ABSTRACT
The lack and/or inconsistent behavior of results found in literature have limited Azospirillum use in maize crops. In this regard, this study aimed at evaluating the performance and chlorophyll a fluorescence of maize hybrids inoculated with Azospirillum brasilense under different nitrogen levels and protected crop conditions with nutrient solution. The experiment was conducted in a greenhouse at Instituto Federal Goiano, Rio Verde Campus. Adapted jars from "Leonard" were used in the experiment. For each bottle, 1.5 kg of substrate with washed sand and vermiculite in a proportion of 1:1 and nutrient solution of Sarruge was used. The experiment was conducted in a completely randomized design in a 4 × 2 × 2 factorial arrangement with four replications, totaling 160 treatments: four maize hybrids: simple hybrid– H1 (NS 90); modified simple hybrid– H2 (AS 1581); double hybrid– H3 (DKB 310); triple hybrid– H4 (ATL 310); with absence and presence of N (NP and NA) and absence and presence of inoculation (IP and IA). Physiological evaluations of chlorophyll a fluorescence using a modulated portable fluorometer, MINI-PAM model were performed at the end of the experiment. Initial fluorescence (F0), maximum fluorescence (Fm), potential quantum yield (Fv/Fm) variable fluorescence/maximum fluorescence), effective quantum yield (ΔF/Fm’), photochemical dissipation (qp), non-photochemical dissipation (qn and NPQ) and electron transport rate (ETR) were determined. On average, there was a difference in ETR of about 34% between treatments. For H4, it was observed that Fv/Fm was lower with inoculant when compared with plants without inoculation, a difference of approximately 11%, on average, was observed in value of Fv/Fm ratio. Due to better efficiency in absorption and utilization of nitrogen promoted by bacteria (A. brasilense), inoculated maize hybrids showed a good photosynthetic performance.
Key words: biological nitrogen fixation, nitrogen economy, maize hybrids.
Maize (Zea mays L.) is one of the most important consumed cereals in the world due to its productive potential and nutritional value and can be used both in food and feed (Fancelli, 2011). In Brazil, large maize areas are cultivated, about 16 million ha (crop and off-season or second crop), concentrated mainly in the states of South, Southeast and Midwest, with average national productivity of about 4.4 tha-1 (Conab, 2013). In off-season maize crop, the recommendation to lower amounts of fertilizers is routine. This is justified by the lower yield potential due to higher climate risks, such as low water and heat availability and less solar radiation (Shioga et al., 2004).
Nitrogen is the most important and limiting mineral in maize yields, requiring its application in large quantities to meet crop demand. Nitrogen is one of nutrients that have the most significant effect on increased grain yield in maize. It has great importance as constituent of protein molecules, enzymes, co-enzymes, nucleic acids and cytochromes as well as its important role as a member of chlorophyll molecule (Gross et al., 2006). According to research of Fancelli (2011), nitrogen deficiency may affect grain yield from 14 to 80%, and also reduce its protein content. The management of nitrogen fertilizations is one of the most complexes due to factors related to nitrogen fertilizer cost due to problems in the efficiency of some sources (Menezes, 2004). This nutrient is characterized by having one of the highest loss indexes, which may occur by leaching, surface runoff, erosion, volatilization and ammonium denitrification. The higher or lower loss index can be controlled by implementation, management and nutrient source to be used (Queiroz et al., 2011). Its availability in soil depends on organic matter content, climatic factors which are difficult to predict, C/N ratio and microbial activities. Nitrate (NO3-) and ammonium (NH4+) are the more readily absorbed forms by plants (Camargo and Sá, 2004). Each ton of produced grain demands on average 16.4 kg of N, which 64% is exported to grain (Setiyono et al., 2010).
Thus, due to growing demand for sustainability in agricultural production systems, some authors have presented biological nitrogen fixation (BNF) as an alternative to nitrogen fertilizer saving, which can supplement or even replace this fertilizer use (Bergamaschi, 2006).
Biological fixation may be responsible for approximately 65% of total N set on Earth, therefore it is the second most important biological process after photosynthesis (Cantarella, 2007). Among the N fixer microorganisms found in association with grass roots of species of Azospirillum genus are one of the most studied groups (Hartmann and Baldam, 2006). Although, Azospirillum genus is consisted of seven species, the most extensively studied for the use on inoculation is Azospirillum brasilense (Huergo et al., 2008). Despite the potential use of this technique, the application of diazotrophic A. brasilense through solution in seeds, with or without synthetic nitrogen rates, does not interfere with plant development and maize crop yield. The adoption of this practice does not replace the use of nitrogen fertilizers and neither allows rate reduction (Repke et al., 2013).
Plants inoculated with Azospirillum showed a higher rate of photosynthesis and of stomatal conductance, resulting in higher grain yield when compared with non-inoculated plants, as well as the nutrient content in the grains was higher in inoculated plants (Saikia et al., 2007). The lack of results and inconsistent behavior of results found in literature and that of Bartchechen et al. (2010) may vary according to the cultivar, climatic conditions, research methodology and has limited the use of Azospirillum in maize crop.
