ABSTRACT
Cowpeas (Vigna unguiculata) are important grain legumes grown by resource poor farmers across Sub-Saharan Africa. In Kenya, cowpeas continue to produce low yields due to erratic rainfall caused by the current climate change. This study sought to enhance drought tolerance in Kenyan cowpeas through transformation by Agrobacterium tumefaciens using L-Pyrroline-5-carboxylate synthase (P5CS) gene. Cowpea variety K80 was inoculated with Agrobacterium tumefaciens strains EHA105 and LBA4404 harbouring recombinant plasmids pCAMBIA1301:VuP5CS or pCIP100:VuP5CS through vacuum infiltration of pre-germinated seeds at 60 kpa for 25 min. The presence of the transgene in the transformed cowpea plants and its transfer to progeny was confirmed by PCR analysis of T0 and T1 plants. The highest transformation efficiency of 20.5% was achieved with strain EHA105 harbouring plasmid pCAMBIA1301:VuP5CS. A segregation analysis of the transgenes gave a 1:4 ratio for the VuP5CS transformed: non-transformed plants and did not follow the 3:1 Mendelian inheritance pattern for dominant genes. There was no difference in proline content in the transformed and non-transformed T1 plants. However, the roots of the transformed plants were significantly longer than those of the non-transformed plants. The numbers of harvested seeds were also significantly higher in the transformed plants with 10 to 11 seeds per pod in comparison to the non-transformed plants with an average of 5.58 seeds per pod, indicating drought tolerance potential of the transformed plants. The T2 and T3 plants need to be screened further to evaluate the stable integration of the transgene and physiological characterization under water stress.
Key words: Vigna unguiculata, in planta transformation, Agrobacterium tumefaciens, VuP5CS (Vigna unguiculata pyrroline-5-carboxylate synthase).
Cowpeas are an important grain legume grown widely in Kenya with the bulk of the production being from the rural poor. It has high nutritional value with crude protein comprising about 25% of the seed dry weight (Tshovhote et al., 2003), and leaves which are harvested when young, are used as vegetables rich in vitamin B and folic acid (
Ogunmodede and
Oyenuga, 1970). Cowpeas are also used as fodder for livestock and are a good rotation crop. Apart from its nutritional value, cowpeas act as a source of income for several rural households who sell their surplus produce in the market. According to Singh
et al. (2003), the haulms of cowpeas are dried on roof tops and sold as fodder in the dry season, fetching between 50-80% of the seed price on dry weight, thus, providing a good source of income. As a cover crop, cowpeas help in suppressing weeds and also being a leguminous crop, that helps in fixing nitrogen in the soil. It therefore acts as a soil conditioner, improving soil fertility. Most importantly, cowpeas have the ability to tolerate poor soil conditions (Popelka et al., 2004).
Africa produces 92% of cowpeas globally, where approximately 200 million people consume it on a daily basis (Popelka et al., 2004). Nigeria is the leading producer and consumer of cowpeas worldwide, with an estimated annual production of 2.4 million tons grown on 5 million hectares, followed by Niger, Brazil and USA (USAID, 2010). Cowpea productivity in Kenya has been extremely low, with yields ranging between 102 to 239 Kg/Ha, which is far less than the potential yields of 1200 to 1800 Kg/Ha according to a study by Kimiti et al. (2009). The low yields were attributed to poor soil fertility, weeds such as the parasitic Striga gesnerioides and Alectra vogelii, inadequate farm inputs and long dry spells which was experienced by 96% of households interviewed. The Kenya Agricultural and Livestock Research Organization (KALRO) has done a lot of research on cowpeas focusing on disease and insect pests (Karanja et al., 2012) to reduce loss of produce. Most breeding programs have focused on increasing crop yields which may counteract the conservative strategies acquired through evolution to protect plants against water stress which is found in their wild relatives (Tardieu, 2012).
Although, cowpeas are known to be drought tolerant, Fatokun et al. (2009) showed that there was variation of drought tolerance among germplasm lines. The International Institute of Tropical Agriculture (IITA) and the Institut Senegalais de Recherches Agricoles (ISRA) have conventionally bred early maturing high yielding cowpea cultivars (IT84S-2246, Bambey 21) to escape drought, and these have been widely adopted by farmers. These varieties however performed poorly when exposed to drought during the reproductive stages (Agbicodo et al., 2009). According to Shinozaki and Shinozaki (1997), a number of genes induced during water stress produce important metabolic proteins to protect cells from water deficit as well as regulate genes for signal transduction in response to water stress. These gene products are classified in two groups, functional and regulatory proteins. Proline is one of the functional proteins in plants involved in water stress tolerance and cellular adaptation (Shinozaki and Shinozaki, 1997).
In several higher plants including cowpeas, proline has been found to accumulate in reproductive organs and tissues during drought, suggesting its role in providing energy during the reproductive phase (Bates et al., 1973; Szabados and Savoure´, 2009; Gazendam and Oelofse, 2007). This is due to the catabolism of proline which occurs in the mitochondria, and is connected to oxidative respiration providing energy for plants to resume growth after stress. Proline is also an important regulator of cellular reactive oxygen species (ROS) balance, influencing programmed cell death (Szabados and Savoure´, 2009), and protects plant cells from damage due to oxidative stress (Gill and Tuteja, 2010). Thus, one option of enhancing proline biosynthesis so as to improve tolerance to drought is by increasing expression of rate-limiting genes in transgenic plants (Szabados and Savoure´ 2009). The rate limiting catalyst in the proline biosynthetic pathway is pyrroline-5-carboxylate synthase (P5CS) which uses glutamate as a substrate (Kishor et al., 2005). Based on the fact that cultivated cowpea varieties are self-pollinated, the cowpea genome therefore has a narrow gene pool. The application of plant transformation can thus be useful, in that only the gene of interest is transferred to the plant, while other desirable traits are maintained.
There are reports on tissue culture and regeneration of cowpeas (Raveendar et al., 2009; Bakshi et al., 2011) as well as transformation (Solleti et al., 2008; Higgins et al., 2013). Agrobacterium mediated transformation has been used in the recent years to deliver crop varieties with insect pest resistance, herbicide resistance, bio-fortified crops among others. Agrobacterium-mediated transformation has been preferred as a gene delivery method over biolistics in plant transformation as it is cheaper and results in higher transformation efficiencies. Complex gene integration patterns as well as insertion of multiple copies with biolistics makes it less favourable in plant transformation as it could lead to gene silencing (Kikkert et al., 2005). In cowpeas, transformation has been achieved through inoculation with Agrobacterium suspension of embryo axis (Adesoye et al., 2010), cotyledonary nodes (Bakshi et al., 2011; Higgins et al., 2013) with average transformation efficiencies ranging between 0.1 to 3.9%. Higgins et al. (2013) successfully transformed West African cowpea varieties with the Bacillus thuringiensis (Bt.) insect resistant gene to protect against pod borers. Other cowpea genotypes which have been successful in tissue culture and transformation studies include Pusa komal (Bakshi et al., 2011), Cheng-jiang VII (Tang et al., 2012), IITA cultivars and Nigerian landraces (Ilori and Pellegrineschi 2011). However, there has been no documented attempt in the improvement of Kenyan cowpea varieties through transformation.
