ABSTRACT
The present study investigated the in- vivo and vitro bioactivities of the essential oil of Duguetia lanceolata branches (EODL) and caryophyllene oxide (CO). The chemical composition of EODL was analyzed by GC and GC/MS. Acetic acid-induced writhing, formalin, hot plate and tail-immersion methods were used to evaluate the antinociceptive effect in mice. Anti-inflammatory activity was tested by carrageenan-induced paw edema and pleurisy in rats, and in mouse models of acute ear inflammation induced by croton oil and arachidonic acid. 2,2-Diphenyl-1-picrylhydrazyl (DPPH), reducing power and ð›½-carotene bleaching assays were used to determine the antioxidant bioactivity. ï¢-Elemene, caryophyllene oxide and ï¢-eudesmol were the major components found in EODL. When tested, EODL and CO reduced the number of writhes and both phases of the time of paw licking, while the reaction time on a hot plate and the pain latency duration by the tail-immersion assay were increased. EODL and CO were effective against the paw edema, exudate volume, leucocyte migration and ear edema. EODL and CO also showed in vitro antioxidant activity. These results suggest that caryophyllene oxide is one of the active compounds found in EODL and could partially justify the bioactivity of this oil.
Key words: Duguetia lanceolata, essential oil, caryophyllene oxide, antinociceptive activity, anti-inflammatory activity, antioxidant activity.
Nociceptive pain derived from a tissue injury occurs due to activation of nociceptors that send signals to the central nervous system in response to a potentially harmful stimulus (Dubin and Patapoutian, 2010; Rodriguez, 2015). The nociceptors are then sensitized by the action of mediators such as acetylcholine, bradykinin, histamine, serotonin, leukotriene, substance P, platelet activating factor, acid radicals, potassium ions, prostaglandins, thromboxane, interleukins, tumor necrosis factor (TNF), nerve growth factor (NGF) and cyclicadenosine monophosphate (cAMP), which are present in the tissue environment (Amaya et al., 2013). These mediators are also associated with the development of inflammation. Inflammation is characterized by vasodilatation, increase permeability of the capillaries, migration of leucocytes into the tissue and swelling of the tissue cells (Amaya et al., 2013). In addition, pain and inflammation, as well as the generation of free radicals, have been related to various pathological conditions, including cardiovascular and metabolic complications, diabetes, peptic ulcer, cancer, neurodegenerative diseases (Sen et al., 2010; Carbone and Montecucco, 2015; Fakhoury, 2015). Under these conditions involving pain and inflammation, opioid analgesics, corticosteroids and non-steroidal anti-inflammatory drugs have been widely used (Coutinho and Chapman, 2011; Bacchi et al., 2012; Sousa et al., 2013; Ren et al., 2015). However, the adverse effects of these drugs, such as sedation, dizziness, nausea, vomiting, constipation, physical dependence, tolerance, and respiratory depression, irritation of gastric mucosa and ulcer, water retention and nephrotoxicity, have compromised their therapeutic applications (Benyamin et al., 2008; Slater et al., 2010; Teslim et al., 2014). On the other hand, alternative therapies, highlighting acupuncture, homeopathy, anthroposophy and, especially, herbal medicine have received great attention in recent decades for the treatment of different disorders (Pak et al., 2015). The use of natural products, as essential oils and their components, has been shown large pharmaceutical and pharmacological potentials for the treatment of pain and inflammation associated with oxidative damage (Edris, 2007; Miguel, 2010).
Essential oils belong to the terpenes (mono- and sesquiterpenes), phenylpropanoids and polyketides (Bakkali et al., 2008; Miguel, 2010). These compounds have been studied for their spasmolytic, anxiolytic, anticonvulsant, antinociceptive, anti-inflammatory and antioxidant properties (Bakkali et al., 2008; Miguel, 2010; Wei and Shibamoto, 2010; Sousa, 2011). Regarding the biological activities and the action of oil constituents, the anti-inflammatory activity, for example, has been revealed for limonene, linalyl acetate, β-trans-caryophyllene, 1,8-cineole, p-cymene, thymol, eugenol, chamazulene and α-bisabolol that have inhibitory action on lipoxygenase, an important enzyme involved in the leukotriene pathway (Wei and Shibamoto, 2010; Kamatou and Viljoen, 2010). Another mechanism is based on the action of 1,8-cineole, santoline, spathulenol and caryophyllene oxide, α-pinene, camphor and p-cymene that have a capacity to inhibit the pro-inflammatory interleukins expression (IL-1β and IL-6) (Chao et al., 2005; Juhás et al., 2009). In addition, α-humulene and trans-caryophyllene are able to inhibit the NF-kB activation and neutrophil migration (Passos et al., 2007). These actions can also be related to the antinociceptive effects of components of essential oils since caryophyllene oxide is active in nociception and inflammation models (Chavan et al., 2010). However, compounds as (-)-linalool and (-)-menthol possess analgesic action by their interaction with several receptors, together with opioids, adenosine A1 and A2, cholinergic M2, and produces changes in K+ channels (Sousa, 2011).
Duguetia lanceolata St. Hil. (Family: Annonaceae) known as “pindaíba”, can be mainly found at the Brazilian Cerrado and Atlantic Forest in Minas Gerais, Mato Grosso and São Paulo States (Sousa et al., 2008). This plant has been used as traditional medicine for various purposes such as anti-inflammatory, cicatrizing, analgesic, sedative and antimicrobial (Sousa et al., 2004, 2008). From the chemical point of view, b-elemene, caryophyllene oxide, b-selinene, b-eudesmol and humulene epoxide II are the most concentrated components in the essential oil from barks and this oil has antimicrobial and cytotoxic properties (Sousa et al., 2012). In addition, the alkaloidal fraction produced a yield of 0.34% (Fischer et al., 2004). Pharmacological studies have proven the antinociceptive and anti-inflammatory (Sousa et al., 2004, 2008) and antiplasmodial (Fischer et al., 2004) activities.
Chemically and pharmacologically, the essential oil from D. lanceolata branches (EODL) has not been previously studied, despite few reports published by our research group using other parts (barks and leaves) of this plant species. Based on this fact, in order to establish scientific evidence for the therapeutic uses, the present study evaluated the chemical composition and the antinociceptive, anti-inflammatory and antioxidant activities of EODL using in- vivo and vitro tests. For a better understanding of these activities, caryophyllene oxide (CO), a reference component, was also assessed.
Plant material collection, identification and preparation
Plant material from D. lanceolata St. Hil. was collected from the city of Juiz de Fora, Minas Gerais State, Brazil (43° 21’ 01” W longitude, 21° 45’ 51” S latitude) in April 2010. A voucher specimen (CESJ no 29750) was identified, authenticated and deposited in the Herbarium Leopoldo Krieger of the Federal University of Juiz de Fora, Juiz de Fora, MG, Brazil. After collection of plant, the branches were dried at room temperature and triturated in an electric grinder for the extraction of the essential oil.