In this regard, this study aimed at evaluating the performance and chlorophyll a fluorescence of maize hybrids inoculated with A. brasilense under different nitrogen levels and protected crop conditions with nutrient solution.
Maize (Zea mays L.) is one of the most important consumed cereals in the world due to its productive potential and nutritional value and can be used both in food and feed (Fancelli, 2011). In Brazil, large maize areas are cultivated, about 16 million ha (crop and off-season or second crop), concentrated mainly in the states of South, Southeast and Midwest, with average national productivity of about 4.4 tha-1 (Conab, 2013). In off-season maize crop, the recommendation to lower amounts of fertilizers is routine. This is justified by the lower yield potential due to higher climate risks, such as low water and heat availability and less solar radiation (Shioga et al., 2004).
Nitrogen is the most important and limiting mineral in maize yields, requiring its application in large quantities to meet crop demand. Nitrogen is one of nutrients that have the most significant effect on increased grain yield in maize. It has great importance as constituent of protein molecules, enzymes, co-enzymes, nucleic acids and cytochromes as well as its important role as a member of chlorophyll molecule (Gross et al., 2006). According to research of Fancelli (2011), nitrogen deficiency may affect grain yield from 14 to 80%, and also reduce its protein content. The management of nitrogen fertilizations is one of the most complexes due to factors related to nitrogen fertilizer cost due to problems in the efficiency of some sources (Menezes, 2004). This nutrient is characterized by having one of the highest loss indexes, which may occur by leaching, surface runoff, erosion, volatilization and ammonium denitrification. The higher or lower loss index can be controlled by implementation, management and nutrient source to be used (Queiroz et al., 2011). Its availability in soil depends on organic matter content, climatic factors which are difficult to predict, C/N ratio and microbial activities. Nitrate (NO3-) and ammonium (NH4+) are the more readily absorbed forms by plants (Camargo and Sá, 2004). Each ton of produced grain demands on average 16.4 kg of N, which 64% is exported to grain (Setiyono et al., 2010).
Thus, due to growing demand for sustainability in agricultural production systems, some authors have presented biological nitrogen fixation (BNF) as an alternative to nitrogen fertilizer saving, which can supplement or even replace this fertilizer use (Bergamaschi, 2006).
Biological fixation may be responsible for approximately 65% of total N set on Earth, therefore it is the second most important biological process after photosynthesis (Cantarella, 2007). Among the N fixer microorganisms found in association with grass roots of species of Azospirillum genus are one of the most studied groups (Hartmann and Baldam, 2006). Although, Azospirillum genus is consisted of seven species, the most extensively studied for the use on inoculation is Azospirillum brasilense (Huergo et al., 2008). Despite the potential use of this technique, the application of diazotrophic A. brasilense through solution in seeds, with or without synthetic nitrogen rates, does not interfere with plant development and maize crop yield. The adoption of this practice does not replace the use of nitrogen fertilizers and neither allows rate reduction (Repke et al., 2013).
Plants inoculated with Azospirillum showed a higher rate of photosynthesis and of stomatal conductance, resulting in higher grain yield when compared with non-inoculated plants, as well as the nutrient content in the grains was higher in inoculated plants (Saikia et al., 2007). The lack of results and inconsistent behavior of results found in literature and that of Bartchechen et al. (2010) may vary according to the cultivar, climatic conditions, research methodology and has limited the use of Azospirillum in maize crop.
In this regard, this study aimed at evaluating the performance and chlorophyll a fluorescence of maize hybrids inoculated with A. brasilense under different nitrogen levels and protected crop conditions with nutrient solution.
The experiment was conducted in a controlled environment with temperature and relative humidity of 27°C and 70%, respectively, at Instituto Federal Goiano, Rio Verde Campus, located in the southwestern of the state of Goiás, situated at 17° 47’ 53’’ North latitude and 51° 55’ 53’’ South latitude with 743 m of altitude.
Adapted Leonard jars were used (Vincent, 1970) for experiment conduction, however in order to maintain sterile conditions, "pet" bottles were cut with scissors, 14 to 15 cm height from base. Subsequently, an aseptic process immersed in sodium hypochlorite (5%) for 1 h was performed. After this period, material was rinsed in running water with distilled water and autoclaved.
