ABSTRACT
This work proposes NADP-ME activity as bio-indices for herbicidal sensitivity in Azolla pinnata R.Br. under 2,4-D toxicity. Azolla fronds were illuminated under saturated photosynthetic flux and bicarbonate induction. There was significant variation in the NADP-ME activity under light and dark conditions. This increased more when varying concentrations of 2,4-D were used for the up regulation of enzyme activity especially under illumination. There was evident modulation through activators (citrate and succinate) and inhibitors (pyruvate and oxaloacetate) under light and dark conditions at 2,4-D concentration. This occurs at the same time in overriding the herbicidal stress linked to the adjustment of the cellular redox of regulatory sites using dithiothreitol. This shows that Azolla can quench 2,4-D, which suggests NADP-ME as a biomarker through its sensitivity and mode of activities under xenobiotic contaminated soil.
Key words: NADP-malic enzyme, aquatic fern, herbicide, modulators, malate.
Abbreviation:
2,4-D, 2,4-Dichloro phenoxy-acetic acid; NADP-ME, NADP linked Malic Enzyme; ROS, Reactive Oxygen Species; RH, Relative Humidity; MS Media, Murashige and Skoog media; MgCl2, Magnesium Chloride; PMSF, Phenylmethylsulfonyl fluoride; EDTA, Ethylene diamine tetraacetic acid; DTT, Di-thio threitol.
There are few key regulatory enzymes in carbon reduction pathways of plants where both concentrations of cellular metabolites as well as physico-chemical factors are responsible for regulation. NADP-malic enzyme (NADP-ME: L-Malate; NADP-oxido-reductase [oxaloacetate de-carboxylating]) is a oxidative decarboxylating enzyme regardless of C3 and C4 plants that regenerate CO2 at the expense of NADP (Xu, et al., 2013). NADP-ME [EC-1.1.1.40] is most crucial for photosynthesis in respect to re-fixation of CO2 from oxidative decarboxylation of malate with generation of NAD(P)H+H+. Moreover, malate, the substrate for NADP-ME, is the influencing factor of photosynthetic flux through ratio of NADP+ to NAD(P)H+H+. The redox potential of tissue by NADP+: NAD(P)H+H+ is now a crucial factor for different abiotic stress responses, and thus NADP-ME serves as an index for stress sensitivity (Wang et al., 2017). The activity of NADP-ME is under the control of few cellular inputs like high pH (≥8.0), high CO2, malate and HCO3- concentration in the chloroplast. This is the chloroplastic light regulatory protein indexed in oxidative stress (Turkan et al., 2018). Any fluctuation of abiotic inputs may be a factor to determine the optimum activity of this enzyme. Environmental stresses changing the cellular redox may be marked by NADP-ME activities and its deviation from controlled conditions. Thus, NADP-ME also serves as a redox indicator under changing condition of photo oxidation, photorespiratory condition of high light intensities and low CO2:O2 ratio (Kustka et al., 2014).
Photo oxidation is accomplished by few abiotic stressors in the form of toxic chemical residues, predominantly sourced by industrial effluents. Those chemicals may be artificial and hazardous to environment distributed through biological system. Overall, these are called xenobiotics, and in the context of pollutants, dioxins and polychlorinated biphenyl derivatives are emphasized (Neilson, 2017). Out of the agricultural pollutants, herbicides are the predominant ones included with xenobiotics. Non-target plant species are more vulnerable to herbicides through the residual effect of 2,4-D in soil. Herbicide has been more frequently reported in different strategies to de-contaminate the soil after weeding (Gill and Garg, 2014). A systematic herbicide is also realised through its residual in soil after its absorption by roots and its downstream effects in plants (Foy, 2018). Waste water, effluents from industry and run-off from agricultural fallows, and herbicidal residues contaminate surface water. Effluents from waste water treatment plant containing 80% herbicides could greatly contaminate surface water (Tournebize et al., 2017). In aquatic ecosystem marshy plants predominantly interact with herbicidal toxins in relation to their hyperaccumulation and sustenance. Regarding herbicidal stress, photo oxidation is the determining factor for sustainability in plants (Anjum et al., 2011). With this, the relevance of NADP-ME may be questioned as a regulatory index for tolerance to herbicides. Few aquatic macrophytes including some non-flowering plants like Salvinia, Marsilea, and Azolla were also reported for their hyper-accumulating nature (Das et al., 2013; Dhir, 2018). Azolla, an aquatic fern, is more established with its inherent nitrogen fixing ability to contribute to soil fertility. In addition, it can greatly prevent herbicides from contaminating water bodies. Based on this, we hypothesize that Azolla may be a bio-indicator species for detecting 2,4-D toxicity through the modulation of NADP-ME activities. Therefore, NADP-ME is used as the bio-index for the changes that occur in cellular redox under 2,4-D toxicity. Thus, in the present experiment, the NADP-ME activity under 2,4-D toxicity is discussed in Azolla and its modulation under light and darkness that may open up the regulatory mode of the enzyme.