Extraction of the essential oil
Dried and powdered branches (100 g) of D. lanceolata were subjected to extraction by hydrodistillation using Clevenger-type apparatus for 2 h at 100°C. After extraction, the essential oil was dried over anhydrous sodium sulphate and stored in sealed vials at low temperature (-18°C) (Lucchesi et al., 2004).
GC-FID and GC/MS analyses
EODL was subjected to the analysis by gas chromatography coupled with flame ionization detector (GC-FID) and gas chromatography coupled with mass spectrometry (GC-MS). Quantitative and qualitative analysis of EODL were carried out by using a Shimadzu GC 2010 machine equipped with a ZB-1MS fused silica capillary column (30 m × 0.25 mm × 0.25 μm film thickness). Analysis conditions by GC-FID were as follows: injector temperature, 260°C, detector temperature, 290°C and programmed temperature from 60 to 290°C (10°C/min); hydrogen at 1.0 mL/min was used as carrier gas. Using these conditions, the percentages of the compounds were obtained.
The GC/MS qualitative analysis of EODL was performed by using a GC-QP2010 PLUS Shimadzu machine with a ZB-5MS fused silica capillary column (30 m × 0.25 mm × 0.25 μm film thickness). The parameters used in this analysis were as follows: injector temperature, 270°C, detector temperature, 290°C and programmed temperature from 60 to 290°C (3°C/min); helium at 1.0 mL/min was used as carrier gas. EODL components were identified by comparison of their retention indices and mass spectra with published data (Adams, 2007) and computer matching with WILEY 275 and National Institute of Standards and Technology (NIST 3.0) libraries provided with the computer controlling the GC-MS system. The retention indices were calculated for all volatile constituents using the retention data of linear C8–C24 n-alkanes.
Chemicals and reagents
Chemical and reagents used in this study were as follows: Croton oil, arachidonic acid, capsaicin, dexamethasone, indomethacin, phosphoric acid, rutin and (-)-caryophyllene oxide (Sigma-Aldrich, St. Louis, MO, USA), acetic acid (Vetec Química Farm. Ltda, Rio de Janeiro, RJ, Brazil), potassium ferrocyanide (Labsynth, Diadema, SP, Brazil), formaldehyde and acetylsalicylic acid (Reagen Quimibrás Ind. Química S.A., Rio de Janeiro, Brazil), ascorbic acid (Cromoline Química Fina, Diadema, SP, Brazil), DPPH, morphine hydrochloride (Merck Inc., Whitehouse Station, NJ, USA), naloxone, carrageenan (Sigma Chemical Co, St. Louis, MO, USA), ketamine chloride and xylazine chloride (Syntec, Hortolândia, SP, Brazil).
Animals
Male Wistar rats (90–110 days, weighing 180–220 g) and male Swiss albino mice (50–70 days, weighing 25–30 g) were used for the experiments. All animals were obtained from Central Biotery of the Federal University of Juiz de Fora and the experimental procedures were performed in the Laboratory of Pharmacology of Natural Products of this institution. The animals were grouped and housed in plastic cages (47 × 34 × 18 cm3) under a 12 h light/12 h dark cycle at room temperature (22±2°C), fed with the balanced feed (Nuvilab Rodents - Nuvital Nutrients, Colombo, Brazil) and received water. After each procedure, the animals were euthanized with an overdose of ketamine and xylazine. Animal care and the experimental protocol were in accordance with the principles and guidelines recommended by the Brazilian College of Animal Experimentation (COBEA) and the rules of the Council for International Organizations of Medical Sciences. Experiments were approved by the local Ethical Committee (protocol number 049/2012).
Acute toxicity
In this procedure, mice were divided into groups of ten animals and received oral doses of 0.5, 1, 1.5, 2 and 3 g/kg of EODL dissolved in sterile saline containing 1% DMSO, and the control group was administered with the vehicle (sterile saline in 1% DMSO). The groups were observed for 48 h and at the end of this period, the mortality was recorded for each group. General signs of toxicity, motor activity and mortality parameters were recorded for 48 h. The 50% lethal dose (LD50) was determined by probit test using a percentage of death versus doses’ log (Litchfield and Wilcoxon, 1949).
Writhing test
The writhing test was performed according to the method described by Collier et al. (1986) using 0.6% acetic acid as inducer of nociception. Initially, groups of mice (n = 8) were treated with EODL (50, 100 and 200 mg/kg) and CO (10, 25 and 50 mg/kg) orally. The negative control group received, sterile saline in 1% DMSO (control group, 10 mL/kg, p.o.) orally, while the positive control group was administrated acetylsalicylic acid (200 mg/kg, p.o.) and indomethacin (10 mg/kg, p.o.). After 1 h of treatment, intraperitoneal injections (0.25 mL) of 0.6% acetic acid were administered. The total number of writhes was quantified between 10 and 30 min after injection of acetic acid. At this time interval, the acetic acid produced less variation in the number of writhes in each experimental group.
Formalin test
In this experiment, the methodology described by Hunskaar and Hole (1987) was used. The animals were pre-treated with EODL (50, 100 and 200 mg/kg, p.o.), CO (10, 25 and 50 mg/kg, p.o.) and morphine (5 mg/kg, subcutaneous, s.c.). Control animals were treated with similar volume of sterile saline in 1% DMSO (10 mL/kg, p.o.). After 1 h of treatment, 20 µL of 2.5% formalin (in 0.9% saline) were injected under the skin of the dorsal surface of the right hindpaw. The duration of paw licking was determined between 0 and 5 min (first phase) and 15 and 30 min (second phase) after formalin injection. These intervals were established to dissociate nociceptive and inflammatory pain, respectively.
Hot plate test
Following the methodology described by Eddy and Leimbach (1953), the mice were grouped (n = 8) and treated with EODL (50, 100 and 200 mg/kg, p.o.), CO (10, 25 and 50 mg/kg, p.o.), morphine (5 mg/kg, s.c.) and sterile saline in 1% DMSO (10 mL/kg, p.o.). In addition, the effect of EODL (200 mg/kg, p.o.), CO (50 mg/kg, p.o.) and morphine (5 mg/kg, s.c.) in the presence of naloxone (2 mg/kg, s.c.) were also evaluated in separate groups of animals (n = 8). After drug administration, the animals were placed on a hot-plate (Model LE 7406, Letica Scientific Instruments, Barcelona, Spain) at 55 ± 1°C and the reaction times were measured at 0, 30, 60 and 90 min observing two behavioral components, namely paw licking and jumping. To avoid animal paw lesion, the cut-off time was set at 30 s.
Tail immersion test
Based on the description of Ramabadran et al. (1989), the animals were divided into groups of eight animals (n = 8) to perform the tail immersion test. The negative control received sterile saline in 1% DMSO (10 mL/kg, p.o.) orally, while morphine (5 mg/kg, s.c.) was administrated in positive control subcutaneously. Three groups of mice were treated with EODL (50, 100 and 200 mg/kg, p.o.) and three others with CO (10, 25 and 50 mg/kg, p.o.). Then, the reaction times of animals were recorded at 0, 30, 60, 90 and 120 min after the tail immersion in water maintained at a constant temperature (55±1°C). To avoid tissue damage, the cut-off was set at 10 s. In this test, immersion of an animal’s tail in hot water provokes an abrupt movement of the tail and sometimes the recoiling of the whole body.
