inhibition of the germination process (Pujol et al., 2000).
The response of seeds germination to salinity has been reported to be more complex than plant growth because it depends on the availability of stored compounds. Several studies on the effect salinity on seeds germination focused on halophytes and non-halophytes (Joshi et al., 2005; Abari et al., 2011; Zhanwu et al., 2011). They responded to salt stress in similar way that achieves germination (Khajeh-Hosseini et al., 2003; El-Keblawy and Al-Rawai, 2005).
The inhibitory effect of salt stress on seeds germination is due to an osmotic effect and ion toxicity (Bajji et al., 2002; Tobe et al., 2004; Hajlaoui et al., 2007). Therefore, seeds may be generally less salt tolerant than mature plants (Dodd and Donovan, 1999). However, seeds of some halophytic plants may be more salt-tolerant than growing plants (Ungar, 1996). Bakhshi et al. (2013) demonstrated that germination factor such as germination rate and percentage was decreased with increasing NaCl concentration in some halophytic plants species.
Halophytes are potentially useful for ecological applications, such as landscaping, or rehabilitation of damaged ecosystems. They may also present economical interest as food, forage, or for production of metabolites. Laboratory investigations indicate that halophytes have adapted to saline habitats by their ability to adjust osmotically to increasing salinity levels (Gul et al., 2013).
Tunisia has a large diversity of halophytic plant species, found in different types of climax vegetation- in naturally occurring sabkhas (salt-flats) as well as saline soils and inland and littoral salt marshes. However, only limited published information is available on the status, biology and potential use of these plants.
In this study, we seek to provide detailed information on the germination responses of six halophytic plants species (Acacia cyanophylla Lindl., Acacia ampliceps Maslin, Medicago arborea L. Casuarina glauca Sieber ex. Spreng., Ceratonia siliqua L. and Hedysarum carnosum Desf.).
Therefore, the aim of this study is to evaluate and compare their germination performance under increasing levels of salinity, based on different germination parameters.
Seed source
Seeds of Acacia cyanophylla, Acacia ampliceps, Medicago arborea, Hedysarum carnosum, Casuarina glauca and Ceratonia siliqua are collected from Tunisian stands. After harvest, seeds are immediately stored at 5 °C.”.
Germination conditions
For each species seeds selected for uniformity (on the basis of colour and size), were surface sterilized with 0.1% HgCl2 for 5 min followed by thorough washing under tap water. All instruments for seed germination process were autoclaved at 121°C temperature on 15 PSI pressure for 20 min.
One hundred seeds of each species were placed in Petri dishes containing two layers of filter paper continuously moistened with distilled water or NaCl solutions (50, 100 and 200 mM). The Petri dishes were incubated at 25°C, in darkness. The treatments were replicated five times.
Seeds of A. cyanophylla, A. ampliceps and C. siliqua were previously immersed for one hour in boiled water prior to germination at all treatments. This pre-treatment is currently used to alleviate the mechanical restriction of the germination in softening the cover layers of the embryo. A seed was considered germinated when the tip of the radical had grown free of the seed coat (Wiese and Binning, 1987). Germinated seeds are counted daily until no more occurred for a period of three consecutive days.
To study the recovery of seed germination from NaCl treatments, the ungerminated seeds are immersed in distilled water for 1 h. Then, they are placed on filter paper in Petri dishes, moistened with distilled water and germinated at 25°C.
Parameters studied
At the end of experiment, the following germination parameters were recorded:
(i) Final germination percentage:
FGP = (number of germinated seeds/number of total seeds) × 100.
(ii) Germination rate index (GRI) was calculated following Esechie (1994):
GRI = [(G1/1) + (G2/2) + (Gx/X)]
Where, G = germination on each alternate day after placement , 1, 2, x = corresponding day of germination.
(iii) Corrected germination rate index (CGRI) was defined following Smith and Dobrenz (1987):
CGRI (%) = (A/B) * 100
Where: A: Number of seeds germinated in a given concentration of salt for 17 days, B: Number of seeds germinated in distilled water (control) for 17 days.