Low transformation efficiency is characteristic in grain legumes including cowpeas. Higgins et al. (2013) achieved a transformation efficiency of 0.3% when cotyledonary node explants were transformed with a plasmid containing the herbicide resistance bar gene, while Popelka et al. (2006) sachieved a transformation efficiency of 0.1% with one fertile plant in a thousand explants transmitting the bar gene, encoding resistance to phosphinothricin, to their progeny. Bakshi et al. (2011) achieved 3% transformation efficiency of cowpea cotyledonary node explants transformed with the nptII selectable marker gene using vacuum infiltration and sonication with transformed explants selected on medium containing kanamycin. In the transformation of Vigna mungo, a close relative of cowpeas, Saini et al. (2003) only achieved a transformation frequency of 1% when they transformed cotyledonary nodes with plasmid pCAMBIA2301.
This study sought to enhance drought tolerance in Kenyan cowpeas through Agrobacterium mediated transformation with the VuP5CS gene. According to Mao et al. (2006), although several cowpea cultivars had been explored for their shoot organogenesis potential, the studies indicated that the regeneration of cowpeas in vitro was genotype specific, requiring the optimization of tissue culture conditions for each individual genotype. To address this challenge, the in planta cowpea seed transformation was considered as an alternative to omit the tissue culture stage of regenerating whole plants. The VuP5CS gene was selected for this study as it naturally occurs in cowpea plants and would therefore be more acceptable to farmers as opposed to introduction of foreign genes which has been battled in the production of other transgenic crops in Kenya. This study could aid in achieving cowpea varieties with tolerance to biotic and abiotic stresses hence, delivering higher yield for farmers.
Study location
The study was carried out at the Biosciences Eastern and Central Africa (BecA) – International Livestock Research Institute (ILRI) hub In Nairobi, Kenya with the GPS co-ordinates 1°16'07.5"S 36°43'21.1"E.
Bacterial culture
Agrobacterium culture medium was prepared using low salt Luria Bertani agar (LBA – Duchefa Biochemie BV.) at 30 g/L and autoclaving at 121°C for 20 min. Filter sterilized antibiotics were added to the media after cooling under a micro flow cabinet as follows: 50 mg/L kanamycin, 50 mg/L rifampicin and 100 mg/L streptomycin. The medium was then dispensed into Petri dishes and after solidifying, 1.0 µL of glycerol stocks of pCAMBIA 1301 and pCIP100 retrieved from 80°C freezer was streaked onto the medium. These were sealed with parafilm and incubated at 28 °C for 48 hrs.
Liquid Luria Bertani (LB) nutrient medium was prepared by dissolving 40 g of LB broth (Duchefa Biochemie BV.) in 1.0 L of distilled water and autoclaved. After cooling, 10 mL of the liquid LB medium was measured into 50 mL falcon tubes and the filter sterilized antibiotics kanamycin and rifampicin added at 50 mg/L each. A pipette tip was used to pick a colony of Agrobacterium from the 48 hr culture and dipped into the liquid LB medium contained in the falcon tubes and sealed with parafilms. The tubes were incubated on a shaker overnight at 28°C and a rotor speed of 225 rpm.
Plasmid isolation
Plasmids were isolated from the overnight Agrobacterium cultures using the GeneJet plasmid miniprep kit (Thermo Fisher Scientific Inc.) following the manufacturers’ instructions to confirm the presence of the plasmids pCAMBIA 1301 and pCIP100. The extracted plasmid DNA was quantified using the NanoDropTM 2000 UV-Vis spectrophotometer (Thermo Fischer Scientific Inc.) to check the quantity and quality of the DNA and the nptII, hpt and gusA gene fragments PCR amplified using specific primers (Table 1), to confirm the presence of the selectable marker genes in the plasmids. Using the plasmid map for plasmid pCAMBIA 1301, restriction enzymes NcoI and BstEII were selected to cleave the 2 kb length of the gusA gene between the 35S promoter and the NOS terminator. Restricted plasmid DNA was de-phosphorylated using alkaline phosphatase at 1.0 µL/µg of digested DNA to avoid re-ligation and incubated at 37°C for 30 min followed by heat inactivation at 65°C for 5 min. The VuP5CS gene was PCR amplified using VuP5CS specific primers (Table 1) and purified using GeneJET PCR purification kit according to the manufacturer’s instructions. The purified PCR product was digested with the BstEII and NcoI restriction enzymes and ligated to the restricted and de-phosphorylated vector backbones of pCIP100 and pCAMBIA 1301 in the ratio of 1:3 (vector: insert = 30 ng:90 ng) using T4 DNA ligase and incubated overnight at 4°C.
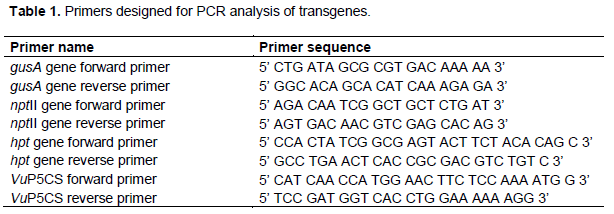
Agrobacterium transformation
Two strains of Agrobacterium tumefaciens, LBA4404 and EHA105 were transformed with the recombinant plasmids pCIP100:VuP5CS and pCAMBIA 1301:VuP5CS through electroporation and cultured in LBA with kanamycin and rifampicin at 50 mg/L each for 48 hrs at 28 °C. For selection of strain LBA4404, 100 mg/L of streptomycin was added to the bacterial selection medium. After 2 days of culture, the colonies which were able to grow were individually picked with a pipette tip and cultured in liquid LB medium with the same antibiotic concentrations. Plasmid DNA was isolated and PCR amplified with VuP5CS, nptII, hpt and gusA specific primers. Plasmid DNA whose samples had positive PCR amplification for the transgenes were digested with NcoI/BstEII, EcoRI and HindIII enzymes to confirm the presence of the VuP5CS gene. The double digest with NcoI and BstEII was expected to give 2 fragments, one which is 9 kb and another of 2.4 kb, the size of the VuP5CS gene. On the other hand, a double digest with EcoRI and HindIII was expected to result in 3 fragments of 8 kb, 2.3 kb and 616 bp (EcoRI) or 333-bp (HindIII) because both enzymes have two recognition sites within the VuP5CS gene sequence.