Carrageenan-induced paw edema method
Carrageenan-induced paw edema in Wistar rats was performed according to the method described previously by Winter et al. (1962). The animals were divided into groups of six, which received oral doses of EODL (50, 100 and 200 mg/kg, p.o.), CO (10, 25 and 50 mg/kg, p.o.), sterile saline in 1% DMSO (10 mL/kg, p.o.) or indomethacin (10 mg/kg, p.o.).
After 60 min of treatment, the paw edema was induced by the injection of 0.1 mL 1% carrageenan (an edematogenic agent) into the subplantar region of the right hind paw of the rat, while in the left paw (used as control), 0.1 mL of sterile saline was injected. The measurement of paw edema was calculated by the difference between the volume displaced by the right and the left paw using a plethysmometer (model LE 7500, Letica Scientific Instruments, Barcelona, Spain) and evaluated at 1, 2, 3 and 4 h postcarrageenan response.
Carrageenan-induced pleurisy method
Using the methodology described by Vinegar et al. (1973) with some modifications, eight groups of animals were separated with six Wistar rats each (n = 6). The groups were treated with EODL (50, 100, and 200 mg/kg, p.o.), CO (10, 25, and 50 mg/kg, p.o.), sterile saline in 1% DMSO (p.o.) or indomethacin (10 mg/kg, p.o.). After 1 h of treatment, the pleurisy was induced by intrapleural administration of 0.4 mL 1% carrageenan suspension in sterile saline between the third and fifth ribs on the right side of the mediastinum.
Animals were euthanized with an overdose of ketamine and xylazine after 4 h of carrageenan injection, and the skin and pectoral muscles were retracted. A longitudinal incision was made between the third and fifth ribs on each side of the mediastinum. Then, the exudate was collected and transferred to a 15 mL conical centrifuge tube and the total volume was determined. A 20 µL aliquot of the exudate was used to determine the total leucocytes using Neubauer haemocytometer chamber under microscopic analysis.
Croton oil-induced ear edema
Croton oil-induced ear edema in mice was performed as described by Schiantarelli et al. (1982). Eight groups with eight mice each (n = 8) were separated and 20 μL of 2.5% croton oil (v/v, diluted in acetone) were administrated topically on the inner surface of the right ear. To accompany the baseline in the groups, 20 μL of acetone (vehicle) were applied in the left ear. After 15 min, the right ears were treated with EODL (0.1, 0.5 and 1.0 mg/ear), CO (0.1, 0.5 and 1.0 mg/ear) or dexamethasone (0.1 mg/ear, positive control), while the negative control (untreated) received 20 μL of acetone. The weight of the ear edema (mg) was evaluated after 6 h of croton oil application by the difference between the masses of the right and left ears.
Arachidonic acid-induced ear edema
Edema was induced in mice (n = 8/group) by topical administration on the inner surface of the right ear using arachidonic acid (AA) (2.0 mg/ear in 20 μL of acetone) according to Young et al. (1984). The left ear received 20 μL of acetone as vehicle. After 15 min, the right ear was topically treated with EODL (0.1, 0.5 and 1.0 mg/ear in 20 μL of acetone), CO (0.1, 0.5 and 1.0 mg/ear in 20 μL of acetone), and indomethacin (2.0 mg/ear in 20 μL of acetone, positive control). The negative control (untreated) received topically 20 μL of acetone on the right ear. As in croton oil test, the weight (mg) of the ear edema was evaluated after 1 h of AA application by the difference between the masses of the right and left ears.
DPPH radical scavenging activity
Using the stable DPPH free radical, the free radical-scavenging activity of EODL and CO was determined by the method of Mensor et al. (2001). For this reaction, EODL (60 to 280 µg/mL) and CO (60 to 280 µg/mL) were prepared and added to an equal volume (0.03 mM) of DPPH in test tubes in triplicate. After 60 min, the absorbance was recorded at 518 nm. Rutin was used as standard control. EC50 values denote the concentration (μg/mL) of sample, which is required to scavenge 50% of DPPH free radicals.
Test of iron reducing power
The iron reducing power of EODL and CO was determined according to the method described by Oyaizu (1986). EODL (80 to 320 µg/mL) and CO (80 to 320 µg/mL) were prepared with 0.2 mM phosphate buffer pH 6.6 and 1% potassium ferrocyanide incubation at 50°C for 20 min. This mixture was added to 10% trichloroacetic acid followed by centrifugation at 3000 g for 10 min. Then, the supernatant was mixed with 1% ferric chloride and the absorbance measured at 700 nm in triplicate. Ascorbic acid (0.5 to 10µg/mL) was used as reference substance. EC50 (50% effective concentration) values (µg/mL) were calculated and indicated the effective concentration at which the absorbance was 0.5 for reducing power.
ð›½-Carotene/linoleic acid bleaching assay
ð›½-Carotene bleaching assay was carried out according to the method developed by Miller (1971). For this, 0.2 mg/mL ð›½-carotene (1 mL) diluted in chloroform was mixed with linoleic acid (0.02 mL) and Tween 20 (0.2 mL). Using the rotary evaporator (BUCHI, Germany), the chloroform was removed and the mixture was diluted with 100 mL of distilled water to form an emulsion. The emulsion (5 mL) was transferred into different test tubes containing EODL (38.46 to 1.20 μg/mL) and CO (38.46 to 1.20 μg/mL) and placed in a water bath at 50°C for 2 h. Absorbance of the samples was measured at every 15 min for 105 min at 470 nm using a spectrophotometer (UV-VIS Spectrophotometer, Shimadzu). Rutin and BHT were used as positive control (at the same concentration as samples).
All samples were assayed in triplicate and the results were expressed in percentage of inhibition of lipid peroxidation (%) which is the sample concentration providing 50% inhibition of linoleic acid oxidation.
Statistical analysis
Data are expressed as mean ± S.E.M. Statistical significance was analyzed by the one-way analysis of variance (ANOVA) followed by the Student Newman-Keuls or Tukey test. P values below 0.05 were considered significant. The percentage of inhibition was calculated by using: 100 − T × 100/C (%) or T × 100/C – 100 (%); where C and T indicate non-treated (vehicle) and drug-treated, respectively (Jaiswal and Sontakke, 2012). The Graph Pad® Prism 5.0. Software was used for statistical analyses.
Chemical characterization by GC/MS
A total of thirty-seven compounds were identified in EODL and classified as monoterpene hydrocarbons, oxygenated monoterpenes, sesquiterpene hydrocarbons, oxygenated sesquiterpenes and hydrocarbons (Table 1). Among the terpenes identified, thirteen were monoterpenes and twenty-two were sesquiterpenes. b-Pinene (1.1%) and trans-pinocarveol (1.0%) were revealed as the most abundant monoterpenes. Considering the sesquiterpenes, b-elemene (8.3%), caryophyllene oxide (7.7%), b-eudesmol (7.2%), b-selinene (7.1%), humulene epoxide II (6.6%) and β-caryophyllene (6.2%) were the most concentrated components.