Plant material and experimental conditions
Azolla pinnata R.Br., a free floating aquatic fern, was collected from the fresh water pond in the university campus for the present experiment. Initially, plants were transferred to cemented tank filled with tap water for further use. Thereafter, plants were transferred to a nutrient solution containing ¼th MS media for acclimatization (Murashige and Skoog, 1962). Thereafter, the plants were supplemented with varying concentrations of 2,4-D: 100, 250, 500 and 1000 µM for 7 days in growth chamber at 35 ± 1°C, 85% RH and 14/10 h (L/D).
Biochemical analysis
After 7 days of treatment the plants were harvested and separated into root and fronds and stored at -80ËšC. Later, they were used for different biochemical assays.
NADP-ME activity
NADP-ME activity was determined according to Drincovich et al. (2001). Discs of Azolla fronds were incubated on 20 ml of 2 mM NaHCO3- in Petri dish. They were illuminated within saturated light at an intensity of 900 –1000 µEm-2s-1. After 30 min of illumination and dark incubation, the tissues were extracted in a prechilled mortar and pestle with 1.5 ml of extraction buffer containing 100 mM Tris HCl (pH=7.5), 10 mM MgCl2, 2 mM EDTA, 10 mM PMSF, 10% (v/v) glycerol, 20% PVP and 10 mM β-ME. The extract was centrifuged at 15000×g for 15 min. The supernatant was used for further enzymatic assay. The enzyme activity was assayed spectrophotometrically at 340 nm by measuring the NADPH produced with 3 ml of assay mixture containing 100 mM Tris-HCl (pH=7.5), 10 mM MgCl2, 0.5 mM NADP+ and enzyme extract. The reaction started after adding 0.01 mM malate (limiting) and 4 mM malate (saturating) concentrations.
Influence of NADP-ME activity under different modulators:
For the determination of regulatory activities of enzyme both from illuminated and dark-adapted fronds under 0.01 and 4 mM malate concentrations, two activators: 2 mM citrate and 2 mM succinate and two inhibitors: 10 mM pyruvate and 0.5 mM oxaloacetate were used for the present experiment, according to Murmu et al. (2003). The extracted and purified protein was used to determine the effect of activators and inhibitors. 3 ml of assay mixture was read at 340 nm to assay the enzyme activity as described earlier.
Regulation of light induction by DTT
The light induced activation of many photosynthetic enzymes is modulated by the reduction of dithiols on the active site residue. In the present experiment the enzyme extracted from the illuminated and dark incubated fronds were assayed by adding and withdrawing 10 mM DTT along with other assay buffer mixture as mentioned above. The reaction started after adding 0.01 mM and 4 mM malate concentrations. The enzyme activity was assayed spectrophotometrically at 340 nm by measuring the NADPH produced, according to Murmu et al. (2003).
Statistical analysis
All the data were recorded with three replications (n=3) and data were expressed as mean ± SE. The statistical analysis was performed by one-way (ANOVA) followed by least significance difference (LSD) test taking p≤0.05 levels of significance (Gomez and Gomez, 1984). Windows Microsoft Excel 2007 software was employed for computation and data analysis.