(iv) Recovery Rate (RR) of germination was calculated as indicated by Gulzar and Khan (2001):
RR(%) = [(a-b)/(c-b)] * 100.
Where: a: Number of seeds germinated after being transferred to distilled water, b: Number of seeds germinated in NaCl solution and c: Total number of seeds” describing the coefficient (c) of the formula of “Recovery Rate (RR) of germination.
Statistical analyses
One-way ANOVA is carried out to test for differences between species at a single salinity level and for differences between the effects of the different levels of salinity on a single species. When appropriate, differences between means are compared using Newman-Keuls test of Statistica version 5.5. P<0.05 is used to define statistical significance. Two-way ANOVA was used to test the effects of the main factors (species and salt stress) and their interactions on the different study parameters. Principal component analysis (PCA) was used to evaluate differences in salt tolerance between the study species. SPSS 13.0 for Windows was used to perform PCA. The PCA is an exploratory tool, useful for reducing the number of variables in a given data matrix by collapsing the dimensionality of the data. This was accomplished by projecting the original data onto new axes, or principal components (PCs). These PCs were ranked according to the amount of the variance of the original sample that was accounted for by each PC. PC 1 accounted for most of the variance, PC 2 accounted for the next greatest amount of variance and so on. The PCA was used to observe relationships between samples by plotting the scores of their PCs in two or more dimensions. Since the first two PCs accounted for the greatest amount of variance in the samples, they were typically the scores that were plotted (Schultz and Ducklow, 2000).
Cumulative seed germination
The evolution of germination percentage of seeds of six halophytic plants species under control and saline condition (50, 100 and 200 mM NaCl) was investigated. Significant difference was observed between treatments and all six halophytic plants species. For all studied species, NaCl treatments reduced the final germination percentages and retarded germination process (Figure 1). At 0 mM NaCl, seed germination was delayed by 1 day for Acacia cyanophylla, Acacia ampliceps, Medicago arborea and Hedysarum carnosum, by 3 days for Ceratonia siliqua and by 4 days for Casuarina glauca. At 50 mM NaCl, the germination delay does not change significantly. At 100 mM NaCl, C. siliqua shows a significant increase in the lag time (11 days). At 200 mM NaCl, all the studied species shows an increase in lag time. It was noted that 12 days lag time for A. cyanophylla, 9 days for C. siliqua, 5 days for M. arborea and C. glauca, 4 days for H. carnosum and 2 days for A. ampliceps (Figure 1). Also, the speed of germination is slower in saline solutions than in distilled water, where optimum germination percentage was reached after 15 days. The effect of salt stress on the delay in germination was reported for others halophytic plants species like Atriplex lentiformis, where the speed of germination is slower in salinity treatments than in control (Mahmood, 1995). The delay in germination was also noted for Hedysarum scoparium (Tobe et al., 2001) and Casuarina glauca (Liu et al., 2006). Some reports interpret the effects of salinity on the germination of seeds of different plant species in terms of osmotic potential, while others explain the reduction in germination as toxic effects of ionic elements (Poljakoff-Mayber et al., 1994). Indeed, the high intracellular concentrations of both Na+ and Cl- can inhibit the metabolism of dividing and expanding cells (Neumann, 1997), retarding germination and even leading to seed death (Zhang et al., 2010).
