ABSTRACT
Microbial biotransformation of coumarin (I) was undertaken using a battery of twenty five microorganisms. Among them, the fungus Cunninghamella elegans NRRL 1392 was the sole microbe that efficiently metabolized coumarin (I) into umbelliferone (II), 3, 4-dihydrocoumarin (V) and transcinnamic acid (VI). Umbelliferone was also biotransformed into 6, 7-dihydroxycoumarin (aesculetin, VII) and p-coumaric acid (VIII). On the other hand, warfarin (III) was biotransformed into 4`-hydroxywarfarin (IX) and also dicoumarol (IV) was transformed into 4-hydroxycoumarin (X) by the same microorganism. The structures of the metabolites were established using only one property and spectroscopic techniques including melting points, 1H NMR, 13C NMR and mass spectroscopy. The cytotoxic effect of coumarin substrates and their metabolites was also investigated.
Key words: Biotransformation, coumarins, Cunninghamella elegans, cytotoxic.
Coumarin ring system (benzo-α-pyrone) (Dean, 1963) is widely encountered in natural products in many plant families, such as Umbellifereae, Rutaceae and others (Murray et al., 1982; Berenbaum, 1991). Coumarin (I) is used extensively as perfume constituent (Trumble et al., 1990), while its derivatives umbelliferone (II), warfarin (III) and dicoumarol (IV) may exhibit activity against several types of animal tumors (Beier and Nigg, 1994). Furthermore, umbelliferone (II) demonstrated an antioxidant activity (Beier and Oerti, 1983), while dicoumarol (IV) and warfarin (III) possessed a potential antineoplastic (Jamal and Casley Smith, 1989) as well as anticoagulant (Musajo and Rodighiero, 1962) effects, respectively. The use of microbial transformation in simulation of mammalian drug metabolism was firstly reported by Smith and Rosazza (1975) to insight the drug metabolic pathway, mechanism of action and toxicity. These studies were based on the parallels, which exist between phase-1 and phase-2 reactions in both microbial and mammalian systems (Van Scott, 1976; Scott et al., 1976) and had led in overcoming several complications of low yield, high coast and impure metabolites appeared during the studies of drug mammalian metabolism. Recently, this approach is used in the synthesis of new biologically active revelant products (Wackett et al., 1995).
Bioconversion of some coumarins derivatives into new therapeutic products using several microorganisms was recently reported by Nigam et al. (2013). The present study is focused on the application of microorganisms as models to study and predict fate of the investigated coumarins in mammalian systems.
Substrates and microorganisms
Substrates were obtained from the following sources: coumarin (I) was obtained from Cairo Company (Egypt). Warfarin (III) and dicoumarol (IV) were purchased from Sigma Chemical Company Inc. St. Lewis, Mo., while umbelliferone (II) was purchased from Fluka Chemical Company Inc.; Milwaukee, Uli. Sabouraud dextrose agar was obtained from Oxoid Ltd., England. Microorganisms were obtained from American Type Culture collection (ATCC, Rockville, Maryland) or from Northern Regional Research Laboratories (NRRL, Peoria, Illinois) (Siegers and Bostelmann, 1993). A list of microorganisms is shown in Table 1.
Microbial biotransformation protocol
Preparation of the culture medium
The fermentation experiments were performed using a medium consisting of 5 g peptone, 10 g dextrose, 10 ml glycerol, 5 g yeast extract, 5 g NaCl, 5 g K2HPO4 and volume adjusted to 1000 ml with distilled water. The pH was adjusted to 6.0 before sterilization at 121°C for 15 min (Myers et al., 1994).
Cultivation of microorganisms
The cultures were initiated to grow from lyophilized state by adding 1 ml of sterile water to microorganism vials. Few drops of suspended cells were streaked on Sabouraud-Dextrose plates to check the purity. The pure cultures were maintained on slants of either Potato-Dextrose agar or Sabouraud-Dextrose agar. The fresh slants were incubated for few days at room temperature before storage in a refrigerator at (4°C). The fermentation liquid cultures were initiated by transferring a few drops of suspended cells or a loopful into 25 ml sterile liquid medium present in 125 ml Erlenmeyer flask then placing on a gyratory shaker operating at 250 rpm and 27°C.The temperature was maintained at 27°C (Myers et al., 1994).