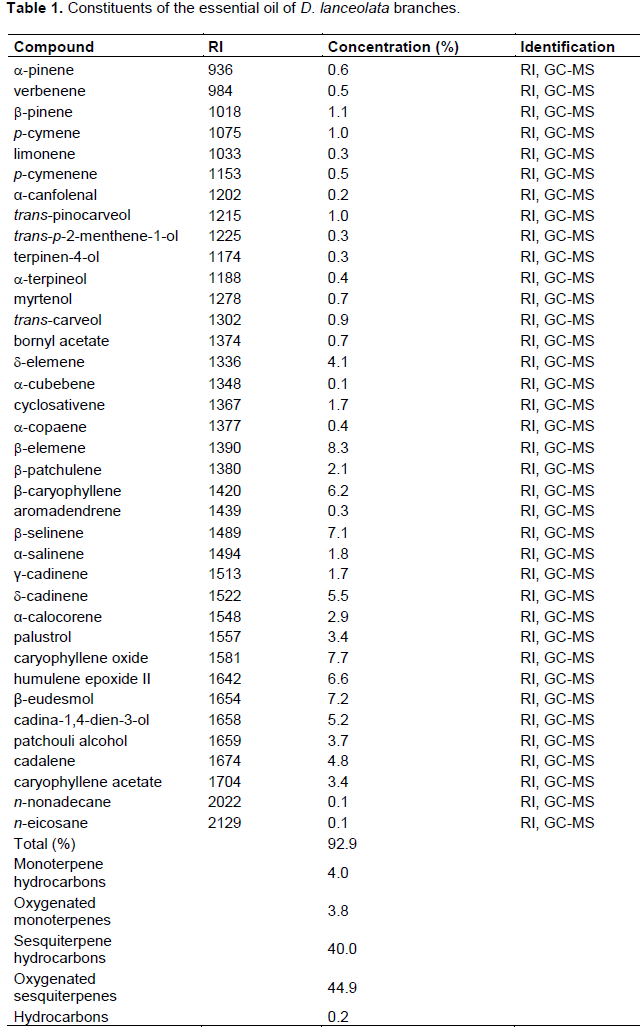

Acute toxicity
After 48 h of treatment, EODL was toxic to mice with LD50 of 2.9 g/kg (95% confidence intervals 1.7-4.9 g/kg). General signs of toxicity (as cyanosis, piloerection, writhing, ptosis, tremors, convulsions, ataxia, hypnosis, red urine and diarrhea) were not detected. The parameters motor activity, such as respiration, corneal reflex, righting and withdrawal, body tone and amount of pats, were also unaffected.
Effects of EODL and CO on acetic acid-induced writhing
The treatment with EODL (100 and 200 mg/kg, p.o.) produced a significant inhibition of the abdominal writhes when compared with the control (Figure 1). After administration of CO, an interesting antinociceptive effect was also observed at doses of 10, 25 and 50 mg/kg with reduction of the number of writhes in 7.81, 11.43 and 13.23%, respectively (Figure 1). As drug references, ASA and indomethacin were effectives against acetic acid-induced nociception.
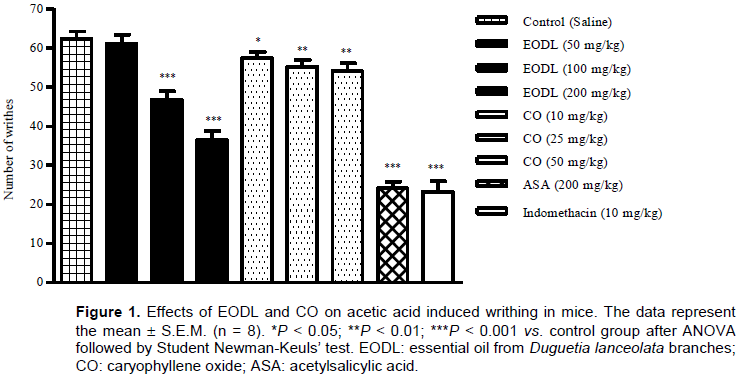
Effects of EODL and CO on the formalin test
The intraplantar injection of formalin promoted a biphasic characteristic response (first and second phases) (Figure 2). In the first phase (neurogenic), EODL caused a significant and dose-dependent inhibition in the licking time at the doses of 50 (14.34%), 100 (31.3%) and 200 mg/kg (44.4%), while CO reduced the paw licking time by 9.6 and 21.1% at the doses of 25 and 50 mg/kg, respectively. After treatment, doses of 50, 100 and 200 mg/kg of EODL also inhibited the second phase (inflammatory). CO was active at the second phase, producing a significant response of 10.8 (25 mg/kg) and 13.9% (50 mg/kg). Morphine (5 mg/kg, s.c.), an opioid analgesic, significantly reduced the formalin response in both phases, while indomethacin had no effect in the early phase.
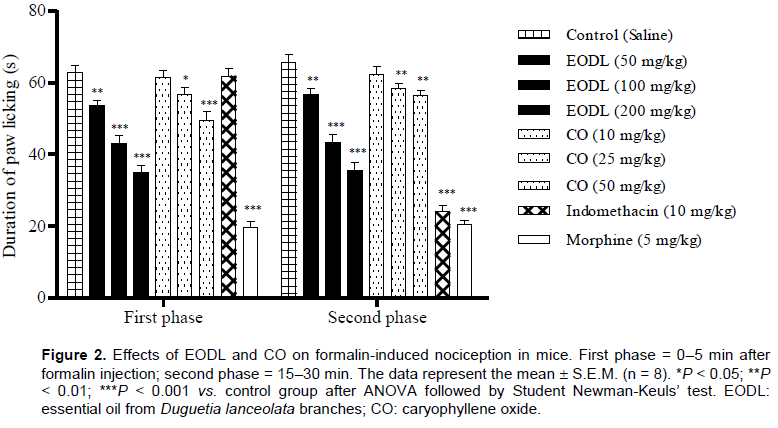
Effects of EODL and CO on the hot plate test
The central effect observed in the first phase of the formalin test was confirmed through the hot plate assay. The effect of EODL and CO in the hot plate test varied according to the doses and the time of observation (Table 2). At 0 and 30 min times, no significant antinociceptive effect was detected. The reaction time in the hot plate significantly increased after 60 and 90 min of treatment with EODL and CO. The treatment was also performed in the presence of naloxone, an opioid antagonist. It was observed that the naloxone was able to inhibit the response of the morphine, but it was not able to completely block the effect of EODL and CO.