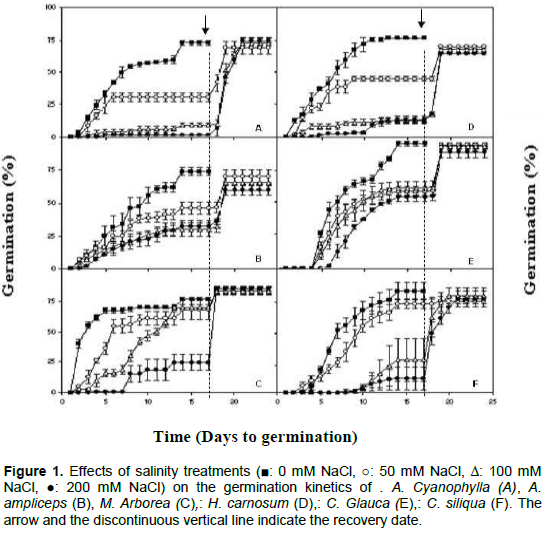
The highest germination percentage, in control samples and 200 mM, was observed in C. glauca. In five other species, the germination percentage was reduced signiï¬cantly in comparison with control values since the low dose of NaCl (50 mM). At higher NaCl concentrations (200 mM NaCl), germination was virtually suppressed in Acacia cyanophylla (Figure 1). These results are in agreement with other studies, salinity decreased the germination of Prosopis juliflora (El-Keblawy and Al-Rawai, 2005), Atriplex halimus (Abbad et al., 2004), Atriplex lentiformis (Mahmood, 1995), Atriplex griffithii (Khan and Rizvi, 1994; Khan and Ungar, 1997a), Casuarina equisetifolia, Medicago ruthenica (Guan et al., 2009), Halocnemum strobilaceum (Qu et al., 2008) and Hedysarum scoparium (Tobe et al., 2000).
Seed germination rate
For all the species studied, the germination rate index (GRI) was decreased significantly (P<0.05) with increasing salinity (Table 1).
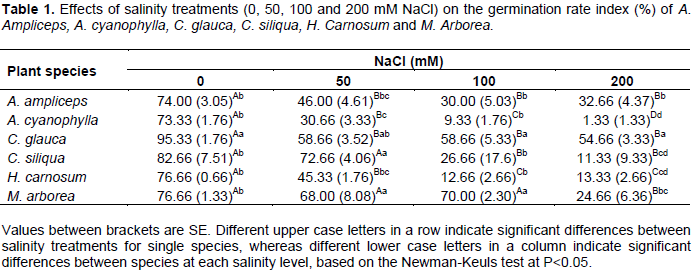
The highest values of germination rate were recorded on distilled water (control). They were statistically similar for all species, except C. glauca which showed the highest value of germination rate index. It was fairly around 95.33%. At 50 mM NaCl, the germination rate index was decreased significantly and more extensively in A. ampliceps, A. cyanophyllda, and H. carnosum compared to M. arborea, C. glauca and C. siliqua for which the germination rate index was respectively around 68, 58.66 and 72.66% while, for the first three species, it was only about 46, 30.66 and 45.33% respectively. At 100 mM NaCl, M. arborea and C. glauca showed the higher rates of germination than others species. They have respectively 70 and 58.66% of their seeds germinated. At 200 mM NaCl, only C. glauca has the highest final germination rate (54.66%) compared to other species. Moreover, under this condition (200 mM NaCl) the germination of A. cyanophylla appears to be hampered and she had the lowest final germination rate which did not exceed 1.33%. This is in agreement with those obtained by Debez et al. (2004) on Cakile maritima, Yildirim et al. (2011) on Physalis and Dallali et al. (2012) on two Hedysarum species.
Most of these studies suggested that seed germination of halophytic plants usually occurs when soil salinity levels are low and soil moisture is relatively high. Optimum germination of halophyte seeds is often obtained under freshwater and inhibited by increasing salinity concentrations (Gul et al., 2013), but the ability to germinate at higher salinities is varied with species (Table 1).
At these higher salinities, germination rate was decreased rapidly, which may be a result of osmotic stress or reduced enzyme activities, etc. Salt has been linked with the reduction of α-amylase activity in the seeds (Almansouri et al., 2001). Amylase is an important catalyst in the breakdown of starch reserves, which provides sugars for embryo growth and development.