To each bottle, 1.5 kg of substrate with washed sand and vermiculite in a proportion of 1:1 was used. It were placed in polyethylene plastic bags and washed for sterilization in autoclave for 1 h at 121°C, for two consecutive days. Autoclaved mixture was placed on top of bottle, and nutritive solution of Sarruge (1975) was inserted at the bottom, according to Table 1. And afterwards, pots were covered with plastic bags paper of "kraft" type in order to prevent algae development
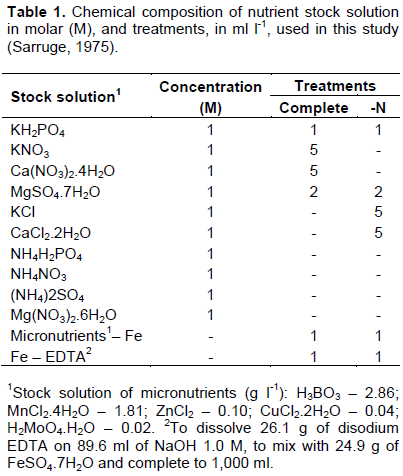
Maize seeds were surface disinfected for 2 min with ethyl alcohol at 70% and sodium hypochlorite at 2% for 3 min (Hungria et al., 1994), then washed for 10 times in distilled water and autoclaved. Two seeds per pot were planted and after seven days of germination, manual thinning of excess plants was performed, leaving only one plant per pot.
The experiment was conducted in a completely randomized design in a 4 × 2 × 2 factorial arrangement with four replications, totaling 160 treatments: four maize hybrids (simple hybrid– H1; modified simple hybrid– H2; double hybrid– H3; triple hybrid– H4); with presence and absence of N (NP and NA); and presence and absence of inoculation (IP and IA).
Maize hybrids were chosen according to materials for planting in the region, which are widespread among crop producers in the Southwestern of the State of Goiás, (H1–NS 90; H2–AS 1581; H3–DKB 310 and H4–ATL 310), N supply was in accordance with nutrient solution (Table 1) and inoculation with Azototal® Azospirillum brasilense based commercial product (AbV5 and AbV6 strains) and was performed at sowing time with 100 mL of liquid inoculate for each 25 kg of seed with 2.0 × 108 CFU ml-1 guaranteed, applied and directly homogenized in seeds, according to the Manufacturer’s specifications.
Nutrient solution was prepared in a quantity sufficient to fill the bottom of jars. A total of 200 mL of nutrient solution per pot was used. Different solutions were performed ​​according to treatments (with N "complete solution” and without N “solution – N"). Solution was remade every time that a replacement was necessary.
Physiological assessments concerned with chlorophyll a fluorescence were performed at 35 days after emergence, when all hybrids reached a total of four expanded leaves, recommended time to perform nitrogen fertilizer application. The chlorophyll a fluorescence measures were obtained using modulated portable fluorometer, MINI-PAM model (Walz, Effeltrich, Germany), equipped with a special clamp to model sheet support 2030-B (Bilger et al., 1995; Rascher et al., 2000). Initial fluorescence (F0), maximum fluorescence (Fm), potential quantum yield (Fv/Fm = variable fluorescence/maximum fluorescence), effective quantum yield (ΔF/Fm’), photochemical dissipation (qp), non-photochemical dissipation (qn and NPQ) and electron transport rate (ETR) were used. The maximum quantum efficiency of photosystem II (Van Kooten and Snel, 1990) was calculated after 30 min of dark adaptation using equation Fv/Fm = (Fm-F0)/Fm; where F0 is the minimum fluorescence yield, excited by a low intensity modulated red light (0.03 mmol m-2 s-1), and Fm is the maximum fluorescence obtained by applying 0.8 s pulse of saturating actinic light (>6,000 μmol m-2 s-1). The effective quantum efficiency of photosystem II (Genty et al., 1989) will be determined by overlapping a saturation pulse in leaves prior adapted to ambient light, calculated as ΔF/Fm’= (Fm’-F) /Fm’; where F is the maximum fluorescence yield during saturation pulse. The ΔF/Fm’ is used to estimate the apparent rate of electron transport (ETR). According to Bilger et al. (1995) by using the equation ETR = ΔF/Fm’ × PFD × 0.5 × 0.84, which PFD is the photon flux density (μmol m-2 s-1) incident on sheet; 0.5 is the corresponding value to fraction of excitation energy distributed to FSII (Laisk and Loreto, 1996); and 0.84 is the corresponding value to fraction of incident light that is absorbed by leaves (Ehleinger, 1981). The non-photochemical coefficient of Stern-Volmer was calculated as NPQ = (Fm, Fm')/Fm' (Bilger and Bjorkman, 1990). The chlorophyll a fluorescence measurements occurred in the period between 7 and 10 a.m.
Data were tabulated and submitted to analysis of variance by F test (p≤0.05), and when significant, they were submitted to Tukey test using the statistical software SISVAR ® (Ferreira, 2011).
For the maximum quantum yield of photosystem II (Fv/Fm), a significant effect (p ≤ 0.05) for the interaction between inoculant and maize hybrids was observed, however there was no significant effect of nitrogen. ŽivÄák et al. (2008) observed that Fv/Fm is insensitive to many factors that negatively affect plant photosynthesis.