Biotransformation by C. elegans NRRL 1392
Fermentation of coumarin (I) was investigated using a battery of twenty-five microorganisms listed in Table 1. Stage one culture of each investigated microorganism was initiated separately from two-week old slants (under aseptic conditions) by transferring microbial cells into 250 ml Erlenmeyer flasks, containing 50 ml of sterile liquid medium and allowed to grow for 72 h at 27°C on a gyratory shaker. Stage two cultures were obtained by transferring 5 ml of stage one culture to each of 250 ml flasks containing 50 ml of fresh liquid medium. Both substrate and organism controls were treated in the same way. The culture was allowed to grow for 24 h, before the addition of the substrate solution (0.2 mg coumarin (I) ml -1 dissolved in acetone). Samples (5 ml) were periodically withdrawn from each culture and extracted successively with chloroform, then with ethyl acetate. Each extract was separately evaporated to dryness under reduced pressure and kept for further analysis. The obtained residues were dissolved in few drops of a suitable organic solvent and chromatographed on silica gel G plates (E. Merck , F 254) using solvent system i chloroform- methanol (9.5:0.5, v/v), solvent system ii: chloroform-methanol (9:1), solvent system iii: chloroform-ethyl acetate-formic acid (4:1:0.5, v/v), solvent system iv: toluene-ethyl acetate (2:1, v/v). The plates were dried and visualized under UV light at 254 and 366 nm before and after spraying with alcoholic KOH (Feuer et al., 1976) and anisaldehyde/sulfuric reagents (Thornes et al., 1989). The results of the initial screening and the extent of biotransformation are shown in Table 1.
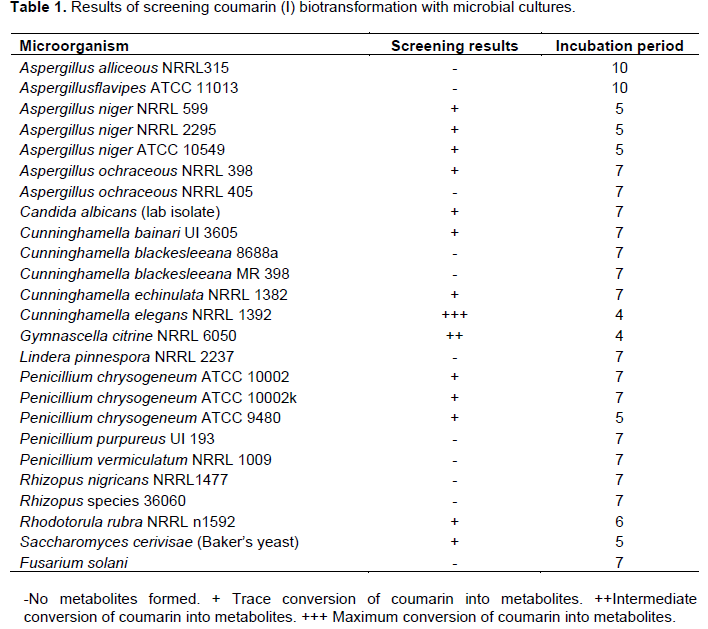
The same procedure was used in large scale fermentation production of each investigated coumarins into different metabolites using C. elegans NRRL 1392 only (Siegers and Bostelmann, 1993).
Isolation, purification and identification of metabolites
The metabolites were isolated from fermentation residues by different chromatographic methods. The residues were crystallized, separately, using the appropriate solvent. The identification of the isolated pure compounds was achieved using "Galenkamp type" melting point apparatus and different spectroscopic methods. Thermo-Finnegan mass spectrometer and API 3200TM LC/MS/MS were used. Both 1H and 13C NMR spectra were taken on JEOL JNM-ECA NMR spectrometer operating at 500 MHz (1H NMR) and 125 MHz (13C NMR), respectively. The chemical shift values were reported as ppm using tetramethylsilane (TMS) as an internal standard and coupling constants were expressed in Hz. Negative mode electrospray ionization mass spectra.