Corrected seed germination rate
Similarly to the final seed germination rate, the corrected seed germination rate was reduced significantly (P<0.05) with increasing salinity for all the studied species (Table 2). At 50 mM NaCl, M. arborea and C. siliqua showed the highest percentages of corrected germination which reached respectively 89.07 and 88.25% At 100 mM NaCl, M. arborea has always preserved the higher corrected germination rate (about 91.45%) compared to others species whereas for C. Siliqua, the corrected seed germination rate dropped significantly to 30.35%. At the level of 200 mM NaCl, A. ampliceps and C. glauca illustrated the highest corrected germination rate with an average of about 43.92 and 57.47% respectively. Dealing with all treatments of salinity, A. cyanophylla presented more reduction in germination in response to salt stress than the other species (Table 2). Indeed for this last, the corrected seed germination rate was around 41.91, 12.80 and 1.75% respectively at treatments of 50, 100 and 200 mM NaCl.
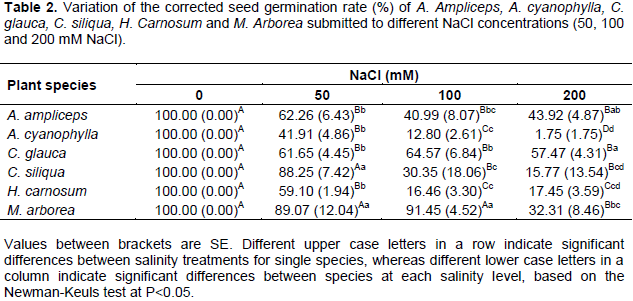
Like the final germination rate, the corrected germination rate shows that the increase in salinity progressively inhibited germination. In agreement with previous results concerning halophytic species seeds, the imposition of salt stress resulted in decreases of both percentage and germination rates (Lachiheb et al., 2004; Sekmen et al., 2012). According to Prado et al. (2000), the decrease in germination may be ascribed to an apparent osmotic ‘dormancy’ developed under saline stress conditions, which may represent an adaptive strategy to prevent germination under stressful environment.
Correlation between the corrected germination rate and the lag time
The relationship between the corrected germination rate and the lag time is depicted in Figure 2. The results indicated that these parameters are relatively dependent. Indeed, the corrected germination rate decreased and lags time was increased with increasing NaCl concentration. For the two salinity treatments 50 and 100 mM NaCl, the relation between the corrected germination rate and the lag time is not strong. In fact, the correlation coefficient (R2) values were respectively about 0.0481 and 0.0574, whereas, for 200 mM NaCl, this relation becomes relatively strong (R2=0.5003) (Figure 2). Seeds of A. cyanophylla, A. ampliceps, M. arborea and H. carnosum have trivial primary dormancy as reflected by 1-day lag time at control treatment, whereas those of C. siliqua and of C. glauca showed significant primary dormancy. This last can be related to various inhibitors (Baskin and Baskin, 1998). The secondary dormancy, as indicated by germination delay under salinity treatments, strongly increased at 100 mM NaCl and was rather amplified at 200 mM NaCl. At this highest level of salt stress the relationship between the corrected germination rate and the lag time was linear, or close to being linear, which suggests a simple physical cause, and may be indicative that osmotic limitation is important in determining the germination rate. So what, delaying the germination of seeds and the increase of the lag time germination of studied species with increasing salt concentration is explained by the time required for the seed to establish mechanisms allowing it to adjust its internal osmotic pressure.