Agrobacterium cultures exhibiting the presence of the recombinant plasmids pCIP100:VuP5CS and pCAMBIA 1301:VuP5CS were refreshed in an overnight culture with 5 µL cultured in 10 mL of the liquid LB medium in a 50 mL falcon tube with kanamycin and rifampicin added at 50 mg/L each. The tubes were incubated on a shaker overnight at 28°C and a rotor speed of 225 rpm and used for seed transformation.
Inoculation of cowpea seeds
Cowpea in planta seed transformation by vacuum infiltration was modified from De Ronde et al. (2001). Seeds of cowpea variety K80 were surface sterilized with 2 washes using 70% ethanol followed by 1% sodium hypochlorite for 30 min. Sterile seeds were germinated in Petri plates lined with sterile filter paper moistened with sterile distilled water. After 2 days, the seed coat was removed and the seeds vacuum infiltrated for 25 min at 60 kpa with the inoculation medium containing A. tumefaciens cultures grown overnight. The A. tumefaciens cultures strain EHA105 or LBA4404 with plasmids pCAMBIA1301:VuP5CS, pCIP100:VuP5CS, pCAMBIA1301 or pCIP100 were resuspended in liquid co-cultivating medium (LCM) comprising of MSB5 medium supplemented with 30 g/L sucrose made up to 1 L and pH adjusted to 5.8 with no solidifying agents, followed by autoclaving. After cooling, filter sterilized growth regulators BAP and GA3 were added at 0.5 mg/L and 0.25 mg/L respectively, and thiol compounds dithiothreitol (DTT) at 150 mg/L and 0.4 mg/L L-cysteine, with 20 mM 2-morpholinoethanesulphonic acid (MES) to act as a pH buffer. This was supplemented with 200 µM acetosyringone. Inclusion of thiol compounds L-cystein and DTT in the inoculation and co-cultivation medium enhance gene transfer and reduce browning of wounded explants (Popelka et al., 2006; Higgins et al., 2013). Tween 20 (0.02%) was added to the LCM as a surfactant/wetting agent.
Five sets of treatments and control were carried out with the inoculums having different recombinant plasmids in different strains of Agrobacterium grown overnight as follows:
0) Agrobacterium tumefaciens strain LBA4404 with no plasmid as a negative control.
1) Agrobacterium tumefaciens strain LBA4404 with pCAMBIA 1301.
2) Agrobacterium tumefaciens strain LBA4404 with pCAMBIA1301: VuP5CS.
3) Agrobacterium tumefaciens strain EHA105 with pCIP100.
4) Agrobacterium tumefaciens strain EHA105 with pCAMBIA 1301:VuP5CS.
5) Agrobacterium tumefaciens strain EHA105 with pCIP100: VuP5CS
The inoculated pre-germinated seeds (Treatment 0: 27 seeds; Treatment 1: 36 seeds; Treatment 2: 44 seeds; Treatment 3: 38 seeds; and 45 seeds each for Treatments 4 and 5) were left in the inoculums for 2 h and planted in sterile vermiculite. After 3 days, the first set of leaves was visible and one week later the seedlings were transplanted in sterile soil with farmyard manure in the ratio of 1:1. Leaf samples were taken from the second set of trifoliate leaves of plants from treatments 2, 4 and 5 for PCR analysis of transgenes. Samples from treatments 1 and 3 were used for GUS histochemical assay while samples from treatment 0 were used as a negative control.
The potted plants were allowed to set seed in the greenhouse and a few seeds from the PCR positive T0 plants planted to generate T1 plants. A GUS histochemical assay and PCR analysis was done on the T1 plants to analyse the presence of the uidA, nptII or hpt genes.
Analysis of transformed plants
GUS histochemical assay
The GUS histochemical assay was modified from Jefferson et al. (1987). GUS assay buffer solution (1 mL) containing 100 µL x-gluc (5-bromo-4-chloro-3-indolyl-β-D-glucuronic acid), 890 µL Tris-NaCl and 10 µL of 10% triton, was added to the apical leaf in the second set of trifoliate leaves generated from inoculated seeds in 2 mL eppendorf tubes. The tubes were wrapped in aluminium foil and incubated at 37°C for 24 h. The treated leaves were washed with 70% ethanol, and the wash repeated every 24 h for 3 days to wash off the chlorophyll.
A second GUS assay was carried out using whole plants of 2 week old T1 seedlings of seeds from a single pod of a PCR positive T0 plant where seeds were inoculated with A. tumefaciens strain EHA105 with pCIP100 having the gusA reporter gene (treatment 3). Seeds harvested from a T1 plant from treatment 3 were also subjected to the GUS histochemical assay.
Molecular characterization of transformed plants
DNA was extracted from leaf discs of shoots generated from seeds transformed with A. tumefaciens harbouring the plasmids pCIP100, pCAMBIA1301, pCIP100:VuP5CS or PCAMBIA:VuP5CS and non-transformed cowpea plants using the CTAB method modified from Doyle (1987). DNA extraction buffer was prepared with 2% CTAB, 10 mL of 1 M Tris-HCl, 4 mL of 0.5 M EDTA, 28 mL of 5 M NaCl and 38 mL of distilled water. β-mercaptoethanol (2 mL) was added last in a fume chamber to make a total of 100 mL of extraction buffer.
Three week old transformed and non-transformed plants were used with leaf samples taken from the apex of the second set of trifoliate leaves from all treated plants from the 5 treatments. Samples were immediately wrapped in labelled aluminium foil and placed in liquid nitrogen. The samples were ground using autoclaved mortar and pestle and transferred to a 50 mL falcon tube with 12 mL of 2% CTAB extraction buffer (pre-warmed at 65°C) added and vortexed for 10 s. Samples were then incubated at 65°C for 30 min with inverting every 10 min and at room temperature for 10 min. Twelve mL (equal volume to the CTAB extraction buffer used) of isopropanol: chloroform (1:24) was added in a fume chamber and gently mixed for 10 min. The mixtures were centrifuged at 3000 rpm for 10 min and about 12 mL of the aqueous phase transferred to a clean 50 mL falcon tube, and precipitated with 8.4 mL (70% of the volume) of isopropanol, followed by overnight incubation at – 20°C.
DNA was pelleted by centrifugation at 14000 rpm at room temperature for 10 min. The pellet was washed with 1 mL of 70% ethanol in a centrifuge at 13,200 rpm and 4°C for 5 min, followed by an additional spin to remove residual ethanol. The tubes were air dried at room temperature in a clean air flow cabinet for 30 min and the pellet resuspended in 1 mL of 1 M NaCl and incubated for 15 min at 37°C. RNase A (4 µL of 1 mg/mL) was added to the DNA solution and incubated at 37°C for 45 min. This was transferred to clean falcon tubes and 3 mL (3 volumes) of absolute ethanol added to the DNA solution and incubated for 30 min at 20°C to precipitate DNA. The jelly-like DNA precipitate was looped using a pipette tip and transferred to a 2 mL eppendorf tube. The pellet was washed two times with 70% ethanol to remove excess salts and air dried for 30 min in a clean air flow cabinet. The pellet was eluted in 30 µL nuclease free water and DNA concentration quantified using a nanodrop.