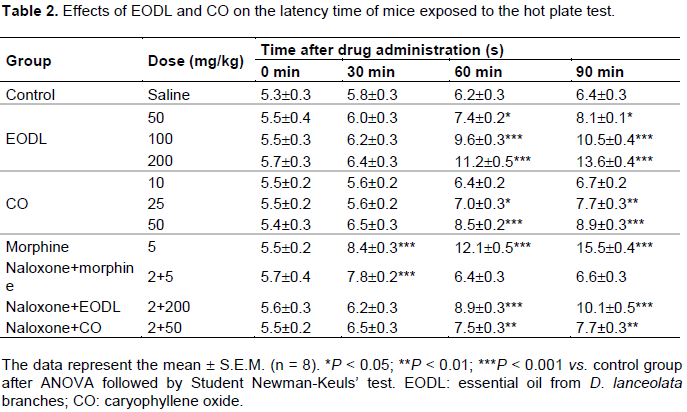
Effects of EODL and CO on the tail immersion test
The antinociceptive effect of EODL and CO on the tail immersion latencies is shown in Table 3. After 90 and 120 min of treatment, doses of 100 and 200 mg/kg of EODL and 25 and 50 mg/kg of CO significantly increased the pain latency duration. Morphine was active after 30 min of treatment.
Effects of EODL and CO on the carrageenan-induced paw edema
In this study, EODL and CO showed a significant inhibitory effect on the edema formation at the 3 and 4 h (Figure 3). At 4 h, EODL produced reduction of the paw edema in 18.3 (50 mg/kg), 32.3 (100 mg/kg) and 44.1% (200 mg/kg). In this time, doses of 10, 25 and 50 mg/kg of CO also reduced the paw edema in 11.8, 18.3 and 31.2%, respectively. Indomethacin reduced the paw edema by 25.9% after 4 h of carrageenan application.
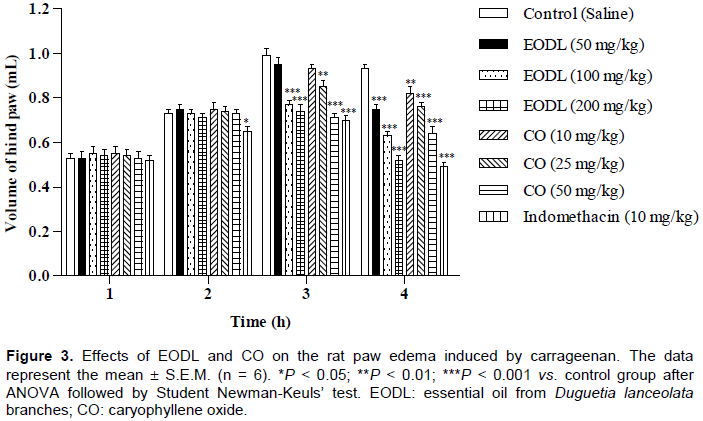
Effects of EODL and CO on the carrageenan-induced pleurisy
There was an intense formation of exudate volume and leucocyte migration in the pleurisy of the control group after 4 h of intrapleural application of carrageenan. EODL at the highest dose of 200 mg/kg significantly reduced the carrageenan induced exudate volume and number of leucocytes (50.0 and 32.2%, respectively) as compared to the indomethacin (10 mg/kg) (60.0 and 37.8%, respectively). Decrease of exudate volume and number of leucocytes was also observed at 50 and 100 mg/kg (Table 4). In addition, these biological parameters were significantly inhibited after treatment with CO (10, 25 and 50 mg/kg).
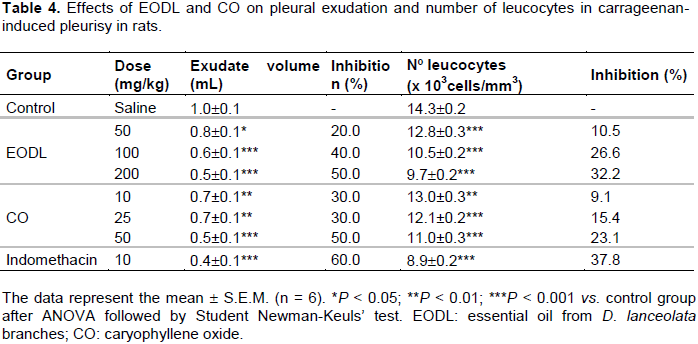
Effect of EODL and CO on the croton oil-induced ear edema
The negative control group demonstrated the greatest degree of edema, while the mice group pretreated with EODL and CO revealed significant reduction of the edema in a concentration dependent manner (Figure 4). The positive control (dexamethasone, 0.1 mg/ear) caused a reduction of the edema by 64.6%.
Effect of EODL and CO on the AA-induced mice ear edema
EODL significantly decreased the ear edema induced by arachidonic acid in a concentration-response manner by 21.9, 34.1 and 46.3%, at 0.1, 0.5 and 1.0 mg/ear, respectively. On the other hand, only 1.0 mg/ear of CO was able to inhibit ear edema by 19.5%. The level of inhibition induced by indomethacin (2.0 mg/ear) was 51.2% (Figure 5).
DPPH radical scavenging, Fe+3 reducing power and β-carotene bleaching antioxidant activity assessment
The DPPH activity of EODL was higher than that of Fe+3 reducing power (Table 5). Using DPPH radical, CO produced EC50 greater than 280 μg/mL. In addition, EODL and CO inhibited the lipid peroxidation in 41.5 and 24.1%, respectively. As expected, rutin, ascorbic acid and BHT exhibited potent antioxidant activity (Table 5).
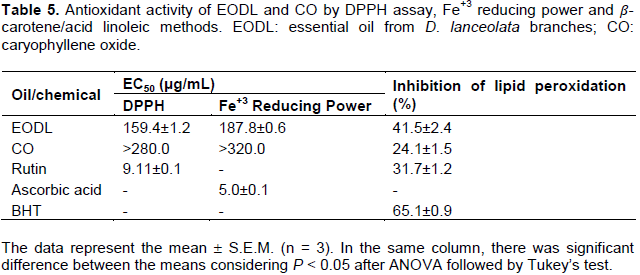
One previous chemical study on the essential oil obtained from D. lanceolata barks performed by our research group revealed that the major components were sesquiterpenes, b-elemene, caryophyllene oxide, b-selinene, b-eudesmol and humulene epoxide II using GC/MS (Sousa et al., 2012). In the present study, 37 compounds were identified, which accounts for 92.7% of the total oil content with the presence of mono- and sesquiterpenes. Despite the difference in relation to the total oil content and number of components, the chemical composition was similar to barks’ oil (Sousa et al., 2012). Interestingly, although some compounds have been found in other species of Duguetia, the divergent data regarding the previous component characterization might be related to the constitution of the plant material (Maia et al., 2006; Almeida et al., 2010). It is possible that the similarity of compounds into genus has a chemotaxonomic significance and the major compounds can represent the chemical markers of EODL. In addition, essential oils and their components have been associated with several biological properties, antinociceptive, anti-inflammatory and antioxidant activities (Bakkali et al., 2008; Miguel, 2010).
The acute toxicity test revealed that EODL (LD50 of 2.9 g/kg) can be toxic to mice and this data appeared as crucial to define the doses in the pharmacological experiments (50, 100 and 200 mg/kg). In this test, the major signs of toxicity and parameters motor activity were not affected. However, considering the components found in EODL, β-elemene showed low toxicity when administered to cancer patients (Chen et al., 2012; Li et al., 2013), while CO inhibited the mitochondrial electron transport chain having high toxicity (Monzote et al., 2009). Therefore, our findings are relevant as regard the safety of the use of EODL in the medicinal applications.