Regardless of treatment, with and without inoculation, H1, ​​H2 and H3 maize hybrids showed no difference in values ​​of Fv/Fm ratio. For H4, it was observed that Fv/Fm was lower with inoculant when compared with plants without inoculation, a difference of approximately 11%, on average, was observed in value of Fv/ Fm ratio (Figure 1). Canelas et al. (2013) observed effect on promoting greater photosynthetic capacity of the plant of corn when inoculated with Herbaspirillum seropedicae.
With inoculant presence, Fv/Fm mean obtained in H1, ​​H2, H3 and H4 maize hybrids were 0.78, 0.79, 0.78 and 0.71, respectively. It was noted, therefore, that there were differences between H2 and H4 in Fv/Fm ratio. The highest value was recorded in H2 and the lowest value in H4. In plants with inoculation absence, however, there were no differences in Fv/Fm ratio among maize hybrids. The Fv/Fm mean obtained from these plants was 0.78 for H1, 0.77 for H2, 0.75 for H3 and 0.79 for H4 (Figure 2).
The Fv/Fm ratio is a sensitive indicator of plant photosynthetic performance, because it shows the functional state of photosynthetic apparatus (Björkman and Demming, 1987; Johnson et al., 1993). For most species, the values ​​of Fv/Fm ratio are between 0.75 and 0.85 when plants are not under stress conditions; however, values ​​lower than 0.75 would be indicative of plant exposure to stress, indicating occurrence of photoinhibition in photosynthetic apparatus (Ronquim et al., 2009).
In this study, from all evaluated maize hybrids, only H4 hybrid when inoculated with the bacteria, A. brasilense presented Fv/Fm values below 0.75. The other hybrids (H1, H2 and H3) maintained their ​​Fv/Fm values within normal, independent of inoculation, indicating that there was no reduction in photochemical efficiency of FSII. However, although H4 has presented decreases in Fv/Fm, there are some studies in literature describing as normal values until 0.71, especially in crops. Thus, it can be said that there was no damage in photosystem II of maize hybrids. Araújo et al. (2014) observed that the inoculation with the Z-94 strain of H. seropedicae promoted increase in root volume, root length, shoot dry mass, chlorophyll content, shoot N content and N use efficiency.
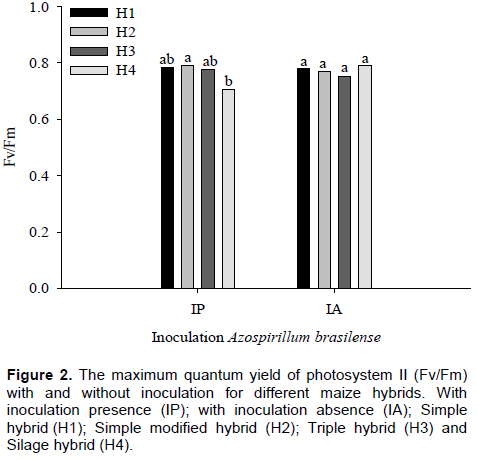
was significant for effective quantum efficiency variable of photosystem II (∆F/Fm’), according to F test, at 5%. In plants with inoculants, differences between nitrogen levels in the value of ∆F/Fm’ were observed. Similar responses were found in plants without inoculation (Figure 3). Apostol et al. (2008) observed that the effective PSII quantum yield computed as ΔF/Fm’ is fluorescence parameter that gives better results in discriminating between treatments with doses intermediate to nitrogen.
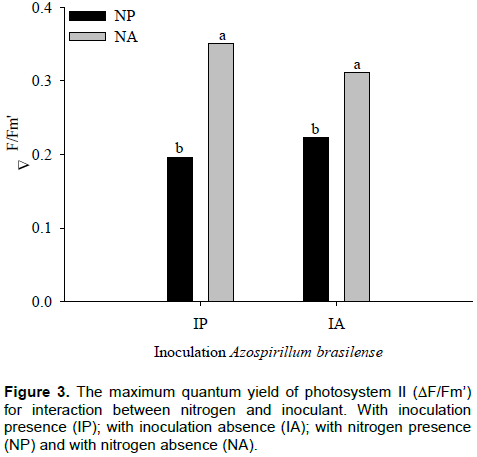
With inoculant, the highest and lowest value of ∆F/Fm’ was 0.35 and 0.20 in plants with nitrogen and without nitrogen, respectively. In plants without inoculant, the highest value was 0.31, with nitrogen, and the lowest value of 0.22 without nitrogen for ∆F/Fm’. An increase of 56% with inoculant, and 71% without inoculation in ∆F/Fm’ with nitrogen application is shown in Figure 3. In Figure 4, it is observed that, regardless of with or without nitrogen, the inoculation did not affect the ∆F/Fm’ in maize hybrids. The parameters derived from rapid and non-invasive measurements of the ChlF kinetics can serve for assessment of leaf photosynthetic performance influenced by different nitrogen nutrition, useful for crop research and practical applications (ŽivÄák et al., 2014).