Agarose gel electrophoresis
The extracted DNA was subjected to agarose gel electrophoresis to check the integrity of the DNA with 5 µL of DNA loaded onto agarose gel [1% w/v agarose in 0.5 X TBE buffer stained with 2.5 µL GelRedTM (Biotium)] and 6 µL of 100 bp ladder loaded in the first lane. The gel was run at 100 V for 45 min and visualized using a UV transilluminator and the image captured for analysis of the DNA.
Polymerase chain reaction (PCR) analysis.
The nptII, hpt and gusA specific primers were used in screening for the presence of the transgenes in the DNA extracted from transformed and non-transformed plants. A master mix was prepared by adding 0.4 mL each of the nptII, hpt or gusA forward and reverse primers (10 ng/mL) to 18.2 µL of nuclease free water to make a total volume of 19 mL. This was added into the 20 mL reaction Bioneer AccuPower® PCR Premix followed by the addition of 1 mL of extracted DNA. In two separate tubes, 2 µL of plasmid DNA containing the nptII gene (positive control) or water (negative control) were added and the mixtures vortexed for 5 s followed by a 5 s centrifugation.
The PCR amplification was carried out in a thermal cycler with the initial denaturation at 95°C for 5 min; denaturation, annealing and extension at 95°C 58°C and 72°C respectively for 30 s with 35 cycles each; final extension at 72°C for 10 min. The PCR program for amplification of the hpt gene fragment using plant DNA from putative transformed plants was optimized with the annealing and extension time set at 1 min each. For amplification of the nptII fragment, the denaturation and annealing durations were increased to 45 s and 2.5 min for the extension respectively, with an annealing temperature of 62°C.
Measurement of proline levels in cowpea leaves
The ninhydrin test was used in analyzing the quantity of free proline amino acids. When heated with ninhydrin, they form a purple blue coloured Hydantion compound which is measured colorimetrically (Amanullah, 2011). Proline concentration in leaves obtained from T
1 plants of
in planta seed transformation was measured using a modified protocol by Bates et al. (1973) to determine the levels of free proline in leaf tissues. The protocol is based on the formation of red colored formazone by proline with ninhydrin in acidic
medium, which is soluble in organic solvents like toluene.
A standard curve of proline was prepared using a serial dilution of 1 mL of L – proline in 3% sulphosalycylic acid at 1, 10, 50, 100, 150, 200, 250 and 300 µM. Using a fume chamber, 500 µL of each dilution was added to 500 µL of glacial acetic acid and 500 µL ninhydrin reagent (2.5 g Ninhydrin, 60 mL glacial acetic acid and 40 mL 6 M phosphoric acid dissolved at 70°C and stored in the dark for not more than 24 h.) in a 15 mL falcon tube and boiled for 45 min in a water bath and then cooled on ice for 30 min. An equal volume of toluene was added to each sample, vortexed for 1 min and centrifuged at 700 rpm for 5 min. The optical density of the toluene solution was measured at 520 nm using a spectro-photometer. The measurements were used to make a standard curve for proline. Two hundred milligrams of frozen plant
tissue from T
1 plants transformed with plasmids pCIP100:
VuP5CS or pCAMBIA1301:
VuP5CS and non-transformed plants were grinded separately using a micro pestle in an eppendorf tube and homogenized in 2 mL of 3% sulphosalycylic acid. The homogenized leaf samples were centrifuged at 4000 rpm for 5 min and 500 µL of the supernatant treated with 500 µL of ninhydrin reagent and 500 µL of glacial acetic acid. The optical density of the toluene solution was measured at 520 nm and the proline content calculated using the standard curve.
The gradient from the standard curve generated (y = 357.9x + 8.211) was used in calculating the concentration of proline in the measured samples. To calculate the concentration of proline per gram fresh weight, the following formula was applied according to Bates et al. (1973).
[(µM proline x mL toluene / 115.5 µg/µM] / [(g sample) / 2] = µM proline / g of fresh weight material
Where: µM proline is worked out from the optical density using the gradient from the standard curve, mL toluene is the volume of toluene used, 115.5 µg/µM is the molarity of proline, g sample is the weight of the leaf sample used and 2 is the volume of sulphosalicyllic acid used to homogenize the ground leaf samples.
Physiological analysis of transformed plants
After the T1 plants had set seed, watering was withdrawn from both the transformed and non-transformed plants. After 3 weeks of water withdrawal, the pods were harvested and plants carefully removed from the soil to ensure their roots didn’t break. A total of 8 randomly selected plants transformed with plasmid pCIP100:VuP5CS and 8 non-transformed plants as well as 4 plants transformed with A. tumefaciens strain LBA4404 with no plasmid from treatment 0 had their root lengths measured and recorded. The number of seeds harvested from the transformed and non-transformed plants from each pod was also counted and recorded. A one way analysis of variance (ANOVA) was applied using R statistical software to separate the means.
Germination and generation of T1 cowpea plants from in planta seed transformation
Cowpea seeds inoculated with A. tumefaciens strain EHA105 harbouring the recombinant plasmids pCIP100: VuP5CS or pCAMBIA1301:VuP5CS germinated after 3 days in vermiculite with the germination in all the treatments being between 41 and 92% (Table 2). Seeds inoculated with A. tumefaciens strain LBA4404 had lower germination compared to those inoculated with strain EHA105 with the lowest germination of 41% being from seeds inoculated with A. tumefaciens strain LBA4404 having no plasmid. All the seedlings which germinated grew to maturity and flowered normally, setting seed in four months (Figure 1). Pods were harvested at maturity and seeds from each pod stored in separate envelopes labelled with the plant number, pod number and number of seeds. The T0 seeds from PCR positive plants were used in generating T1 plants.
Expression of β-glucuronidase activity in transformed cowpea plants
GUS histochemical analysis of plants from in planta cowpea seed transformation in 20 leaves sampled 2 weeks after germination showed no β-glucuronidase activity. However, 2 week old leaves from T1 plants sown from seeds which had shown no GUS activity in the T0 plants showed β-glucuronidase activity in 4 out of 14 plants sampled.
The GUS expression was observed in the root and leaf base with more intensity of the blue coloration seen in the roots (Figure 2). The blue coloration was not seen in the non-transformed plants assayed. The localization of the gusA gene where the β-glucuronidase activity is expressed explains why the GUS activity was not observed in the T0 leaf samples because samples were picked from the leaf tips of the seedlings. The segregation of the gusA gene was 1:3.5 (4:14) for the GUS positive: GUS negative plants. Analysis of seeds harvested from the T1 plants had all seeds from one pod exhibiting β-glucuronidase activity.