Response pattern of light and darkness on NADP-ME activities
The optimum activities of NADP-ME in frond tissues of A. pinnata R.Br. were standardised upon illumination around the saturation of PAR (900 – 1000 µE m-2s-1) in a solution of 2 mM HCO3-. From the two sets of experiment, the observations were made either in illuminated or non-illuminated conditions. The assay mixture was pre-incubated in alkaline medium (pH=7.5- 8.0) under saturated 4 mM and limiting 0.01 mM malate. From both the tissue extract containing partially purified protein recorded an optimum activity (µM/mg FW/h) while being incubated under illumination and darkness for 30 min. The dark-adapted tissues were almost non-responding under the concentrations of 2,4-D; however, a significant discrepancy was recorded compared to that under light. This undoubtedly proves the efficacy of light under constant HCO3- concentration as against dark. The optimum activity was not significantly (p≤0.05) different as compared to control as the plants proceed through 2,4-D concentrations (except at initial concentration). In comparison to control (0 µM 2,4-D), the values of activity were curtailed by 33.46 and 47.8% under 100 µM 2,4-D at 0.01 mM and 4 mM malate respectively. However, the peak activity was recorded at 1000 µM 2,4-D by 1.12 and 2.104 fold as compared to control at 4 mM and 0.01 mM malate concentration respectively (Figure 1).
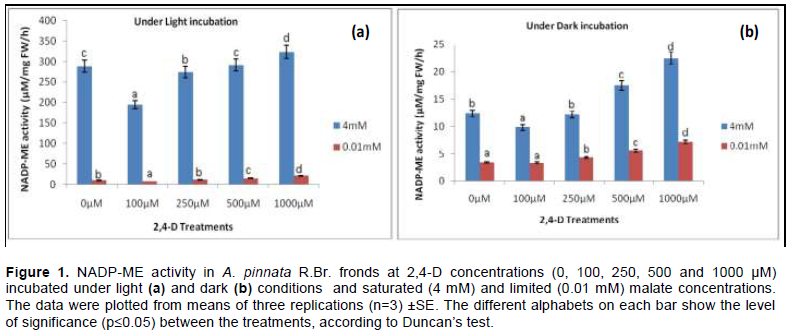
NADP-ME activity and its kinetics under chemical modulators
NADP-ME increases both at transcripts and protein level when tissues vary with irradiance gradient. The pre-incubation in HCO3- solution for the optimum enzyme activity was earlier standardised at 2 mM, according to Raghavendra (2000). Contextually, the light activation of NADP-ME through HCO3- conceives the effects of activators/ inhibitors as recorded in the present experiment. Likewise, two sets of activators (2 mM citrate and 2 mM succinate) and inhibitors (10 mM pyruvate and 0.5 mM oxaloacetate) were applied. For convenience of experimental analysis, the maximum concentration of herbicide (1000 µM) as tolerated by Azolla plants was the standard compared to control (0µM) when it interacts with both the effectors (activators and inhibitors) under malate concentrations.
Regardless of malate concentrations, both activators and inhibitors recorded significant variations in NADP-ME activity under light and dark conditions. For limiting malate concentrations (0.01 mM), the effects of activators (citrate and succinate) were maximised more in light than in the dark. Thus, plants from herbicide treatment were more induced by citrate in light by 1.41 and 1.12 fold over darkness at 0 µM and 1000 µM respectively. Another activator, succinate had similar trend but not significant (p≤0.05) due to the effect of light and darkness. In brief, the activators had modulated the NADP-ME activities under herbicidal toxicity through the effects of light and darkness at the ratios of 1.18 and 1.03 (Figure 3). For inhibitory activity pyruvate and oxaloacetate were chosen; they had similar trend in their mode under light and darkness, but no significant variation. Still, the reduction of activity under herbicide was 42.11% less in darkness than light. The maximum inhibition was done by oxaloacetate at 42.6% under dark condition compared to light under same malate concentration.
On the contrary, at saturated malate concentration the effect of irradiance is more pronounced for both activators and inhibitors. On average, citrate and succinate increased the enzyme activity by 1.18 fold and 1.03 fold more under light than darkness (Figure 2). Pyruvate and oxaloacetate also inhibited the activities when compared at 1000 µM 2,4-D toxicity against light and dark treatments. Pyruvate minimized more the activity by 42.11% more in darkness than under light (significant at p≤0.05); whereas, for oxaloacetate it was 42.59% in the same condition.