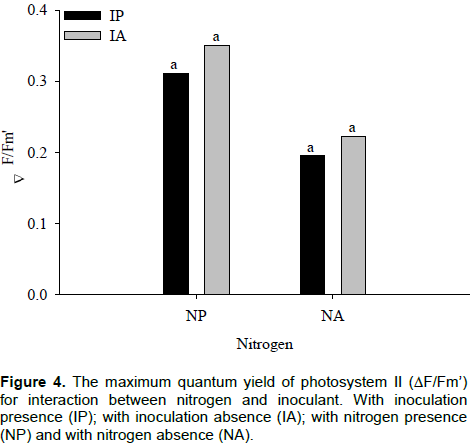
The ∆F/Fm’ is the most sensitive indicator of plant physiological state and usually, decrease in values of this characteristic ​​denotes stress condition. With nitrogen deficit, and independent of inoculation with A. brasiliense, plants showed a smaller ∆F/Fm’, thus, indicating a better physiological response of maize hybrids supplied with nitrogen. Nitrogen application provides to plants a better use of energy absorbed to photosynthetic processes. Kappes et al. (2013) observed that the application of N in coverage provided greater foliar chlorophyll content in maize plants.
Similar responses to ∆F/Fm’ were obtained in ETR, which increased with nitrogen application, confirming a higher CO2 assimilation rate as compared to plants with nitrogen deficit. It is noted, therefore, that in maize plants, absence of nitrogen caused substantial reduction in transport of electrons to carbon fixation. In contrast, H4 maize hybrid showed increase in ETR in plants with no inoculation with A. brasilense. Galindo et al. (2016) verified that nitrogen rates of up to 200 kg ha-1 improve grain yield for corn that has been inoculated with A. brasilense, regardless of the N source utilized.
Electron transport rate (ETR) was significantly affected by nitrogen (p ≤ 0.01) and interaction between inoculant and maize hybrids (p ≤ 0.05). For maize hybrids without and with nitrogen, average ETR values of 91 and 138 mmol m-2 s-1 were obtained, respectively. On average, there was a difference in ETR of about 34% between treatments. Therefore, with nitrogen, maize plants showed increase of 47 mmol m-2 s-1, which means increase of approximately 66% in ETR value (Figure 5).
Tang (2000), Yang et al. (2002), Duan et al. (2007) and Ji-rui et al. (2013) observed that the nitrogen fertilizer application had a great impact on the ETR and the EQY, increased the chlorophyll content of crop leaves, electron transport capacity of PS I and PS II, and extended the high-value duration of the photosynthetic rate in leaves.
Electron transport rate showed no significant difference between with and without inoculation for H1, H2 and H3, but when compared with inoculant effect in H4, there was an increase in ETR of about 67% in maize plants without inoculation (Figure 6).
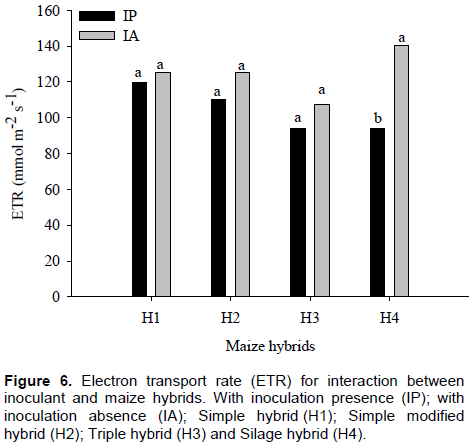
In plants with inoculants, there were no differences among maize hybrids for ETR values, which for H1, H2, H3 and H4 were 125.5, 125.1, 107.2 and 93.8 mmol m-2 s-1, respectively. However, without inoculation, it was found that H4 showed the highest mean ETR value (140 mol m-2 s-1) and H3, the lowest value (94 mmol m-2 s-1) (Figure 7). Carvalho et al. (2011) observed in six maize genotypes that the stress was recorded to limit photosynthetic machinery.
The interaction between nitrogen and maize hybrids for non-photochemical quenching (NPQ) was significant (p ≤ 0.01). By comparing nitrogen within each maize hybrid, it was found that only H3 showed no difference between NPQ values. However, in H1, H2 and H4 hybrids, differences of 33, 54 and 36%, respectively were observed in NPQ values, between plants with and without nitrogen. It must be noted that there was high NPQ values ​​obtained in maize hybrids with nitrogen absence (Figure 8). Souza et al. (2013) verified increase in the NPQ of maize genotypes to conserve their photosystems from adverse effects of stress.
In plants with nitrogen, there were no differences in NPQ values ​​among H4, H1, H2 and H3 maize hybrids with values of 0.41, 0.51, 0.65 and 0.84, respectively.