The present results show that EODL and CO have antinociceptive effect since they reduced the abdominal writhes in mice (Figure 1). The mechanism might be associated with the blocking of the cyclooxygenase and/or lipoxygenase pathways preventing formation of inflammatory mediators, as prostaglandins (PGE2 and PGF2α) (Deraedt et al., 1980; Schmidt et al., 2010). After application of acetic acid, these mediators were generated in the abdominal cavity and contributed to the increased sensitivity to nociceptors activating the ascending pathways of pain (Deraedt et al., 1980; Schmidt et al., 2010). However, this finding confirms the analgesic effect of CO described by Chavan et al. (2010) and is similar to that reported for the barks’ oil (Sousa et al., 2004) and ethanol extract from leaves of D. lanceolata (Sousa et al., 2008).
According to Shibata et al. (1989), the formalin-induced pain produces mediators such as substance P and bradykinin which participate in the manifestation of the first phase, while histamine, serotonin, prostaglandin and bradykinin are involved in the second phase causing central and peripheral responses, respectively. The present study results (Figure 2) show that EODL and CO are able to promote a significant inhibition in both phases by blocking the direct stimulation of nociceptors (central effect; 0 to 5 min) and inflammatory response (peripheral effect; 15 to 30 min) (Hunskaar and Hole, 1987; Shibata et al., 1989; Tjolsen et al., 1992). These effects are similar to the actions of opioid and nonsteroidal anti-inflammatory drugs. It is worth mentioning that this data has not been previously described using the formalin test, but research conducted by our group demonstrated these effects with the barks’ oil (Sousa et al., 2004) and ethanol extract of the leaves (Sousa et al., 2008).
The hypothesis of central effect revealed in the formalin test was investigated using two animal models (hot plate and tail immersion methods) to check the action on opioid receptors in the spinal and supraspinatus levels (Le Bars et al., 2001). This research results demonstrated that the oral administration of EODL and CO exerted significant prolongation in the response latency time to the heat stimulus (Tables 2 and 3) showing a central analgesic effect, particularly on µ opioid receptors (Le Bars et al., 2001). In this test, the central effect of EODL and CO might also be associated with non-opioid system as pretreatment with naloxone did not completely changed the latency time (Table 2), which was also observed in a previous study using the ethanol extract of D. lanceolata leaves (Sousa et al., 2008). Although CO has revealed an antinociceptive effect using hot plate test in a study by Chavan et al. (2010), the data showed that this effect may involve additional mechanisms on non-opioid systems. Considering the tail immersion assay, the lack of response at the dose of 50 mg/kg may be due to the greater sensitivity of this model, since it is a spinal integration (reflex) (Carstens and Wilson, 1993).
Initially, the anti-inflammatory effect of EODL and CO (indicated in the formalin test) was investigated using carrageenan-induced paw edema method. These results showed a significant reduction in paw edema which may be associated with the inhibition of prostaglandins biosynthesis that appears in the second phase (3 to 5 h) of the postcarrageenan response (Necas and Bartosikova, 2013) and were similar to the studies reported by Sousa et al. (2004, 2008). However, for a better understanding of this effect, the inflammatory response against carrageenan-induced pleurisy was evaluated. As described by Ammendola et al. (1975), cyclooxygenase inhibitors (indomethacin, phenylbutazone, aspirin and flufenamic acid) are able to reduce the exudate volume and leucocytes migration between 3 and 6 h after application of carrageenan. Thus, as shownin Table 4, EODL and CO inhibited these inflammatory parameters after 4 h of carrageenan application, producing a similar action to the anti-inflammatory agents. Therefore, EODL and CO played a crucial role as protective factors against the carrageenan-induced acute inflammation.
The ear edema models have often been used to corroborate the findings obtained from other methods of acute inflammation through a better understanding of the mechanisms involved in the pharmacological responses. However, although the mechanisms are similar, the topical route has a great advantage in establishing products that can be applied in inflammatory skin diseases (such as psoriasis and allergic contact dermatitis) (Nestle et al., 2009). Based on this viewpoint, the croton oil and arachidonic acid were used as skin inflammation inducers and evaluated in the presence of EODL and CO. According to Gábor (2000), the topical application of croton oil promotes an inflammatory response with oxidative stress that increases vascular permeability, edema formation and leucocyte migration with release of prostaglandin E2, leukotrienes, histamine, serotonin and IL-1. These mediators are generated through different activation pathways and are blocked by cyclooxygenase and 5-lipoxygenase inhibitors, corticosteroids (for example dexamethasone), and leukotriene B4 receptor antagonists. The results showed that EODL and CO were effective on mice ear edema induced by croton oil in a concentration dependent manner. Based on this evidence and in the results of the nociception (abdominal writhing and formalin) and inflammation (paw edema and pleurisy) tests described above, at least, one of the mechanisms involved is associated with the inhibition of prostaglandin synthesis. In this sense, the results of the inhibitory action of EODL and CO on the ear edema induced by arachidonic acid confirmed this hypothesis (Figure 5).
In recent decades, essential oils have been targeted for investigation due to its ability to scavenge free radicals and reduce cellular damages that are related to different pathologies, particularly those associated with inflammatory processes (Miguel, 2010; Adorjan and Buchbauer, 2010). The present study findings revealed that EODL possesses antioxidant effect using DPPH, reducing power and ð›½-carotene assays, while CO inhibited the lipid peroxidation. Nevertheless, considering the antinociceptive and anti-inflammatory properties supported in this study, the antioxidant action of EODL and CO may partially clarify such properties, since reactive oxygen species (ROS) have been implicated in inflammatory response with generation of inflammatory mediators, edema formation, leucocyte migration and pain (Miguel, 2010). It is important to note that the antinociceptive activity of EODL and CO is also related to the reduction of the liberation of these mediators which are induced by ROS.
Finally, the effects described in this work can be assigned to other components of EODL. Limonene, β-trans-caryophyllene, p-cymene and α-pinene are able to inhibit enzyme involved in the leukotriene pathway or the blocking of the production of pro-inflammatory interleukins (IL-1β and IL-6) (Chao et al., 2005; Juhás et al., 2009; Wei and Shibamoto, 2010). Analgesic and anti-inflammatory effects of the essential oil of Ugni myricoides are associated with its main components (caryophyllene oxide, humulene epoxide II and β-caryophyllene), especially α-pinene (52.1%) (Quintão et al. 2010).
The results were obtained through the in- vivo and vitro well-established experiments performed in the present study by adding more subsidies for the use of essential oil from D. lanceolata branches due to their antinociceptive, anti-inflammatory and antioxidant properties. Based on the data, caryophyllene oxide is one of the active constituents of this essential oil and represents a promising target for the treatment of pain, inflammation and oxidative stress damage. However, further studies should be conducted to ensure the safety, feasibility and sustainability of usage.