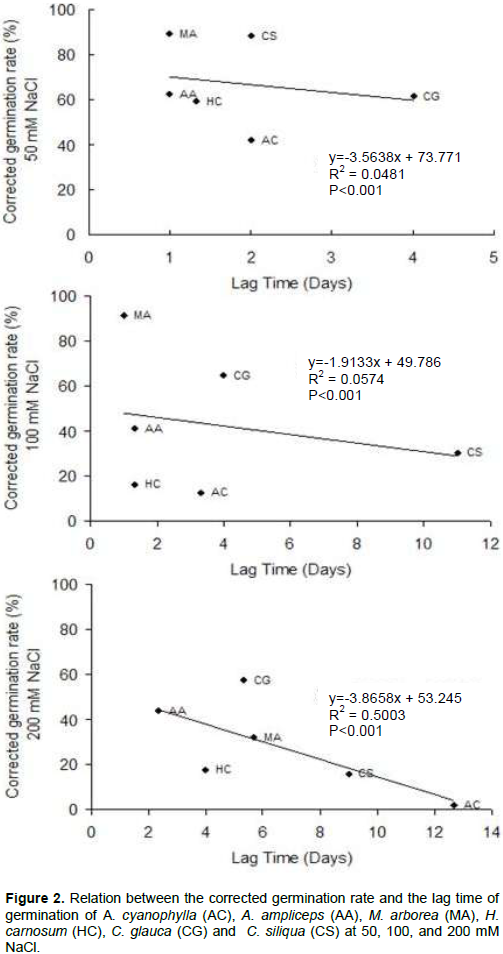
Recovery rate of seed germination
Transfer experiments of seeds to control medium after pretreatment with NaCl were conducted to clarify the mode of action of salt stress on germination. Our results (Table 3) showed that the recovery responses vary from one species to the other and against the level of salinity they are exposed. Indeed, the recovery rate of seeds of A. cyanophylla, H. carnosum and C. siliqua was increased significantly (P<0.05) with increasing salinity. This increase remains more pronounced specially in seeds of C.siliqua where the recovery rate of germination raised from 7.67% at 50 mM NaCl to 71.16 and 71.56% respectively at 100 and 200 mM NaCl. Moreover we noticed also that the recovery rate does not differ significantly between A. ampliceps, M. arborea and C. glauca among the NaCl treatments exceptionally at 200 mM, where A. ampliceps presented the lowest recovery rate with an average of about 40.71% (Table 3). At 50 mM NaCl, C. glauca has the highest recovery rate, whereas C. siliqua has the lowest value. All the other species have intermediate rates. At 100 mM NaCl, no significant difference is noted between studied species for the recovery rate.
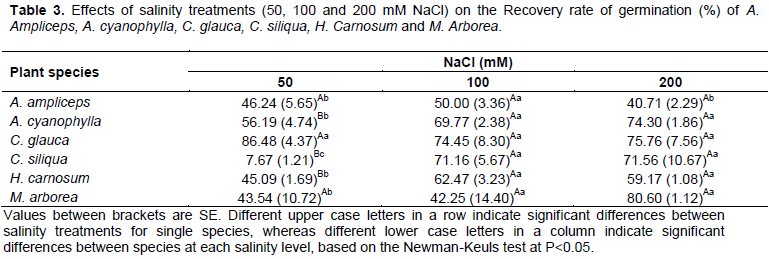
The increase of the recovery rate with increasing salinity seems to be a general rule. In this setting, Khan and Ungar (1997b) reported that halophyte seeds have typicallythe ability to maintain seed viability for extended periods of time during exposure to hyper-saline conditions and then to commence germination when salinity stress is reduced or removed. Most halophytes show an important recovery of germination when stress conditions are alleviated (Khan and Ungar, 1997b; Pujol et al., 2000; Gulzar and Khan, 2001; Qu et al., 2008). This may have ecological significance within highly saline environments, reflecting a physiological response that is understrong selection pressure during the evolution of these species. Moreover, seed survival under hypersaline conditions rather than germinability has been used as criteria for salt tolerance. Survival skills of halophyte seeds which was observed in C. Glauca and M. arborea under hypersaline conditions gives a selective advantage to halophytes and distinguishes them from most of glycophytes (Sekmen et al., 2012).
Previous studies that reported that a recovery of germination upon transfer of seeds from NaCl solution to pure water generally attribute the initial repression of germination and its subsequent recovery to osmotic factors, without exploring alternative hypotheses, such as the export of sodium from the seeds upon transfer to pure water (Debez et al., 2004). Likewise, it is unclear whether the high sodium concentrations seen here in non-germinating seeds are physiologically relevant (that is, a causal factor), or simply a result of the longer duration that non-germinating seeds spent in their respective solutions (Zhang et al., 2010).