However, H1 and H3 maize hybrids with no nitrogen whose obtained values ​​were 1.57 and 0.90, respectively, showing a difference of approximately 57% on average, equivalent to a NPQ value of 0.67. The H2 and H4 maize hybrids do not differ from the others, in plants with no nitrogen, although it also showed higher NPQ values ​​(Figure 9). Kumagai et al. (2009) observed that the NPQ in the flag leaves of the six cultivars increased particularly in the nitrogen-deficient plants
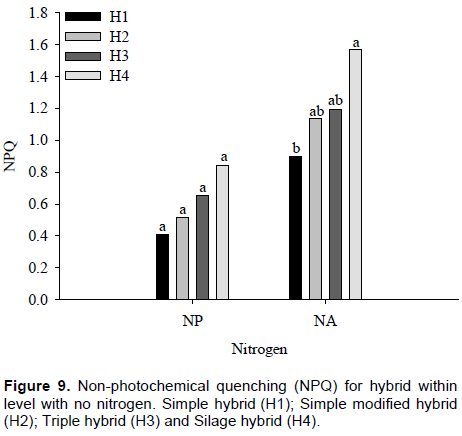
NPQ acts on excess energy dissipation absorbed by reaction centers through heat. Generally, normal NPQ values ​​ are between 0.3 and 0.7. This means that, in plants with nitrogen, NPQ values ​​remained as normal. In maize hybrids without nitrogen, NPQ results obtained in this study are indicative of the presence of some plant protection mechanism. Normally, when values ​​of ∆F/Fm’ and ETR are low, there is increase in NPQ in order to dissipate energy that is not being used in photochemical processes. Pandolfo et al. (2015) did not observe a positive effect from inoculation when they studied N rates in topdressing and A. brasilense inoculation in corn crops.
Good photosynthetic performance of maize hybrids inoculated with A. brasilense may be due to better efficiency in absorption and nitrogen utilization promoted by associated bacteria, probably to some plant damage control mechanism aiming at maintaining photosynthetic process by chlorophyll degradation reduction. When reviewing studies on physiological responses of plants induced by Azospirillum, Barassi et al. (2008) reported improvement in photosynthetic parameters, including chlorophyll content. Thus, A. brasilense inoculation showed great use potential in different maize hybrids, therefore contribute to nitrogen fertilizer saving, as well as provide a greater use of available resources and thus, more sustainable agriculture.
The silage hybrid, when inoculated with A. brasilense, showed values ​​of Fv/Fm below 0.75. The simple, simple modified and triple hybrids maintained its Fv/Fm values within normal and it can be stated that there was no damage on photosystem II of maize hybrids. The nitrogen application provided to plants better use energy absorbed to photosynthetic processes (∆F/Fm’).
Also, for maize hybrids without and with nitrogen, average ETR values ​​of 91 and 138 mol m-2 s-1, respectively, were obtained. Due to better efficiency in absorption and nitrogen utilization provided by bacteria (A. brasilense), inoculated maize hybrids showed good photosynthetic performance.
The authors have not declared any conflict of interests
The authors thank the Ministry of Science and Technology (MCT), the Foundation for Research Support of the State of Goiás (FAPEG), the Coordination for Upgrading Higher Institution Personnel (CAPES) and the Brazilian Council for Scientific and Technological Development (CNPq) for funding the current scientific project.
REFERENCES
Apostol SL, Ion RM, Tugulea L (2008). Spectral methods for nitrogen deficiency evaluation in maize plants. J. Optoelectronics Adv. Mater. 10(6):1478-1481.
|
|
Araújo ÉO, Mercante FM, Vitorino ACT, Paim LR (2014). Inoculation of Herbaspirillum seropedicae in three corn genotypes under different nitrogen levels. Afr. J. Agric. Res. 9(21):1628-1634.
Crossref
|
|
|
Barassi CA, Sueldo RJ, Creus CM, Carrozzi L, Casanovas EM, Pereyra MA (2008). Potencialidad de Azospirillum en optimizer el crecimiento vegetal bajo condiciones adversas. In: Cassán, F.D.; Garcia de Salamone, I. (Ed.) Azospirillum sp.: cell physiology, plant interactions and agronomic research in Argentina. Argentina: Asociación Argentina Microbiol. pp. 49-59.
|
|
|
Bartchechen A, Fiori CCL, Watanabe SH, Guarido RC (2010). Efeito da inoculação de Azospirillum brasilense na produtividade da cultura do milho (Zea mays L.) - Campo Digit@l, Campo Mourão 5(1):56-59.
|
|
|
Bergamaschi C (2006). Ocorrência de bactérias diazotróficas associadas às raízes e colmos de cultivares de sorgo. Dissertação (Mestrado em Microbiologia Agrícola) - Universidade Federal do Rio Grande do Sul, Porto Alegre pp. 14-83.
|
|
|
Bilger W, Björkman O (1990). Role of xanthophyll cycle in photoprotection elucidated by measurements of light induced absorbance changes, fluorescence and photosynthesis in leaves of Hedera canariensis. Photosyn. Res. 25(1):173-185.
Crossref
|
|
|
Bilger W, Schreiber U, Bock M (1995). Determination of the quantum efficiency of photosystem II and of non-photochemical quenching of chlorophyll fluorescence in the field. Oecologia 102(1):425-432.