Large scale fermentation of coumarin (I) by C. elegans NRRL 1392 and isolation of metabolites
Coumarin (I, 1 g of standardd coumarin) dissolved in acetone (25 ml), was equally distributed amongst 50 to 500 ml-flasks containing stage two cultures of C. elegans as mentioned previously. After 4 days, the fermentation was stopped and the cells were removed by filtration.
The fermentation broth was extracted twice with an equal volume of chloroform and the solvent was distilled off under reduced pressure to give 500 mg residue. The obtained residue was chromatographed on silica gel column (20 g, 25 cm L × 1 cm D) using gradient elution method starting with hexane, hexane-chloroform, chloroform then chloroform-methanol with increasing proportions of methanol till 15%. Seventy two fractions (10 ml each) were collected. The fractions (13 to 16) eluted with hexane-chloroform (7:3) were combined, evaporated till dryness to give a pure metabolite (V) (6 mg, in yield of 0.6%). Metabolite (V) was soluble in chloroform and ether. It was subjected to GC/MS analysis as the result was represented by Figure 1.
However, fractions (25 to 33) eluted with chloroform-methanol (99:1, v/v) provided 213 mg of pure metabolite (II) in 21% yield. Metabolite (II) was co-chromatographed with an authentic umbelliferone for identification.
Fractions (41 to 50) eluted with chloroform-methanol (95:5, v/v) were pooled and evaporated to a residue, which was recrystalized from methanol to give a white monoclinic crystals of metabolite (VI) (87 mg), in 9% yield. The 1H and13C NMR data of metabolite (VI) was shown in Table 4.
Large scale fermentation of umbelliferone (II) by C. elegans NRRL 1392
One gram of standard umbelliferone was dissolved in acetone (25 ml) and equally distributed amongst 50 to 500 ml flasks containing stage two cultures of C. elegans as mentioned previously. After 5 days, the fermentation was stopped and the cells were removed by filtration. Fermentation broth was extracted twice with an equal volume of chloroform and the solvent was distilled off under reduced pressure to give 510 mg residue. The residue was chromatographed on silica gel column (22 g, 25 cm L and 1 cm D) and eluted with chloroform, chloroform-ethyl acetate in gradient elution manner till pure ethyl acetate. Fractions eluted with CHCl3-EtOAc (7.5:2.5, v/v) were evaporated under reduced pressure till dryness and recrystallized from methanol to give 125 mg of metabolite (VII) in 12.5% yield. This metabolite was subjected to1H and 13C NMR analysis as the resultant data are shown in Table 2.
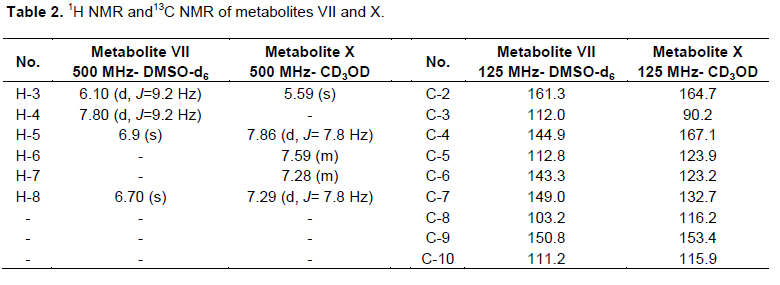
Fractions eluted with CHCl3-EtOAc (1:1, v/v) were pooled and evaporated to dryness to give 180 mg residue. This residue was further rechromatographed on Sephadex LH20 column (8 g, 10 cm L × 0.5 cm D) and eluted with methanol. Thirty fractions (2 ml each) were collected. Fractions (10 to 30) gave 100 mg, a white powder of pure metabolite (VIII) in 10% yield. This metabolite possessed 1H and 13C NMR analysis, where the data are shown in Table 4.