The authors have not declared any conflict of interest.
This work was supported by the Coordenação de Aperfeiçoamento de Pessoal de Nível Superior (CAPES), Conselho Nacional de Desenvolvimento Científico e Tecnológico (CNPq), Fundação de Amparo à Pesquisa do Estado de Minas Gerais (FAPEMIG) and Pró-Reitoria de Pós-Graduação, Pesquisa e Inovação of the Federal University of Juiz de Fora. The authors are grateful to Éder Luis Tostes and Jésus de Paula Sarmento for the technical support.
REFERENCES
Adams RP (2007). Identification of Essential Oil Components by Gas Chromatography/Mass Spectrometry. 4th ed.; Allured Publishing Co.: Carol Stream, IL, USA.
|
|
Adorjan B, Buchbauer G (2010). Biological properties of essential oils: An updated review. Flavour Frag. J. 25:407-426.
|
|
Almeida JRG, Facanali R, Vieira MAR, Marques MOM, Lúcio ASSC, Lima EO, Agra MF, Barbosa-Filho JM (2010). Composition and antimicrobial activity of the leaf essential oils of Duguetia gardneriana Mart. and Duguetia moricandiana Mart. (Annonaceae). J. Essent. Oil Res. 22:275-278.
Crossref
|
|
Amaya F, Izumi Y, Matsuda M, Sasaki M (2013). Tissue injury and related mediators of pain exacerbation. Curr. Neuropharmacol. 11:592-597.
Crossref
|
|
Ammendola G, Di Rosa M, Sorrentino L (1975). Leucocyte migration and lysosomal enzymes release in rat carrageenin pleurisy. Agents Actions 5:250-255.
Crossref
|
|
Bacchi S, Palumbo P, Sponta A, Coppolino MF (2012). Clinical pharmacology of non-steroidal anti-inflammatory drugs: A review. Antiinflamm. Antiallergy Agents Med. Chem. 11:52-64.
Crossref
|
|
Bakkali F, Averbeck S, Averbeck D, Idaomar M (2008). Biological effects of essential oils – A review. Food Chem. Toxicol. 46:446-475.
Crossref
|
|
Benyamin R, Trescot AM, Datta S, Buenaventura R, Adlaka R, Sehgal N, Glaser SE, Vallejo R (2008). Opioid complications and side effects. Pain Phys.11:S105-S120.
|
|
Carbone F, Montecucco F (2015). Inflammation in arterial diseases. IUBMB Life 67:18-28.
Crossref
|
|
Carstens E, Wilson C (1993). Rat tail flick reflex: Magnitude measurement of stimulus-response function, suppression by morphine and habituation. J. Neurophysiol. 70:630-639.
|
|
Chao LK, Hua K-F, Hsu H-Y, Cheng S-S, Liu J-Y, Chang S-T (2005). Study of the anti-inflammatory activity of essential oil from leaves of Cinnamomum osmophloeum. J. Agric. Food Chem. 53:7274-7278.
Crossref
|
|
Chavan MJ, Wakte PS, Shinde DB (2010). Analgesic and anti-inflammatory activity of caryophyllene oxide from Annona squamosa L. bark. Phytomedicine 17:149-151.
Crossref
|
|
Chen M, Zhang J, Yu S, Wang S, Zhang Z, Chen J, Xiao J, Wang Y (2012). Anti-lung-cancer activity and liposome-based delivery systems of β-elemene. Evid. Based Complement. Altern. Med. 2012:1-5.
Crossref
|
|
Collier HO, Dinneen LC, Johnson CA, Schneider C (1986). The abdominal response and its suppression by analgesic drugs in the mouse. Br. J. Pharmacol. Chemother. 32:295-310.
Crossref
|
|
Coutinho AE, Chapman KE (2011). The anti-inflammatory and immunosuppressive effects of glucocorticoids, recent developments and mechanistic insights. Mol. Cell. Endocrinol. 335:2-13.
Crossref
|
|
Deraedt R, Jouquey S, Delevallée F, Flahaut M (1980). Release of prostaglandins E and F in an algogenic reaction and its inhibition. Eur. J. Pharmacol. 61:17-24.
Crossref
|
|
Dubin AE, Patapoutian A (2010). Nociceptors: the sensors of the pain pathway. J. Clin. Invest. 120:3760-3772.
Crossref
|
|
Eddy NB, Leimbach D (1953). Synthetic analgesics. II. Dithienylbutenyl-and dithienylbutylamines. J. Pharmacol. Exp. Ther. 107:385-393.
|
|
Edris AE (2007). Pharmaceutical and therapeutic potentials of essential oils and their individual volatile constituents: A review. Phytother. Res. 21:308-323.
Crossref
|
|
Fakhoury M (2015). Role of immunity and inflammation in the pathophysiology of neurodegenerative diseases. Neurodegener. Dis. 15:63-69.
Crossref
|
|
Fischer DC, Gualda NCA, Bachiega D, Carvalho CS, Lupo FN, Bonotto SV, Alves MO, Yogi A, Santi SM, Ávila PE, Kirchgatter K, Moreno PR (2004). In vitro screening for antiplasmodial activity of isoquinoline alkaloids from Brazilian plant species. Acta Trop. 92:261-266.
Crossref
|
|
Gábor M (2000). Mouse ear inflammation models and their pharmacological applications. AkadémiaiKiadó: Budapest, Hungary.
|
|
Hunskaar S, Hole K (1987). The formalin test in mice: Dissociation between inflammatory and noninflammatory pain. Pain 30:103-114.Jaiswal SR, Sontakke SD (2012). Experimental evaluation of analgesic and anti-inflammatory activity of simvastatin and atorvastatin. Indian J. Pharmacol. 44:475-479.
|
|
Juhás Š, Bukovská A, Cikoš Š, Czikková S, Fabian D, Koppel J (2009). Anti-inflammatory effects of Rosmarinus officinalis essential oil in mice. Acta Vet. Brno. 78:121-127.
Crossref
|
|
Kamatou GPP, Viljoen AM (2010). A review of the application and pharmacological properties of α-bisabolol and α-bisabolol-rich oils. J. Am. Oil Chem. Soc. 87:1-7.
Crossref
|
|
Le Bars D, Gozariu M, Cadden SW (2001). Animals models of nociception. Pharmacol. Rev. 53:597-652.
|
|
Li QQ, Wang G, Huang F, Li JM, Cuff CF, Reed E (2013). Sensitization of lung cancer cells to cisplatin by β-elemene is mediated through blockade of cell cycle progression: antitumor efficacies of β-elemene and its synthetic analogs. Med. Oncol. 30:488-498.
Crossref
|
|
Litchfield JT, Wilcoxon FA (1949). A simplified method of evaluating dose-effect experiments. J. Pharmacol. Exp. Ther. 96:99-113.
|
|
Lucchesi ME, Chemat F, Smadja J (2004). Solvent-free microwave extraction of essential oil from aromatic herbs: Comparison with conventional hydro-distillation. J. Chromatogr. A 1043:323-327
Crossref
|
|
Maia JGS, Andrade EHA, Carreira LMM, Oliveira J (2006). Essential oil composition from Duguetia species (Annonaceae). J. Essent. Oil Res. 18:60-63.