Reversal of light induced changes by redox system
Most of the Calvin cycle enzymes are photo regulatory and are based on changes of redox through di-sulphide (S-S) residues of the amino acids in active site. NADP-ME is no exception and could be modulated by light induced reduction of S-S. In the present experiment, this was also monitored with the partial purified protein incubated with 10 mM DTT under 0.01 and 4 mM malate when frond was immerged with 2 mM HCO3- in darkness/illumination. DTT had a significant role regardless of malate concentrations under darkness/light through herbicidal concentration. Thus, the L/D value under DTT presence recorded 0.59 at 0 µM 2,4-D which changes to 0.52 at 1000 µM 2,4-D when assay was done with limiting malate concentration. On the other hand, at saturated malate concentration the presence of DTT recorded a L/D value of 0.71 at 0 µM 2,4-D against 1000 µM 2,4-D by 0.74 L/D value. In the withdrawal or absence and presence of DTT under both concentrations of malate, the changes of L/D values are 1.38, 1.21 and 0.99, 0.92 under 0µM and 1000 µM 2,4-D respectively (Figure 4).
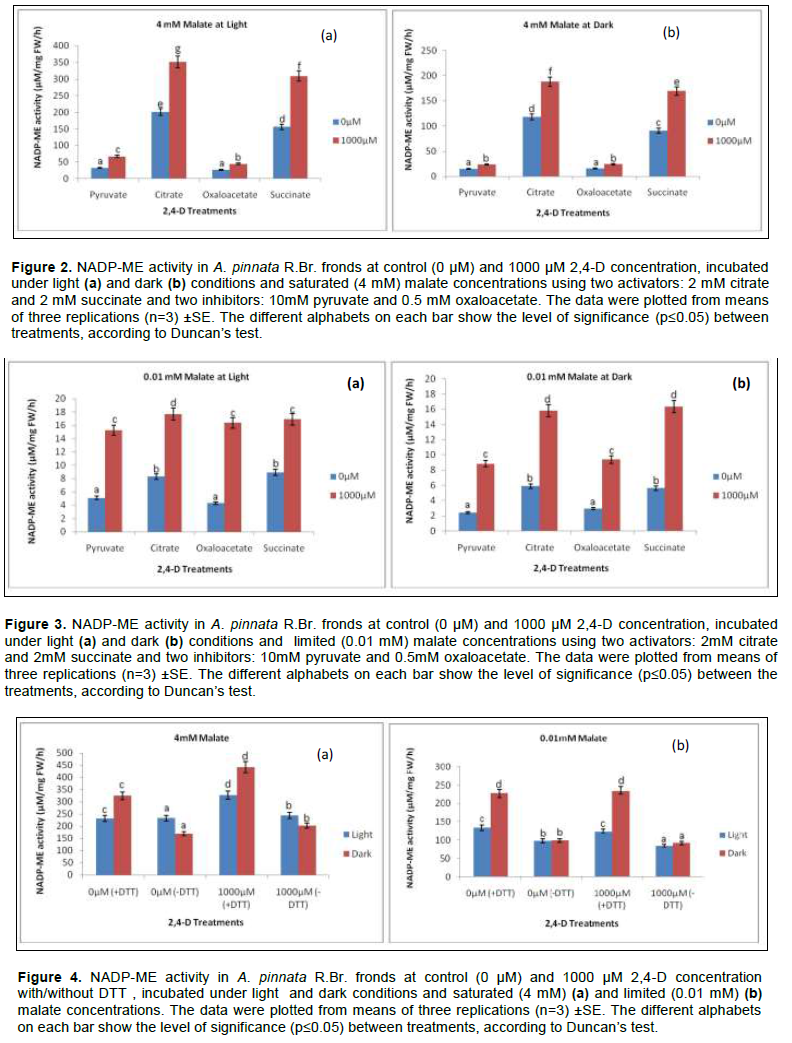
This paper highlights photosynthetic metabolism and its monitoring in herbicidal toxicity as biomarker. From the introduction it appears that photosynthetic enzyme, NADP-ME activity may be taken as redox indicator (Yamori et al., 2018). Therefore, under changing 2,4-D concentrations that disturb the cellular redox may accumulate some bio molecules inducing oxidative stress (Biswal et al., 2011). In our earlier studies Azolla has been reported as a hyperaccumulater of 2,4-D and can quench residual herbicides by antioxidation (De et al., 2017). Herbicide, in different forms, is a serious bottleneck to fertility and disturbs soil micro flora (Wani et al., 2012). In our earlier reports 2,4-D can be hyperaccumulated and transformed chemically into less toxic moiety in Azolla species (De et al., 2017). Therefore, the responses of this species to gene expression would manifest the possible bio indication of herbicidal toxicity. Thus, an initial change of NADP-ME under 2,4-D treatments regardless of light/darkness, activator/inhibitor may suggest its cellular responses under changing redox as said earlier to C4 and C3 flowering species. Now in the present experiment, the enzyme activity was evaluated with different conditions and chemicals in Azolla. 2 mM HCO3- concentration may induce NADP-ME for optimum activity at 4mM malate than 0.01mM. High concentration of CO2 in the bundle sheath is required to continue the photosynthesis even with minimum CO2 compensation point (Bellasio and Griffiths, 2013). This establishes it as an attributing factor for light activation of NADP-ME and that is also under 2,4-D toxicity in Azolla. In comparison to control (0 µM 2,4-D), the activity was improved based on the ongoing concentrations of herbicide. This