Large scale fermentation of warfarin(III) by C. elegans NRRL 1392
One gram of standard warfarin was dissolved in acetone (25 ml) and equally distributed amongst 50 to 500 ml flasks containing stage two cultures of C. elegans as mentioned previously. After 7 days, the fermentation was stopped and the cells were removed by filtration. The fermentation broth was extracted twice with an equal volume of dichloromethane and the solvent was removed under reduced pressure to give 400 mg residue. The metabolites were separated from this residue by preparative thin layer chromatography (TLC) on silica gel G254 plates using solvent system iv as a mobile phase. Zone no. 2 was separated and extracted by ethyl acetate, the solvent evaporated to produce one compound with an Rf = 0.23 in solvent system iv. Metabolite (IX) was produced and purified by recrystalization from ethyl acetate giving 19 mg pure compound. Its 1H and 13C NMR spectral analysis was illustrated in Table 3.
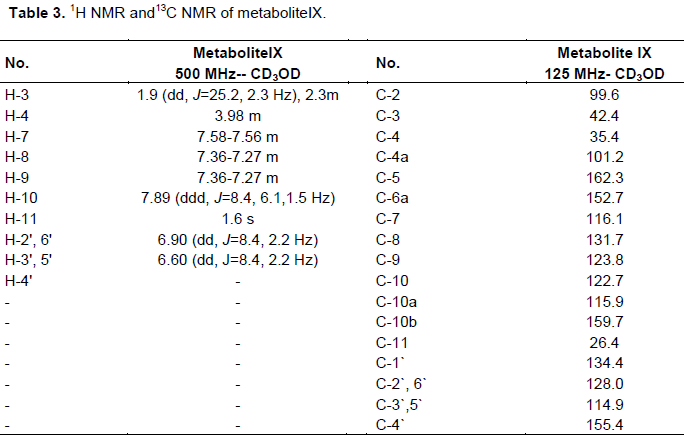
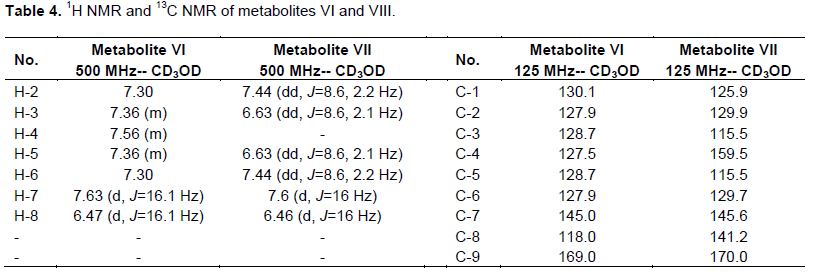
Large scale fermentation of dicoumarol (IV) by C. elegans NRRL 1392
One gram of standard dicoumarol was dissolved in acetone (25 ml) and equally distributed amongst 50 to 500 ml-flasks containing stage II cultures of C. elegans as mentioned previously. After 8 days, the fermentation was stopped and the cells were removed by filtration. The fermentation broth was extracted twice with an equal volume of n-butanol. The extract was evaporated under reduced pressure to give 500 mg residue, which was chromatographed twice on Sephadex LH20 column (25 g, 25 cm × 1 cm), eluted with methanol.
One hundred and twenty fractions (5 ml each) were collected. Fractions (86 to 120) showed one spot. The solvent was removed under reduced pressure and the residue was recrystalized from methanol giving 32 mg of metabolite (X), in 3.2% yield. The obtained pure metabolite undergoes 1H and 13C NMR analysis as the data shown in Table 5
Cytotoxic investigation of the examined coumarins and the resultant metabolities
Potential cytotoxicity of coumarins and the isolated pure metabolites was tested using SRB assay against HEPG2 tumor cell line (Shehan et al., 1990) as follows:
(1) Cells were plated in 96-multiwell plate (104 cells/well) for 24 h before treatment with the compounds to allow attachment of cells to the bottom of the plate.
(2) Different concentrations of each compound under test (1, 2.5, 5 and 10 µg/ml) added to the medium containing the monolayer cell in triplicate wells were prepared for each individual dose.
(3) The wells were incubated with the compound(s) for 48 h at 37°C in an atmosphere of 5% CO2 (using CO2- incubator).
(4) The medium was discarded and the cells were fixed with formalin after 48 h, washed and stained with sulforhodamine B stain.
(5) Excess stain was washed with acetic acid and attached stain was recovered with tris EDTA buffer.
(6) Color intensity was measured in an ELISA reader.