Our data conï¬rm the assumption that salinity usually impairs germination by preventing imbibition (Keiffer and Ungar, 1995). Nevertheless, at higher salinity (200 mM NaCl) toxicity phenomena due to the accumulation of Na+ and Cl- ions, have also appeared, as evidenced by the decline in germination recovery, compared to the control, especially for A. ampliceps seeds.
Discrimination of interspecific variability and interactions species x salt stress
The analysis of variance examining the germination rate index, corrected germination and recovery rates after imposing salt stress are presented in Table 4. Results of this analysis showed that the effect of the salinity level on germination indices is more significant than that of the plant species. The interaction between the two factors (salinity and species) is also significant, but at lower extent. However, the interaction affects the recovery rate rather than the two other rates.
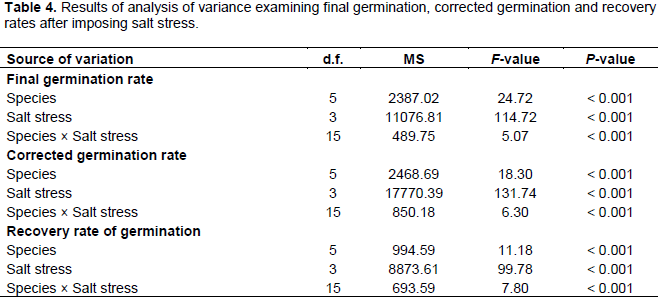
The data processing by PCA is presented in Figure 3. The first two axes of the PCA represented 80.65% of the total variability. The first principal component (PCA 1) expressed 57.06%, while the second axis (PCA 2) accounts for 23.59% of the total variability. PCA 1 seemed to separate the scores of the control treatment from that of the other NaCl treatments. PCA 2 seemed to separate the scores of the best salt tolerant species than that of the worst.
Overall and from the positive side to the negative side of PCA 1, the germination performance was decreased with increasing salinity. Seeds germinated in 0 mM NaCl (control) recorded the greatest contribution to the PCA 1 and thus, they perform better than the other treatments. The graphical representation of the dispersion, of studied
species, in the diagram generated by the first two axes of PCA (1-2) showed the existence of a large interspecific variability. Globally, seeds of C. glauca showed to be the most salt tolerant. However, A. cyanophylla was the most sensible to NaCl treatment. A. ampliceps was the least performant species. For more details and depending on the PCA analyses, it can be make a classification that takes into consideration the germination behaviour of studied species at the level of each salinity treatment. At 50 mM NaCl, the salt tolerance of the study species’ seeds can be classified as follows: C. glauca > M. arborea > A. ampliceps > H. carnosum > C. siliqua > A. cyanophylla. At 100 mM NaCl, the salt tolerance of the study species’ seeds can be classified as follows: C. glauca > M. arborea > C. siliqua > A. cyanophylla > H. carnosum > A. ampliceps. Finally, at 200 mM NaCl, the salt tolerance of the study species’ seeds can be classified as follows: C. glauca > M. arborea > H. carnosum > C. siliqua > A. cyanophylla > A. ampliceps. Thus, seeds of C. glauca were the most salt tolerant, followed by M. arborea. Seeds of A. ampliceps were the least salt tolerant.
The considerable interspecific variability in germination behaviour of halophytic plants species with increasing salinity level was reported by Keiffer and Ungar (1995); Lachiheb et al. (2004) and Gul et al. (2013). For example, Keiffer and Ungar (1995) exposed seeds of five halophytes (Atriplex prostrata, Hordeum jubatum, Salicornia europaea, Spergularia marina and Suaeda calceoliformis) to an extended period of salinity treatments. They used Woodell (1985) classification system and placed Atriplex prostrata seeds in the Type 1, Hordeum jubatum and Spergularia marina in the Type 2, and Salicornia europaea and Suaeda calceoliformis in the Type 3 category.