PCR analysis of transformed T0 and T1 plants from cowpea in planta seed transformation
PCR analysis carried out on DNA extracted from leaves of T0 plants grown from seeds transformed with A. tumefaciens strain EHA105 harbouring plasmids pCIP100 (treatment 3) resulted in 7 out of 35 plants amplifying the 215 bp fragment of gusA gene (Figure 3). Only samples whose gusA gene amplification showed strong bands were considered to be positive. PCR amplification of the 185 bp nptII gene fragment using DNA extracted from leaves obtained from germinated shoots from seeds inoculated with A. tumefaciens strain EHA105 harbouring plasmids pCIP100:VuP5CS (Treatment 5) showed 5 strong bands out of 39 different leaf samples (Figure 4). The seeds from plants of lanes 9, 10, 12 and 18 in figure 4 were planted in soil with 2 pods having 8 and 7 seeds from plant 9, one pod having 9 seeds from plant 10, 2 pods with 14 and 10 seeds from plant 12 and 2 pods having 12 and 8 seeds from plant 18. The youngest leaf in the first set of trifoliate leaves was used in the T1 PCR analysis (Table 3).
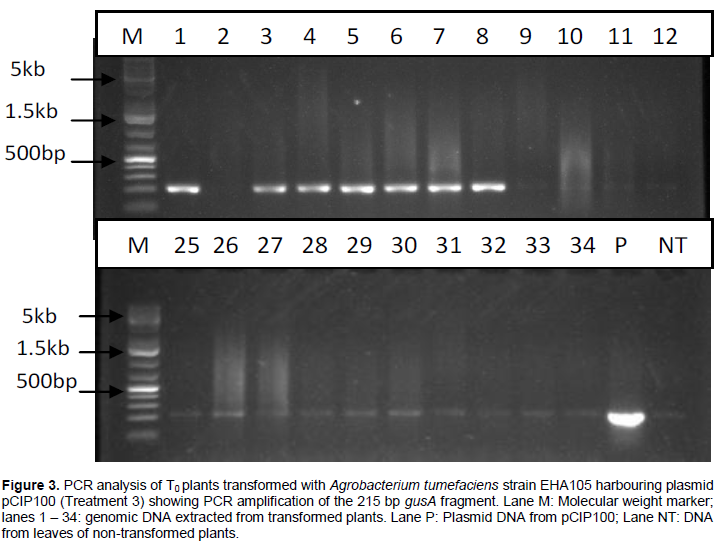
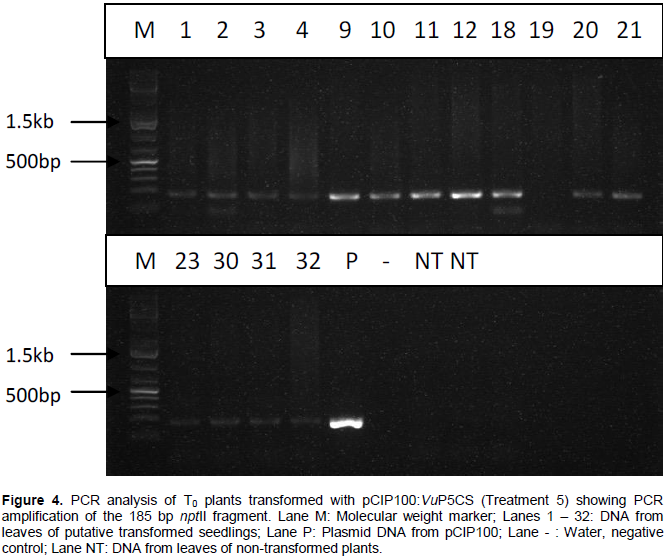

Transformation efficiency in T0 plants from cowpea seeds inoculated by vacuum infiltration was worked out as a percentage of the number of PCR positive plants over the total number of plants inoculated. However, it is worth noting that each of the 5 treatments had different gene constructs or strains of A. tumefaciens. Plants obtained from seeds inoculated with A. tumefaciens without a plasmid (treatment 0) were used as a control as well as non-transformed plants. Leaf samples from plants in treatment 1 where seeds were inoculated with A. tumefaciens strain LBA4404 harbouring plasmid pCAMBIA1301 were used in the GUS histochemical assay. Due to lack of space in the contained greenhouse facilities, the T1 plants could not be analyzed fully and only a few seeds from the PCR positive T0 plants from treatment 4 and 5 were analyzed for the inheritance of the transgene in the subsequent generation. Four leaf samples used were from a plant grown from seeds of a PCR positive T0 plant and were from the same pod. In the T1 plants from treatment 4, only 1 of the 4 samples had a definite positive amplicon for the 890 bp hpt gene fragment (Figure 5), showing the inheritance of the transgene to the progeny with an inheritance pattern of 1:4 for VuP5CS positive: VuP5CS negative.
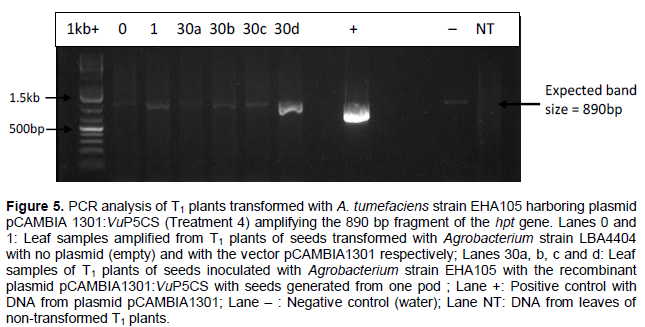
Comparison of proline levels in T1 leaves of transformed and non-transformed cowpea plants
Serial concentration of L-proline at 1, 10, 50, 100, 150, 200, 250 and 300 µM after ninhydrin treatment showed the brick red colour intensity as well as the optical density increasing proportionately to the molar concentration of L-proline (Figure 6). The brick red colour was most intense with the reaction between 300µM proline with acid ninhydrin reagent as illustrated in figure 6a. In figure 6b, the highest brick red intensity was observed with leaf from plant 10 pod b from treatment 5, where seeds were inoculated with A. tumefaciens strain EHA105 harbouring plasmid pCIP100:VuP5CS. The pink colouration was observed in all samples including in the non-transformed leaf samples as well as in leaves from plants transformed with A. tumefaciens with no plasmid indicating the natural occurrence of proline in cowpeas.