Crossref
|
|
|
Björkman O, Demming B (1987). Photon yield of O2 evolution and chlorophyll fluorescence characteristics at 77 K among vascular plants of diverse origin. Planta, New York 170(1):489-504.
|
|
|
Camargo FAO, Sá ELS (2004). Nitrogênio e adubos nitrogenados. In: Bissani CA, Gianello C, Tedesco MJ, Camargo FAO. (Ed.) Fertilidade dos solos e manejo da adubação de culturas. Porto Alegre: Genesis pp. 93-116.
|
|
|
Canelas LP, Balmori DM, Médici LO, Aguiar NO, Campostrini E, Rosa RCC, Façanha AR, Olivares FL (2013). A combination of humic substances and Herbaspirillum seropedicae inoculation enhances the growth of maize (Zea mays L.). Plant Soil 366:119-132.
Crossref
|
|
|
Cantarella H (2007). Nitrogênio. In: Novais, R. F.; Alvarez, V. H.; Barros, N. F; Fontes, R. L.; Cantarutti, R. B.; Neves, J. C. L. (Ed.). Fertilidade do solo. Viçosa, MG: Soc. Bras. Ciênc. Solo pp. 375-470.
|
|
|
Carvalho RC, Cunha A, Silva, JM (2011). Photosynthesis by six Portuguese maize cultivars during drought stress and recovery. Acta Physiol. Plantarum 33:359-374.
Crossref
|
|
|
Conab (2013). Acompanhamento da safra brasileira: grãos. Companhia nacional de abastecimento. Décimo levantamento, Brasília: Conab pp. 2-28.
|
|
|
Duan WW, Zhao HM, Guo CJ, Xiao K, Li YM (2007). Responses of photosynthesis characteristics to nitrogen application rates in summer maize (Zea mays L.). Acta Agron. Sin. 33(6):949-954.
|
|
|
Ehleringer J (1981). Leaf absorptances of Mohave and Sonoran desert plants. Oecologia 102(1):366-370.
Crossref
|
|
|
Fancelli AL (2011). Fenologia, fisiologia da produção e implicações práticas de manejo. In: Milho: produção e produtividade, Piracicaba: USP/ESALQ/LPV. pp. 1-34.
|
|
|
Ferreira DF (2011). Sisvar: a computer statistical analysis system. Ciênc. Agrotecnol. 35(6):1039-1042.
|
|
|
Galindo FS, Teixeira Filho MCM, Buzetti S, Santini JMK, Alves CJ, Nogueira LM, Ludkiewicz MGZ, Andreotti M, Bellotte, JLM (2016). Corn Yield and Foliar Diagnosis Affected by Nitrogen Fertilization and Inoculation with Azospirillum brasilense. Rev. Bras. Ciênc. Solo 40(1):1-18.
Crossref
|
|
|
Genty B, Briantais JM, Baker NR (1989). The relationship between the quantum yield of photosynthetic electron transport and quenching of chlorophyll fluorescence. Biochim. Biophys. Acta 990(1):87-92.
Crossref
|
|
|
Gross MR, Von Pinho RG, Brito AH (2006). Adubação nitrogenada, densidade de semeadura e espaçamento entre fileiras na cultura do milho em sistema plantio direto. Ciênc. Agrotecnol. Lavras 30(3):387-393.
|
|
|
Hartmann A, Baldam JI (2006). The genus Azospirillum. In: Dworkin M, Falkow S, Rosenberg E, Schleifer KH, Stackebrandt E. (Ed.). The Prokaryotes. New York: Springer. pp. 115-140.
Crossref
|
|
|
|
Huergo LF, Monteiro RA, Bonatto AC, Rigo LU, Steffens MBR, Cruz LM, Chubatsu LS, Souza EM, Pedrosa FO (2008). Regulation of nitrogen fixation in Azospirillum brasilense. In: Cassán FD, Garcia de Salamone I. Azospirillum sp.: cell physiology, plant interactions and agronomic research in Argentina. Asociación Argentina de Microbiologia, Argentina pp. 17-35.
|
|
|
Hungria M, Araújo RS, James E (1994). Manual de métodos empregados em estudos de microbiologia agrícola. Brasília: Embrapa/SPI. pp. 20-542.
|
|
|
Ji-Rui L, Guo-Hui M, Yi-Zheng W, Chun-Fang S, Jian S, Rui-Jun Q (2013). Effects of Nitrogen Fertilizer Level on Chlorophyll Fluorescence Characteristics in Flag Leaf of Super Hybrid Rice at Late Growth Stage. Rice Sci. 20(3):220−228.
Crossref
|
|
|
Johnson GN, Young AJ, Scholes JD, Horton P (1993). The dissipation of excess excitation energy in British plant species. Plant Cell Environ. 16(1):673-679.