(7) The relation between surviving fraction of the cells and drug concentration is plotted to get the survival curve of each tumor cell line after treatment with the specified compound.
Microbial transformation screening experiments were performed on coumarin (I) using twenty five different species of microorganisms and culminated in the detection of several metabolites. The results (Table 1) illustrated that C. elegans NRRL 1392 was the best microorganism capable of performing maximum conversion of coumarin at the least incubation period into metabolites followed by Gymnascella citrina NRRL 6050 and to lesser or negative extent for the other investigated microorganisms. The previous studies showed that fungus C. elegans can mimic biotransformation reactions of aromatic compounds performed by mammals (Dhalwal et al., 2008). Therefore, the filamentous fungus C. elegans was selected as the main microbe in the remaining biotransformation experiments.
C. elegans metabolized coumarin (I) into the corresponding metabolites (II), (VI) and (V). The metabolite (II) was obtained as white powders soluble in ethyl acetate and methanol, which had a blue fluorescence in UV light and violet fluorescence when sprayed with alcoholic KOH reagent. It exhibited Rf=0.37 in solvent system I, which was identical to an authentic sample of umbelliferone (Steck and Bailey, 1969). This finding was confirmed by the ESIMS analysis, which showed [M-1] + peak at m/z 161 beside a melting point of 223 to 224°C and m.p. = 223 to 224°C. Metabolite (II) was identified as umbelliferone.
Pure metabolite (V) was revealed as colorless crystals soluble in chloroform and ether, with a Rf = 0.8 in solvent system i; m.p. = 24 to 25°C. It displayed a dark brown fluorescence under ultra violet light and showed no color when sprayed with anisaldehyde/sulfuric acid reagent. Metabolite (v) was subjected to GC/MS analysis as the resultant data is similar to the Liberian data of the authentic sample of 3, 4-dihydrocoumarin represented by Figure 1. Metabolite (V) was identified as 3, 4-dihydrocoumarin.
White monoclinic crystals of metabolite (VI), which is soluble in methanol displayed Rf values of 0.25 and 0.61 in solvent systems i and ii, respectively. It exhibited a dark brown color when sprayed with alcoholic KOH, no color when sprayed with anisaldehyde/sulfuric acid reagent. Metabolite (VI) has a m.p. =133°C and was subjected to ESIMS analysis obtaining a quassi molecular ion at (m/z): 149 [M+1]+. The 1H and 13C NMR data of metabolite (VI) represented in Table 4 were in concordance with the data reported for reference transcinnamic acid (Kalinowska et al., 2007; EL-Askary et al., 2008), so metabolite (VI) was identified as trans cinnamic acid.
Umbelliferone (II) was metabolized by C. elegans into metabolites (VII) and (VIII). Metabolite (VII) was obtained as yellowish green powder, which exhibited Rf values of 0.17 and 0.53 in solvent systems ii and iii, respectively. It showed a dark brown fluorescence in UV light and no color when sprayed with anisaldehyde/sulfuric acid reagent. 1H and 13C NMR data of metabolite (VII) are shown in Table 2, which lacked the H-6, H-7 signals resonating at δH 7.19, 7.45 in coumarin (Perel`son et al., 1970). Furthermore, H-5 signal for this metabolite underwent an upfield shift of 0.51 ppm, which is consistent with the ortho effect of introduced hydroxyl group (Sothers, 1972) at C-6. Examination of 13C NMR spectral data of the metabolite (VII) (Table 2) in comparison with those of coumarin (Kumari et al., 2004) confirmed the hydroxylation at positions attached to C-6 and C-7 as the ipso carbons exhibited a downfield shift of 20 ppm, 17.3 ppm. However, C-5 (ortho carbon) experienced an upfield shift of 15 ppm and C-8 (orthocarbon) showed a upfield shift of 13.5 ppm. All the aforementioned data were identical to data reported for a esculetin (VII) (Steck and Bailey, 1969). Thus, metabolites (VII) was identified as 6,7-dihydroxycoumarin (aesculetin).