may be attributed to high CO2 concentration in the tissues. It also supports the tolerance of 2,4-D in Azolla with relation to sustained photosynthesis through malate metabolism. In Azolla, a C3 species under the hyperaccumulation of 2,4-D, the photosynthetic activity remains less altered through anaplerotic reactions of organic acids. Malate and its inter-conversion is the most possible source of CO2 in other downstream reactions (Ewe et al., 2018). Expectedly, in Azolla any loss of metabolites would be compensated by an over-riding malate accumulation even under excessive accumulation of herbicides. In higher plants during C4 photosynthesis, decarboxylation reactions lead to high concentration of CO2 for RuBP-case activity and thereby photo respiratory loss is minimized (MacAdam and Nelson, 2017). It is assumed that Azolla under varying 2,4-D concentrations is compensated for photo respiratory CO2 loss with NADP-ME overexpresion. 2,4-D can alter the carboxylating machinery and release CO2 inducing photo respiratory flux.
The photo-activation may not be simple under the influence of light and darkness; yet the responses of Azolla to pyruvate (as inhibitor) to decrease NADP-ME activity is interesting. The presence of pyruvate increasing the activity (under illumination) favours the binding to regulatory domain of the enzyme. Similarly, it happens also for oxaloacetate and malate concentration depending on the feedback inhibition of decarboxylation reactions (Tissier, 2018). Any way, 2,4-D may induce more pyruvate conversion into oxaloacetate releasing CO2 and thus inhibition results. The supply of CO2 in the form of HCO3- could be another source to enrich the CO2 over its limiting concentration (Sage, 2013). The influence of HCO3- may also be useful to adjust the higher pH of mesophyll cell to tolerate stress. Through photo respiratory flux, especially under high irradiance (near the saturated photo oxidative condition) cytosol becomes alkaline by decarboxylation reactions. Tolerance to herbicides and other toxic compounds may also be attributed by a higher pH of salt accumulation (Powles, 2018). This possibly could be adjusted, however, partially through over -expression of NADP-ME activity by decarboxylating reactions releasing CO2. Therefore, NADP-ME could be hypothesized as an effective biomarker (yet to be established by isozymes from different plant tissues). Studies have shown that higher angiospermic plants are more flexible to reach optimum activities at 2 mM HCO3-, while Azolla needs a higher concentration. This is because it can tolerate higher pH and stress (Pandey et al., 2013). Azolla, a C3 plant is prone to photo respiratory flux and thus involves in photo-oxidative damages of bio molecules. Despite this, few aquatic plants are still tolerant to photo-oxidative condition due to metal acquisition and being hyperaccumulators of xenobiotics (Gautam, 2017).