Crossref
|
|
|
Kappes C, Arf O, Arf MV, Ferreira JP, Dal Bem EA, Portugual JR, Vilela RG (2013). Inoculation of seeds with diazotrófica bacteria and nitrogen application on leaf and cover in corn. Semina Agric. Sci. 34(2):527-538.
|
|
|
Kumagai E, Araki T, Kubota F (2009). Characteristics of Gas Exchange and Chlorophyll Fluorescence during Senescence of Flag Leaf in Different Rice (Oryza sativa L.) Cultivars Grown under Nitrogen-Deficient Condition. Plant Prod. Sci. 12(3):285-292.
Crossref
|
|
|
Laisk A, Loreto F (1996). Determining photosynthetic parameters from leaf CO2 exchange and chlorophyll fluorescence. Plant Physiol. 110(3):903-912.
|
|
|
Menezes MJT (2004). Eficiência agronômica de fontes nitrogenadas e de associações de fertilizantes no processo de diferimento de Brachiaria brizanta cv. Marandu. Dissertação (Mestrado) - Escola Superior de Agricultura Luiz de Queiroz, Universidade de São Paulo, Piracicaba pp. 20-113.
|
|
|
Pandolfo CM, Vogt GA, Balbinot Júnior AA, Gallotti GJM, Zoldan SR (2015). Desempenho de milho inoculado com Azospirillum brasilense associado a doses de nitrogênio em cobertura. Agropecu. Catarin 27:94-9.
|
|
|
Queiroz AM, Souza CHE, Machado VJ, Lana RMQ, Korndorfer GH, Silva AA (2011). Avaliação de diferentes fontes e doses de nitrogênio na adubação da cultura do milho (Zea mays L.). Rev. Bras. Milho Sorgo 10(3):257-266.
Crossref
|
|
|
Rascher U, Liebig M, Luttge U (2000). Evaluation of instant light-response curves of chlorophyll fluorescence parameters obtained with a portable chlorophyll fluorometer on site in the field. Plant Cell Environ. 23(1):1397-1405.
Crossref
|
|
|
Repke RA, Cruz SJS, Silva CJ, Figueiredo PG, Bicudo SJ (2013). Eficiência da Azospirillum brasilense combinada com doses de nitrogênio no desenvolvimento de plantas de milho. Rev. Bras. Milho Sorgo 12(3):214-226.
Crossref
|
|
|
Ronquim CC, Prado CHBA, Souza JP (2009). Growth, photosynthesis and leaf water potential in young plants of Capaifera langsdorffii Desf. (Caesalpiniaceae) under contrasting irradiances. Braz. Soc. Plant Physiol. 21(3):197-208.
|
|
|
Saikia SP, Jain V, Khetarpal S, Aravind S (2007). Dinitrogen fixation activity of Azospirillum brasilense in maize (Zea mays). Curr. Sci. 93:9.
|
|
|
Sarruge JR (1975). Soluções nutritivas. Summa Phytopathol. Bot. 1(3):231-233.
|
|
|
Setiyono TD, Walters DT, Cassman KG, Witt C, Dobermann A (2010). Estimating maize nutrient uptake requirements. Field Crops Res. Amsterdam 118(1):158-168.
Crossref
|
|
|
Shioga OS, Oliveira EL, Gerage AC (2004). Densidade de plantas e adubação nitrogenada em milho cultivado na safrinha. Rev. Bras. Milho Sorgo Sete Lagoas 3(1):381-390.
Crossref
|
|
|
Souza TC, Magalhaes PC, Castro EM, Albuquerque PEP, Marabesi MA (2013). The influence of ABA on water relation, photosynthesis parameters, and chlorophyll fluorescence under drought conditions in two maize hybrids with contrasting drought resistance. Acta Physiol. Plantarum 35(1):515-527.
Crossref
|
|
|
Tang XR (2000). Effect of N supply on yield and protein content and its mechanism in fodder hybrid rice. Hybrid Rice 15(2):34-37.
|
|
|
van Kooten O, Snel JFH (1990). The use of chlorophyll fluorescence nomenclature in plant stress physiology. Photosyn. Res. 25(1):147-150.
Crossref
|
|
|
Vincent JM (1970). A manual for the practical study of root nodule bactéria. Oxford: Blackwell Sci. pp. 100-164.
|
|
|
Yang Q, Li YM, Xiao K, Dou YH (2002). Effect of different amount of nitrogen on flag leaf senescence and yield components of wheat. J. Hebei Agric. Univ. 25(4):20-24.
|
|
|
ŽivÄák M, BrestiÄ M, Olšovská K, Slamka P (2008). Performance index as a sensitive indicator of water stress in Triticum aestivum L. Plant Soil Environ. 54:133-139.
|
|
|
ŽivÄák M, Olšovská, K, Slamka P, Galambošová J, Rataj V, Shao HB, BrestiÄ M (2014). Application of chlorophyll fluorescence performance indices to assess the wheat photosynthetic functions influenced by nitrogen deficiency. Plant Soil Environ. 60(5):10-215.
|
|