However, metabolite (VIII) is a green powder soluble in methanol and ethyl acetate, possessed Rf values of 0.28 and 0.46 in solvent systems i and ii, respectively. It has a violet fluorescence in UV light and pink color when sprayed with anisaldehyde/sulfuric acid reagent and a melting point at 209 to 211°C. The 1H NMR and 13C NMR of this metabolites (Table 4) was identical to the corresponding data of p-coumaric acid (EL-Askary et al., 2008), which was structurally elucidated as p-coumaric acid.
Warfarin (III) was biotransformed by the same microorganism into metabolite (IX), a small needle crystal soluble in methanol and ethyl acetate. It exhibited a faint brown fluorescence under UV, a faint green color with KOH and with a m.p. = 200°C. 1H and 13C NMR data of metabolite IX are shown in Tables 3, exhibiting two aromatic doublet of doublet signals resonating at dH 6.60, 6.90 ppm (J=8.4, 2.2 Hz), assigned for protons H-3',5' and H-2',6', respectively, which indicated a 1,4-disubstituted benzene ring (Rizzo and Davis, 1989), compared with warfarin (Gebauer, 2007). On the other hand, 13C NMR of this metabolite (Table 3) compared to those of warfarin (Pisklak et al., 2003) showed C-4̀̀ signal strongly deshielded, while the C-3', C-5' signal is shifted upfield by 10 to 12.1 ppm. Furthermore, C-2', C-6' signal underwent a slight upfield shift of about 0.20 ppm. These results are in close agreement with substituent effect of a hydroxyl group on aromatic ring carbons (Sothers, 1972) and support the identification of the metabolite IX as 4'-hydroxy warfarin. C. elegans hydroxylation of warfarin (III) at 4' position was demonstrated to proceed via an arene oxide intermediate and displayed NIH shift (Pisklak et al., 2003).
Dicoumarol (IV) undergoes a specific metabolic reaction by C. elegans producing metabolite X as yellowish green powder, which possessed Rf value of 0.35 using solvent system ii with a dark brown fluorescence under UV light and faint green color when sprayed with anisaldehyde/sulfuric acid reagent. The metabolite was soluble in methanol and hot water. The interpretation of 1H data of metabolite X (Table 2) showed the absence of H-4 signals resonating at δH 7.76 ppm, which is consistent with the ortho effect of introduced hydroxyl group (Sothers, 1972) at C-67.45 and 7.76 ppm in coumarin (Perel`son et al., 1970). H-3 for metabolite X revealed an upfield shift of 0.85 ppm. 13C NMR spectral data of this metabolite illustrated that C-4 exhibited a downfield shift of 26.3 ppm and C-3 (ortho carbon) showed an upfield shift of 26.3 ppm in comparison with that of coumarin (Kumari et al., 2004) confirmed the hydroxylation of C-4 position. All the aforementioned data were identical to data reported for 4-hydroxycoumarin (X) (Traven et al., 1997), thus metabolites X was identified as 4-hydroxycoumarin.
The course of studying the metabolism of the investigated coumarins by C. elegans illustrated that several reaction was attempted. It hydroxylated coumarin (I), umbelliferone (II) and warfarin (III) into the corresponding hydroxyl derivatives, such as umbelliferone (II), 6, 7-dihydroxycoumarin (VII) and 4`-hydroxywarfarin (IX), respectively (Figure 2). Aromatic hydroxylation of coumarin (I) and umbelliferone (II) at positions 7, 6 by C. elegans has also a parallel in the mammalian systems (Lake, 1999; Shimada et al., 1996).
Other reduction reaction of coumarin (I) by C. elegans was attempted producing 3, 4-dihydrocoumarin (V), which was confirmed by GC-MS analysis. Formation of 3, 4-dihydroderivative takes place in coumarin (I) only, which indicated that the enzyme may have narrow substrate specificity.
Two main polar transcinnamic acid (VI) and p-coumaric acid (VIII) were obtained by a cleavage reaction (opening of the lactone ring of the heterocyclic ring system) of coumarin (I) and umbelliferone (II), respectively. This reaction is limited for coumarin (I) and umbelliferone (II), suggesting its (narrow) substrate specificity as the exact mechanism of this reaction needs further investigation.
However, C. elegans converted dicoumarol (IV) by a specific metabolic reaction as cleavage of methylene bridge into 4-hydroxycoumarin(X), in a mechanism which awaits further investigation.