The light regulation of Calvin cycle enzymes in higher plants, Azolla also added some information regarding its modulation under 2,4-D concentration. From our results the reduction by DTT to the oxidized thiol (-S-S-) at regulatory site might lead to activation of enzyme. Under dark condition the inactivation is realized with redox mediated thiol oxidation of Calvin cycle enzymes (Mock and Dietz, 2016). It is interesting to note that Azolla at the pattern of 2,4-D inactivation, particularly, at higher concentrations was quite consistent and compatible with those of angiospermic species. This predicts that the target sites are the thiol ligands for 2,4-D mediated de-activation of NADP-ME (under substrate saturation condition). Therefore, Azolla which has already been recognised as hyper-accumulator species for toxic metalloids might be a good indicator for such herbicidal toxicity. It is quite clear that the regulatory mechanism for Calvin cycle enzymes by changes of thiol-redox is compatible irrespective of plant species (including non-flowering species). This also attenuates a change in cellular redox in down regulation of photosynthetic activity through decarboxylation reactions as illustrated with NADP-ME. So, cellular redox with its oxidised/reduced state would complement the NADP-ME activity in-vitro as a possible bio-marker under herbicidal stress. Azolla, an aquatic fern has served these findings of such conception in the present experiment.
Azolla culture system undoubtedly has an input in nitrogen supplementation in field. Herbicidal toxicity as residual effect in soil is amplified through food chain. In bioremediation mode, a plant species would be an option to quench that much herbicide from soil as well as to detect its presence. The latter is recognized as bio monitoring with some precise cellular responses. NADP-ME activity is evident as a biomarker for herbicidal toxicity expressed through Azolla system. This has given more details with its regulation under environmental factors like light and darkness. Along with practical implication, the Azolla plants have also revealed the insights for cellular regulation of NADP-ME through biochemical modulation. Therefore, this study would be thought-prone to predict NADP-ME as a reliable bio-indices for 2,4-D toxicity in soil.
The authors have not declared any conflict of interests.
The authors are thankful to the Department of Science and Technology, Govt. of India, New Delhi (DST-PURSE programme) for the financial support.
2,4-D, 2,4-Dichloro phenoxy-acetic acid; NADP-ME, NADP linked Malic Enzyme; ROS, Reactive Oxygen Species; RH, Relative Humidity; MS Media, Murashige and Skoog media; MgCl2, Magnesium Chloride; PMSF, Phenylmethylsulfonyl fluoride; EDTA, Ethylene diamine tetraacetic acid; DTT, Di-thio threitol.
REFERENCES
Anjum SA, Xie XY, Wang LC, Saleem MF, Man C, Lei W (2011). Morphological, physiological and biochemical responses of plants to drought stress. African Journal of Agricultural Research 6(9):2026-2032.
|
|
Bellasio C, Griffiths H (2013). The operation of two decarboxylases (NADPME and PEPCK), transamination and partitioning of C4 metabolic processes between mesophylll and bundle sheath cells allows light capture to be balanced for the maize C4 pathway. Plant Physiology P 113.
|
|
|
Biswal B, Joshi PN, Raval MK, Biswal UC (2011). Photosynthesis, a global sensor of environmental stress in green plants: stress signalling and adaptation. Current Science pp. 47-56.
|
|
|
Das K, Mandal C, Ghosh N, Dey N, Adak MK (2013). Cadmium accumulation in Marsilea minuta Linn. and its antioxidative responses. American Journal of Plant Sciences 4(02):365.
Crossref
|
|
|
De AK, Sarkar B, Adak MK (2017). Physiological explanation of herbicide tolerance in Azolla pinnata R. Br. Annals of Agrarian Science 15(3):402-409.
Crossref
|
|
|
Dhir B (2018). Role of Ferns in Environmental Cleanup. In Current Advances in Fern Research pp. 517-531.
Crossref
|
|
|
Drincovich MF, Casati P, Andreo CS (2001). NADPâ€malic enzyme from plants: a ubiquitous enzyme involved in different metabolic pathways. FEBS letters 490(1-2):1-6.
Crossref
|
|
|
Ewe D, Tachibana M, Kikutani S, Gruber A, Bártulos CR, Konert G, Kroth PG (2018). The intracellular distribution of inorganic carbon fixing enzymes does not support the presence of a C4 pathway in the diatom Phaeodactylum tricornutum. Photosynthesis Research pp. 1-18.
Crossref
|
|
|
Foy CL (2018). Adjuvants: terminology, classification, and mode of action. In Adjuvants and agrochemicals CRC Press pp. 1-15.