Crossref
|
|
Mensor LL, Menezes FS, Leitão GG, Reis AS, Santos TC, Coube CS, Leitão SG (2011). Screening of Brazilian plant extracts for antioxidant activity by the use of DPPH free radical method. Phytother. Res. 15:127-130.
Crossref
|
|
Miguel MG (2010). Antioxidant and anti-inflammatory activities of essential oils: A short review. Molecules 15:9252-9287.
Crossref
|
|
Miller HE (1971). A simplified method for the evaluation of antioxidants. J. Am. Oil Chem. Soc. 48:91.
Crossref
|
|
Monzote L, Stamberg W, Staniek K, Gille L (2009). Toxic effects of carvacrol, caryophyllene oxide, and ascaridole from essential oil of Chenopodium ambrosioides on mitochondria. Toxicol. Appl. Pharmacol. 240:337-347.
Crossref
|
|
Necas J, Bartosikova L (2013). Carrageenan: A review. Vet. Med. 58:187-205.
|
|
Nestle FO, Di Meglio P, Qin J-Z, Nickoloff BJ (2009). Skin immune sentinels in health and disease. Nat. Rev. Immunol. 9:679-691.
Crossref
|
|
Oyaizu M (1986). Studies on product of browning reaction-antioxidative activities of products of browning reaction prepared from glucosamine. Jpn. J. Nutr. Diet. 44:307-315.
Crossref
|
|
Pak SC, Micalos PS, Maria SJ, Lord B (2015). Nonpharmacological interventions for pain management in paramedicine and the emergency setting: A review of the literature. Evid. Based Complement. Altern. Med. 2015:1-8.
Crossref
|
|
Passos GF, Fernandes ES, Cunha FM, Ferreira, J, Pianowski LF, Campos MM, Calixto JB (2007). Anti-inflammatory and anti-allergic properties of the essential oil and active compounds from Cordia verbenacea. J. Ethnopharmacol. 110:323-333.
Crossref
|
|
Quintão NL, Silva GF, Antonialli CS, Rocha LW, Cechinel Filho V, Cicció JF (2010). Chemical composition and evaluation of the anti-hypernociceptive effect of the essential oil extracted from the leaves of Ugni myricoides on inflammatory and neuropathic models of pain in mice. Planta Med. 76:1411-1418.
Crossref
|
|
Ramabadran K, Bansinath M, Turndorf H, Puig MM (1989). Tail immersion test for the evaluation of a nociceptive reaction in mice: Methodological considerations. J. Pharmacol. Meth. 21:21-31.
Crossref
|
|
Ren ZY, Xu XQ, Bao YP, He J, Shi L, Deng JH, Gao XJ, Tang HL, Wang YM, Lu L (2015). The impact of genetic variation on sensitivity to opioid analgesics in patients with postoperative pain: A systematic review and meta-analysis. Pain Phys. 18:131-152.
|
|
Rodriguez L (2015). Pathophysiology of pain: Implications for perioperative nursing. AORN J. 101:338-344.
Crossref
|
|
Schiantarelli P, Cadel S, Acerbi D, Pavesi L (1982). Antiinflammatory activity and bioavailability of percutaneous piroxicam. Arzneimittel-Forschung 32:230-235.
|
|
Schmidt AP, Böhmer AE, Schallenberger C, Antunes C, Tavares RG, Wofchuk ST, Elisabetsky E, Souza DO (2010). Mechanisms involved in the antinociception induced by systemic administration of guanosine in mice. Br. J. Pharmacol. 159:1247-1263.
Crossref
|
|
Sen S, Chakraborty R, Sridhar C, Reddy YSR, De B (2010). Free radicals, antioxidants, diseases and phytomedicines: Current status and future prospect. Int. J. Pharm. Sci. Rev. Res. 3:91-100.
|
|
Shibata M, Ohkubo T, Takahashi H, Inoki R (1989). Modified formalin test: characteristic biphasic pain response. Pain 38:347-352.
Crossref
|
|
Slater D, Kunnathil S, Mcbride J, Koppala R (2010). Pharmacology of nonsteroidal antiinflammatory drugs and opioids. Semin. Intervent. Radiol. 27:400-411.
Crossref
|
|
Sousa DP (2011). Analgesic-like activity of essential oils constituents. Molecules 16:2233-2252.
Crossref
|
|
Sousa LP, Alessandri AL, Pinho V, Teixeira MM (2013). Pharmacological strategies to resolve acute inflammation. Curr. Opin. Pharmacol. 13:625-631.
Crossref
|
|
Sousa OV, Del-Vechio-Vieira G, Alves MS, Araújo AAL, Pinto MAO, Amaral MPH, Rodarte MP, Kaplan MAC (2012). Chemical composition and biological activities of the essential oils from Duguetia lanceolata St. Hil. barks. Molecules 17:11056-11066.
Crossref
|
|
Sousa OV, Del-Vechio-Vieira G, Amaral MPH, Pinho JJRG, Yamamoto CH, Alves MS (2008). Efeitos antinociceptivo e antiinflamatório do extrato etanólico das folhas de Duguetia lanceolata St. Hil. (Annonaceae). Lat. Am. J. Pharm. 27:398-402.
|
|
Sousa OV, Soares Júnior DT, Del-Vechio G, Mattosinhos RG, Gattass CR, Kaplan MAC (2004). Atividades antinociceptiva e antiinflamatória do óleo essencial de cascas de Duguetia lanceolata St. Hil., Annonaceae. Rev. Bras. Farmacogn. 14:11-14.
|
|
Teslim OA, Vyvienne M'K, Olatokunbo OM, Oluwafisayo AJ, Mlenzana NB, Shamila M, Nesto T, Grace M (2014). Side effects of non-steroidal anti-inflammatory drugs: The experience of patients with musculoskeletal disorders. Am. J. Health Res. 2:106-212.
Crossref
|
|
Tjolsen A, Berge OG, Hunskaar S, Rosland JH, Hole K (1992). The formalin test: An evaluation of the method. Pain 51:5-17.
Crossref
|
|
Vinegar R, Truax JF, Selph JL (1973). Some quantitative temporal characteristics of carrageenin-induced pleurisy in the rat. Proc. Soc. Exp. Biol. Med. 143:711-714.
Crossref
|
|
Wei A, Shibamoto T (2010). Antioxidant/lipoxygenase inhibitory activities and chemical compositions of selected essential oils. J. Agric. Food Chem. 58:7218-7225.
Crossref
|
|
Winter CA, Risley EA, Nuss GW (1962). Carrageenin-induced edema in hind paw of the rat as an assay for antiinflammatory drugs. Proc. Soc. Exp. Biol. Med. 111:544-547.
Crossref
|
|
Young JM, Spires DA, Bedord CJ, Wagner B, Ballron SJ, De Young LM (1984). The mouse ear inflammatory response to topical arachidonic acid. J. Investig. Derm. 82:367-371.
Crossref
|