Coumarins and related derivatives are widely used in the treatment of many diseases. Thus before ending this investigation, an assay was conducted on coumarin substrates under investigation, as well as the isolated pure metabolites for their cytotoxic activity (Table 5). The cytotoxicity of all coumarins was tested against HEPG2 tumor cell line adopting SRB assay (Maucher and Von Angerar, 1994) and IC50 determination and compared to that of a standard doxorubicin. All tested coumarins exhibited much higher IC50 (low cytotoxic effect) compared to doxorubicin except for 3, 4-dihydrocoumarin, warfarin and 6, 7-dihydroxycoumarin and further studies against other cell lines are worthwhile. All biological studies revealed that the investigated coumarins were metabolized into products, which were far less active, except for 6, 7-dihydroxycoumarin (VII) and 3, 4-dihydrocoumarin (V).
The fungus C. elegans NRRL 1392 metabolized coumarin (I) into umbelliferone (II), 3, 4-dihydrocoumarin (V) and trans cinnamic acid (VI). Umbelliferone was also biotransformed into 6, 7-dihydroxycoumarin (VII) and p-coumaric acid (VIII). On the other hand, warfarin (III) was biotransformed into 4`-hydroxywarfarin (IX) and also dicoumarol (IV) was transformed into 4-hydroxycoumarin (X) by the same microorganism. The cytotoxic effect of coumarin substrates and their metabolites was investigated revealing that both 6, 7-dihydroxycoumarin (VII) and 3, 4-dihydrocoumarin (V) were more active than a standard doxorubicin (positive control).
The authors have not declared any conflict of interest.
REFERENCES
Beier RC, Nigg HN (1994). Toxicity of naturally occurring chemicals in food. Disease Handbook, Diseasescaused by hazardous substances. Eds.: Hui YH, Gorham JR, Murrell KD, Cliver DO, New York, pp: Marcel Dekker, New York: Marcel Dekker 3:1-186.
|
|
Beier RC, Oerti EH (1983). Psoralen and other linear furanocoumarins asphytoalexins in celary. Phytochemistry 22:2595–2597.
Crossref
|
|
|
Berenbaum MR (1991). Coumarins in herbivores. Their Interactions with secondary plant metabolites. Eds. Rosenthal G, Berenbaum MR. New York 37:163-179.
|
|
|
Dean FM (1963). Naturally occurring oxygen ring compounds, 1sted. London, Butter worth.
|
|
|
Dhalwa Kl, Shinde VM, Namdeo AG, Mahadik KR (2008). Antioxidant profile and HPTLC-densitometric analysis of umbelliferone and psoralen in Aeglemarmelos. Pharm. Biol. 46(4):266- 272.
Crossref
|
|
|
EL-Askary HI, Abou-Hussein DR, Gadelrab LN, Nassar NN (2008). Phytochemical and biological investigation of the ethyl acetate fraction of Cymbopogon Proximus Stapf. (Halfabar) herb. Egypt. J. Biomed. Sci. 26:149-157.
|
|
|
Feuer G, Kellen JA, Kovacs K (1976). Suppression of 7,12-dimethylbenz(α) anthracene-induced breast carcinoma by coumarin intherat. Oncology 33:35-39.
Crossref
|
|
|
Gebauer M (2007). Synthesis and structure-activity relationships of novel warfarin derivatives. Bioorg. Med. Chem. 15(6):2414-2420.
Crossref
|
|
|
Jamal S, Casley Smith JR (1989). The effects of 5,6 benzo-[a]-pyrone (coumarin) and DEC on filariticlymphoedema and elephantiasis in India. Preliminary results. India Ann. Trop. Med. Parasitol. 83:287-290.
|
|
|
Kumari GN, Ganesh MR, Anitha R, Aravind S (2004). Complete reduction of 2H-pyran-2-one moiety of coumarin and 6-methyl coumarin by Colletotrichumcapsici. Z. Naturforsch C 59(5-6):405-407.
|
|
|
Kalinowska M, Swislocka R, Lewandowski W (2007). The spectroscopic (FT-IR, FT-Raman and 1H, 13C NMR) and theoretical studies of cinnamic acid and alkali metal cinnamates. Mol. Struct. 834:572-580.