Crossref
|
|
|
Gautam A (2017). Phytoremediation of Arsenate, Zinc and Cadmium with the help of Ceratophyllum demersum L (Doctoral dissertation, Department of Molecular and cellular Engineering Jacob Institute of Biotechnology & Bioengineering Sam Higginbottom Institute of Agriculture, Technology and Sciences).
|
|
|
Gill HK, Garg H (2014). Pesticides: environmental impacts and management strategies. InPesticides-Toxic Aspects. In Tech. DOI: 10.5772/57399
Crossref
|
|
|
Gomez KA, Gomez AA (1984). Statistical Procedures for Agricultural Research. Wiley, New York. 704 p.
|
|
|
Kustka AB, Milligan AJ, Zheng H, New AM, Gates C, Bidle KD, Reinfelder JR (2014). Low CO2 results in a rearrangement of carbon metabolism to support C4 photosynthetic carbon assimilation in Thalassiosira pseudonana. New Phytologist 204(3):507-20.
Crossref
|
|
|
MacAdam JW, Nelson CJ (2017). Physiology of forage plants. Forages, An Introduction to Grassland Agriculture 1(13):1-51.
|
|
|
Mock HP, Dietz KJ (2016). Redox proteomics for the assessment of redox-related posttranslational regulation in plants. Biochimica et Biophysica Acta (BBA)-Proteins and Proteomics 1864(8):967-73.
Crossref
|
|
|
Murashige T, Skoog F (1962). A revised medium for rapid growth and bioassays with tobacco tissue cultures. Physiologia Plantarum 15:473-497.
Crossref
|
|
|
Murmu J, Chinthapalli B, Raghavendra AS (2003). Light activation of NADP malic enzyme in leaves of maize: marginal increase in activity, but marked change in regulatory properties of enzyme. Journal of Plant physiology 160(1):51-6.
Crossref
|
|
|
Neilson AH (2017). Organic chemicals in the aquatic environment: distribution, persistence, and toxicity. CRC Press.
|
|
|
Pandey DN, Gopal B, Sharma KC (2013). Evidence-Based Holistic Restoration of Lake Anasagar, Ajmer, Rajasthan, India.
|
|
|
Powles SB (2018). Herbicide resistance in plants: biology and biochemistry. CRC Press.
Crossref
|
|
|
Raghavendra AS (editor) (2000). Photosynthesis: a comprehensive treatise. Cambridge University Press.
|
|
|
Sage RF (2013). Photorespiratory compensation: a driver for biological diversity. Plant Biology 15(4):624-638.
Crossref
|
|
|
Tissier A (2018). Plant secretory structures: more than just reaction bags. Current Opinion in Biotechnology 49:73-79.
Crossref
|
|
|
Tournebize J, Chaumont C, Mander Ü (2017). Implications for constructed wetlands to mitigate nitrate and pesticide pollution in agricultural drained watersheds. Ecological Engineering 103:415-425.
Crossref
|
|
|
Turkan I, Uzilday B, Dietz KJ, Bräutigam A, Ozgur R (2018). Reactive oxygen species and redox regulation in mesophyll and bundle sheath cells of C4 plants. Journal of Experimental Botany 69(14):3321-3331.
Crossref
|
|
|
Wang Y, Stevanato P, Yu L, Zhao H, Sun X, Sun F, Li J, Geng G (2017). The physiological and metabolic changes in sugar beet seedlings under different levels of salt stress. Journal of Plant Research 130(6):1079-93.
Crossref
|
|
|
Wani SH, Sanghera GS, Athokpam H, Nongmaithem J, Nongthongbam R, Naorem BS, Athokpam HS (2012). Phytoremediation: Curing soil problems with crops. African Journal of Agricultural Research 7(28):3991-4002.
|
|
|
Xu J, Zhang X, Ye N, Zheng Z, Mou S, Dong M, Xu D, Miao J (2013). Activities of principal photosynthetic enzymes in green macroalga Ulva linza: functional implication of C 4 pathway in CO 2 assimilation. Science China Life Sciences 56(6):571-80.
Crossref
|
|
|
Yamori W, Hikosaka K, Way DA (2014). Temperature response of photosynthesis in C3, C4, and CAM plants: temperature acclimation and temperature adaptation. Photosynthesis Research 119(1-2):101-117.
Crossref
|
|