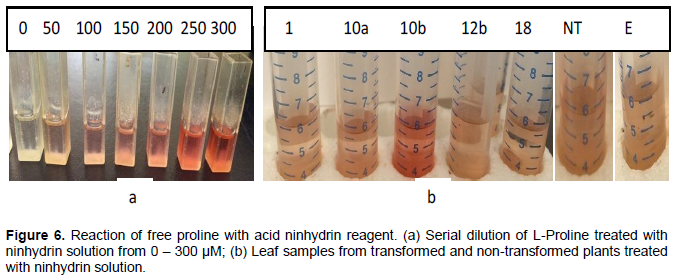
The standard curve (Figure 7) used to work out the concentration of L-Proline resulted in only one leaf sample from plants transformed with plasmid pCIP100:VuP5CS having higher proline concentration in comparison to the non-transformed plants (Figure 8). The proline concentrations in figure 8 match the intensity of the red colouration seen in figure 6b with the highest proline concentration of 93.6 μM/g fresh weight being in plant 5.10 pod b. The lowest proline concentration of 26.5 μM/g fresh weight was in plants transformed with A. tumefaciens strain LBA4404 with no plasmid. The average proline concentration in the transformed leaf samples was 46.15 μM/g fresh weight while the concentration in the non-transformed leaf samples was 41.14 μM/g fresh weight. However, there was no significant difference in proline concentration between the transformed, non-transformed plants and plants transformed with Agrobacterium with no plasmid (F3,10 = 0.4551, p = 0.7195).
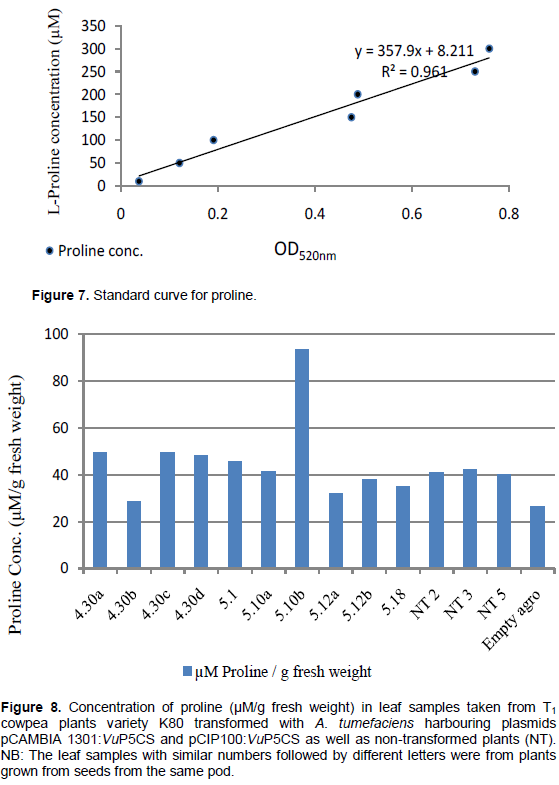
Analysis of roots of transformed and non-transformed T1 cowpea plants
Roots of T1 plants transformed with A. tumefaciens strain EHA105 with plasmid pCIP100:VuP5CS had well established primary and secondary roots which were significantly longer in comparison to the roots of both non-transformed plants and plants transformed with A. tumefaciens with no plasmid (F2,18 = 8.6423, p = 0.00234). Roots of the non-transformed plants were shorter with smaller secondary roots clumped together (Figure 9). The average root length of 8 transformed plants analysed was 22.6 cm, while the average root length of the non-transformed plants was 12.8 cm and 13.0 cm for plants transformed with A. tumefaciens with no plasmid. Nodulation was observed in the roots of both transformed and non-transformed plants.
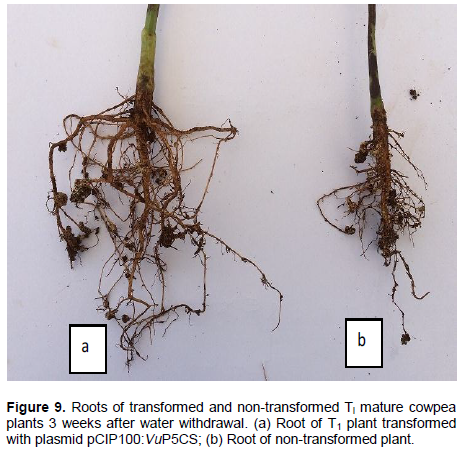
Seeds harvested from VuP5CS transformed T1 plants were significantly more than the seeds harvested from non-transformed plants and plants transformed with A. tumefaciens with no plasmid (F4,181 = 29.573, p < 0.05). There was an average of 10 to 11 seeds per pod from transformed plants while the non-transformed plants had an average of 5 seeds per pod (Table 4). A one way ANOVA using the R statistical software showed no variation in mean number of seeds per pod harvested from plants transformed with strain EHA 105 and LBA4404 or plasmids pCIP100:VuP5CS and pCAMBIA1301:VuP5CS. Similarly, there was no variation in mean number of seeds per pod harvested from non-transformed plants and those from plants transformed with A. tumefaciens with no plasmid (Table 4).
In planta seed transformation has been routinely used in transformation of Arabidopsis (Clough and Bent, 1998) using inflorescence or floral dip, soybean seeds (De Ronde et al., 2001) and cowpea nodal buds (Adesoye et al., 2008). From the T0 plants generated from in planta seed transformation in this study, the highest transformation efficiency achieved was 20.5% with A. tumefaciens strain EHA105 harbouring plasmid pCAMBIA 1301:VuP5CS, and the lowest transformation efficiency of 8.8% was achieved with seeds inoculated with A. tumefaciens strain LBA4404 transformed with the recombinant vector pCAMBIA1301:VuP5CS. Ilori and Pellegrineschi (2011) reported a much lower trans-formation frequency of 0.36% when they transformed pollen in flower buds of Nigerian cowpeas in planta with A. tumefaciens strain pGV 2260 occasioned by flower abortion leading to low pod sets of between 8.9 and 10.2% in the transformed plants. The reason for the flower abortion was attributed to the liquid medium used in the culture of Agrobacterium, suggesting that pollen grains of cowpeas could be hydrophorbic (Ilori and Pellegrineschi, 2011).
In this study, seeds from the same pod of PCR positive T0 plants gave rise to seedlings which when analyzed by GUS histochemical assay, expressed β-glucuronidase activity in 4 out of 14 plants. Stable transformation was however achieved with the T2 plants as the seeds harvested from T1 plants showed the presence of the transgene in all the seeds from the same pod through GUS histochemical staining. In a similar study by Adesoye et al. (2008), in planta transformation used to inoculate cowpea nodal buds by electroporation with the Cry1AB gene against Maruca vitrata and nptII gene produced stable transformation with the T3 plants showing the presence of the Cry1AB gene after PCR analysis. However, the segregation did not follow the Mendelian pattern of inheritance with more of the T1 plants being non-transgenic than expected giving a ratio of between 0 and 1:5.3 for geneticin resistant: susceptible in the T1 progeny. They attributed this to high chimerism in the T0 plants having a lower number of transformed cells compared to the non-transformed cells. Some T1 plants according to Bent (2006) may carry multiple insertion loci thus their T2 progeny families will not exhibit the 3:1 segregation for the transgenes.