Crossref
|
|
|
Lake BG (1999). Coumarin metabolism, toxicity and carcinogenicity: relevance for human risk assessment. Food Chem. Toxicol. 37:423-453.
Crossref
|
|
|
Maucher A, Von Angerar E (1994). Antitumour activity of coumarin and 7-hydroxycoumarin against 7,12-dimethylbenz[a ]anthracene-induced rat mammary carcinomas. Cancer Res. Clin. Oncol. 120(8):502-504.
Crossref
|
|
|
Musajo L, Rodighiero G (1962). The skin photosensitizing furocoumarins. Expreientia 18:153-161.
Crossref
|
|
|
Murray RDH, Mendez J, Brown SA (1982). The natural coumarins occurrence, chemistry and biochemistry. Eds.: Chichester, John Wiley & Sons. Bristol. pp. 67-92.
|
|
|
Myers RB, Parker M, Grizzle WE (1994). The effects of coumarin and suramin on the growth of malignant renal and prostatic cell lines. J. Cancer Res. Clin. Oncol. 120 (Suppl. No.1):S11-S13. Perel`son ME, Sheinker Y, Syrova GP, Turchin KF (1970). NMR spectra of natural coumarin derivatives. Khimiya Prirodnykh Soedinenii 6(1):6-14.
|
|
|
Pisklak M, Maciejewska D, Herold F, WawerI (2003). Solid state structure of coumarin anticoagulants: warfarin and sintrom.13C CPMAS NMR and GIAO DFT calculations. Mol. Struture 649:169-176.
Crossref
|
|
|
Rizzo JD, Davis PJ (1989). Microbial models of mammalian metabolism: Conversion of warfarin to 4′-hydroxywarfarin using Cunninghamellabainieri. Pharm. Sci. 78:183-189.
Crossref
|
|
|
Smith RV, Rosazza JP (1975). Microbial model of mammalin metabolism. J. Pharm. Sci. 64:1737-1759.
Crossref
|
|
|
Scott BR, Pathak MA, Mohn GR (1976). Molecular and genetic basis of furocoumarin reactions. Mutat. Res. 39:29-74.
Crossref
|
|
|
Sothers JB (1972). Carbon-13 NMR Spectroscopy, Eds. Blomquist and Wasserman, Academic Press, New York and Londo.
|
|
|
Shimada T, Yamazaki H, Guengerich FP (1996). Ethnic-related differences in coumarin 7-hydroxylation activities catalyzed by cytochrome P4502A6 in liver microsomes of Japanese and Caucasian populations. Xenobio. 26:395-403.
Crossref
|
|
|
Steck W, Bailey BK (1969), Characterization of plant coumarins by combined gas chromatography,ultraviolet absorption spectroscopy, and nuclear magnetic resonance analysis1. Can. J. Chem. 47(19):3577-3383.
Crossref
|
|
|
Siegers CP, Bostelmann HC (1993). Effect of coumarin on cell cycle proliferationin human tumor cell lines. Irish Coll. Phys. Surg. 22:47-50.
|
|
|
Thornes D, Daly I, Lynch G (1989). Prevention of early recurrence of high risk malignant melanoma by coumarin. Irish Melanoma Grou{p}. Eur. J. Surg. Oncol. 15(5):431-435.
|
|
|
Trumble JT, Dercks W, Quiros CF, Beier RC (1990). Host plant resistance and linear furanocoumarincontent of Apium accessions. J. Econ. Entomol. 83:519-525.
Crossref
|
|
|
Traven VF, Negrebetksy VV, Vorobjeva LI (1997). Keto-Enol tautomerism, NMR Spectra, and HD Exchange of 4- Hydroxycoumarins. Can. J. Chem. 75(4):377-383.
Crossref
|
|
|
Van Scott EJ (1976). Therapy of psoriasis. J. Am. Med. Assoc. 235:197-198.
Crossref
|
|
|
Wackett LP, Hershberger CD (1995). Biocatalysis and biodegradation microbial transformation of organic compounds. Eds.: Marcel Dekker, Inc., New York, USA. pp. 22-24.
|
|