De Ronde et al. (2001) successfully transformed soybean seeds in planta and generated 30% transformed T1 progeny from the T0 seeds inoculated. The efficiency was higher than the best efficiency of 20.5% achieved in the T0 plants in this study. Therefore, it is evident that in planta transformation has the potential to deliver transformed plants faster and without the tissue culture process characterized by long and sensitive regeneration period on selective medium. It is also cheaper to carry out as inoculation can be carried out in established plants in the greenhouse (Adesoye et al. 2008). A simplified method for Agrobacterium-mediated transformation of Arabidopsis thaliana was developed by Clough and Bent (1998) by dipping immature floral buds in solutions of A. tumefaciens. In the analysis of Artemisia thaliana flowers transformed in planta, Bent (2006) noted that T1 plants were hemizygous and some T2 progeny from self-fertilized T1 plants lacked the transgenes. It is therefore necessary to germinate T2 seeds in antibiotic selection medium to eliminate the non-transgenic plants and also plants in which the selectable marker gene has been silenced to allow for phenotypic studies.
The ninhydrin test used to measure proline levels showed no significant difference between the transformed and non-transformed plants. Only one transformed leaf sample had a higher level of proline at 93.6 μM/g fresh weight. This suggests that the accumulation of proline in cowpeas is triggered by water stress as was reported by Gazendam and Oelofse (2007) who concluded that proline levels remained the same in drought tolerant and susceptible cowpea cultivars when the plants were well watered, but increased with water stress, with the susceptiple line accumulating more proline. In contrast, transformation of the tree species Eucalyptus saligna with the P5CS gene using leaf explants, found that the levels of proline increased four times in the leaves of transformed plants in comparison to the level in non-transformed plants without water stress (Dibax et al., 2010). Similarly, Kishor et al. (1995) over expressed the moth bean P5CS gene in transgenic tobacco leading to increased production of proline between830 to 1590 μg/g fresh weight in comparison to 80 to 89μg/g fresh weight in the non-transgenic plants before water stress. After water stress, the proline levels rose to 6500 μg/g and 3500 μg/g fresh weight in the transformed and non-transformed plants respectively. According to Bates et al. (1973), proline increased proportionately faster than other amino acids in plants under water stress, and is thus useful as an evaluating parameter for selecting drought resistant crop varieties.
Although, there was no significant difference in proline levels in the plants transformed with the VuP5CS gene in comparison to the non-transformed plants in this study, the roots of all the 8 plants that were transformed with the VuP5CS gene were two times longer and better developed in comparison to those of non-transformed plants at maturity. This is in agreement with Kishor et al. (1995) who reported 40% longer roots in transgenic P5CS tobacco with twice the biomass of the control plants under salt stress conditions. Similarly, root growth was stimulated with application of proline to shoots of Arabidopsis thaliana with reduced root length observed in P5CS1 mutants in a study by Sharma et al. (2011).
The results in this study suggest that the increased root length and more seeds produced per pod in the transgenic lines would possibly allow continued growth of the plants under water stress, thus, conferring drought tolerance.
This is the first report on in planta transformation of cowpea seeds by vacuum infiltration based on documented literature with transformation efficiency which is 10 to 20 times higher than that which is achieved from in vitro transformed and regenerated cowpea plants in documented literature. A. tumefaciens strain EHA105 gave the highest transformation efficiency of 20.5% and was successfully used to transform cowpea seeds in planta by vacuum infiltration with VuP5CS recombinant plasmids. Proline analysis of the T1 plants transformed with the VuP5CS gene construct had no significant difference in proline content in the transformed and non-transformed plants. The roots of all the transformed plants were twice as long as those of non-transformed plants with the transformed plants having more seeds per pod at maturity, suggesting that the transformed plants may have better growth under water stress. The transgenic VuP5CS cowpeas have the potential to allow farmers a decent harvest in case of early cessation of rains in the arid and semi-arid areas of Kenya where farmers solely depend on rainfall, therefore improving food security.
Other important traits can be introduced into cowpeas using the established protocols. The conclusion of this project and its adoption could potentially benefit farmers in the arid and semi-arid regions of Kenya where cowpeas are grown under rain fed conditions and contribute towards food security in the region.
The results of this study need to be carried on a step further to screen the seeds from the T1 plants to allow for the proline analysis on the T2 plants as well as the physiological characterization of the transformed and non-transformed plants under normal and water stress conditions.
The authors have not declared any conflict of interest.
The laboratory aspects of this project was partly supported by the BecA-ILRI Hub through the Africa Biosciences Challenge Fund (ABCF) program. The ABCF Program is funded by the Australian Department for Foreign Affairs and Trade (DFAT) through the BecA-CSIRO partnership; the Syngenta Foundation for Sustainable Agriculture (SFSA); the Bill & Melinda Gates Foundation (BMGF); the UKDepartment for International Development (DFID) and; the Swedish International Development Cooperation Agency (Sida). Part of the study was funded by the Kenya National Commission for Science, Technology and Innovation. The authors also like to acknowledge the International Institute of tropical Agriculture (IITA) and the International Potato Center (CIP) for their kind donations of plasmids pCAMBIA 1301 and pCIP100 respectively.
REFERENCES
Adesoye AI, Machuka J, Togun AO (2008). CRY 1AB transgenic cowpea obtained by nodal electroporation. Afr. J. Biotechnol. 7(18):3200-3210.
|
|
Adesoye AI, Togun AO, Machuka J (2010). Transformation of cowpea (Vigna unguiculata L. Walp.) by Agrobacterium infiltration. J. Appl. Biosci. 30:1845-1860.
|
|
Agbicodo EM, Fatokun CA, Muranaka S, Visser RGF, Linden CG (2009). Breeding drought tolerant cowpea: Constraints, accomplishments and future prospects. Euphytica 167:353-370.
Crossref
|
|
Amanullah M (2011). Proteins In: Amanullah M (Ed) Medical Biochemistry and Biotechnology, New central book agency (P) Ltd., Kolkata. 391p.
|
|
Bakshi S, Sadhukhan A, Mishra S, Sahoo L (2011). Improved Agrobacterium-mediated transformation of cowpea via sonication and vacuum infiltration. Plant Cell Rep. 30:2281-2292.
Crossref
|
|
Bates LS, Waldren RP, Teare ID (1973). Rapid determination of free proline for water stress studies. Plant Soil 39:205-207.
Crossref
|
|
Bent A (2006). Arabidopsis thaliana floral dip transformation method. Agrobacterium protocols. pp. 87-104.
Crossref
|
|
Clough SJ, Bent AF (1998). Floral dip: a simplified method for Agrobacterium mediated transformation of Arabidopsis thaliana. Plant J. 16(60):735-743.
Crossref
|
|
De Ronde JA, Cress WA, Van der Mescht A (2001). Agrobacterium mediated transformation of soybean (Glycine max) seed with the β-glucuronidase marker gene. South Afr. J. Sci. 97:421-428.
|
|
Dibax R, Deschamps C, Filho B, Vieira GE, Molinari HBC, De Campos MFK, Quoirin M (2010). Organogenesis and Agrobacterium tumefaciens-mediated transformation of Eucalyptus saligna with P5CS gene. Biol. Plant. 54(1):6-12.
Crossref
|
|
Doyle JJ, Doyle JL (1987). A rapid DNA isolation procedure for small quantities of fresh leaf tissue. Phytochem. Bull. 19:11-15.
|
|
Fatokun C, Boukar O, Muranaka S, Chikoye D (2009). Enhancing drought tolerance in Cowpea. Afr. Crop Sci. Confer. Proc. 9:531-536.
|
|
Gazendam I, Oelofse D (2007). Isolation of cowpea genes conferring drought tolerance: Construction of a cDNA drought expression library. Water SA 33:387-391.
|
|
Gill SS, Tuteja N (2010). Reactive oxygen species and antioxidant machinery in abiotic stress tolerance in crop plants. Plant Physiol. Biochem. 48(12)909-930.
Crossref
|
|
Higgins TJ, Gollasch S, Molvig L, Moore A, Popelka C, Watkins P, Armstrong J, Mahon R, Ehlers J, Huesing J, Margam V, Shade R, Murdock L (2013). Genetic transformation of cowpea for protection against bruchids and caterpillars. Proceedings of the fifth world cowpea conference pp. 131-137.
|
|
Ilori CO, Pellegrineschi A (2011). Transgene expression in cowpea (Vigna unguiculata (L.) Walp.) through Agrobacterium transformation of pollen in flower buds. Afr. J. Biotechnol. 10(56):11821-11828.
|
|
Jefferson RA, Kavanagh TA, Bevan MW (1987). GUS Fusions: β-glucuronidase as a sensitive and versatile gene fusion marker in higher plants. EMBO J. 6(13)3901-3907.
|
|
Karanja J, Nguluu S, Gatheru M (2012). Farm yard manure reduces the virulence of Alectra vogelii (Benth) on cowpea (Vigna unguiculata). Afr. J. Plant Sci. 6(3):130-136.
Crossref
|
|
Kikkert JR, Vidal JR, Reisch BI (2005). Stable transformation of plant cells by particle bombardment/biolistics. Methods Mol. Biol. 286:61-78.
|
|
Kimiti JM, Odee DW, Vanlauwe B (2009). Area under grain legumes cultivation and problems faced by small holder farmers in legume production in the semi-arid Eastern Kenya. J. Sustain. Dev. Afr. 11(4)305-315.
|
|
Kishor PBK, Hong Z, Miao C, Hu CA, Verma DPS (1995). Overexpression of Δ-pyrroline-5-carboxylate synthetase increases proline production and confers osmotolerance in transgenic plants. Plant Physiol. 108:1387-1394.
|
|
Kishor PBK, Sangam S, Amrutha RN, Laxmi PS, Naidu KR, Rao KRSS, Rao S, Reddy KJ, Theriappan P, Sreenivasulu N (2005). Regulation of proline biosynthesis, degradation, uptake and transport in higher plants: Its implications in plant growth and abiotic stress tolerance. Curr. Sci. 88(3):424-438.
|
|
Mao JQ, Zaidi MA, Arnason JT, Altosaar I (2006). In vitro regeneration of Vigna unguiculata (L.) Walp. cv. Blackeye cowpea via shoot organogenesis. Plant Cell Tissue Organ Cult. 87:121-125.
Crossref
|
|
Ogunmodede BK and Oyenuga VA (1970). Vitamin B content of cowpeas (Vigna unguiculata Walp.) II. Pyridoxine, pantothenic acid, biotin and folic acid. J. Sci. Food Agric. 21(2):87-90.
Crossref
|
|
Popelka JC, Gollasch S, Moore A, Molvig L, Higgins TJ (2006). Genetic transformation of cowpea (Vigna unguiculata L.) and stable transmission of the transgene to progeny. Plant Cell Rep. 25:304-312.
Crossref
|
|
Popelka JC, Terryn N, Higgins TJV (2004). Gene technology for grain legumes: can it contribute to the food challenge in developing countries? Plant Sci. 167:195-206.
Crossref
|
|
Raveendar S, Premkumar A, Sasikumar S, Ignacimuthu S, Agastian P (2009). Development of a rapid, highly efficient system of organogenesis in cowpea, Vigna unguiculata (L.) Walp. South Afr. J. Bot. 75:17-21.
Crossref
|
|
Saini R, Sonia, Jaiwal PK (2003). Stable genetic transformation of Vigna mungo L. Hepper via Agrobacterium tumefaciens. Plant Cell Rep. 21(9):851-859.
Crossref
|
|
Sharma S, Villamor JG, Verslues PE (2011). Essential role of tissue-specific proline synthesis and catabolism in growth and redox balance at low water potential. Plant Physiol. 157:292-304.
Crossref
|
|
Shinozaki K, Shinozaki KY (1997). Gene expression and signal transduction in water-stress response. Plant Physiol. 115:327-334.
Crossref
|
|
Singh B, Hartmann P, Fatokun C, Tamo M, Tarawali S, Ortiz R (2003). Recent progress in cowpea improvement. Chronica Hortic. 43(2)8-12.
|
|
Solleti SK, Bakshi S, Sahoo L (2008). Additional virulence genes in conjunction with efficient selection scheme, and compatible culture regime enhance recovery of stable transgenic plants in cowpea via Agrobacterium tumefaciens-mediated transformation. J. Biotechnol. 135:97-104.
Crossref
|
|
Szabados L, Savoure´ A (2009). Proline: a multifunctional amino acid. Trends Plant Sci. 15(2)89-97.
Crossref
|
|
Tang Y, Chen L, Li XM, Li J, Luo Q, Lai J, Li HX (2012). Effect of culture conditions on the plant regeneration via organogenesis from cotyledonary node of cowpea (Vigna unguiculata L. Walp). Afr. J. Biotechnol. 11(14)3270-3275.
Crossref
|
|
Tardieu F (2012). Any trait or trait-related allele can confer drought tolerance: just design the right drought scenario. J. Exp. Bot. 63(1)25-31.
Crossref
|
|
Tshovhote NJ, Nesamvuni AE, Raphulu T, Gous RM (2003). The chemical composition, energy and amino acid digestibility of cowpeas used in poultry nutrition. South Afr. J. Anim. Sci. 33(1):65-69.
Crossref
|
|
USAID (2010). Staple food value chain analysis: Country rep. Kenya. pp. 150-157.
|