New maize varieties with tolerance to biotic and abiotic stresses and with better agronomic traits have been developed in Africa maize breeding programs. The spotted stem borer (
Chilo partellus Swinhoe) Pyralidae and African stem borer (
Busseola fusca Fuller) Noctuidae
, Lepidoptera are among the most damaging insect pests that greatly reduce maize grain yield in east African environments (Citation). Tropical environments are favourable for insect development and lead to the formation of several generations of the pests in the same season leading to severe crop yield losses (
Mailafiya et al., 2011). For example, in Kenya, grain yield loss due to stem borers in maize is estimated annually at about 400,000 metric tons or about $72 million (De Groote et al., 2003; De Groote et al., 2005). This amount represents an average of 13.5% of the farmers' total annual harvest of maize.
Development of effective methods requires a better understanding of the genetic basis of the resistances among the germplasm used. Suitable maize germplasm should have resistance to both
B. fusca and
C. partellus. Recent reports indicate that climate change has led to
C. partellus increasingly displacing
B. fusca from the high altitude areas in Kenya (
Mailafiya et al., 2011;
Tefera et al., 2011; Mwimali et al., 2015). Furthermore, farmers exchange maize germplasm across agro-ecologies, therefore, the need to investigate the reaction of these tropical maize inbred lines for resistance to these borers becomes paramount. The aim of this study was to evaluate the responses of tropical maize inbred lines for resistance to
B. fusca and
C. partellus the two major stem borer species in Eastern Africa.
Germplasm
One hundred and twelve (112) maize inbred lines used in the study were sourced from the International Maize and Wheat Improvement Center (CIMMYT), Mexico and the Kenya Agricultural and Livestock Research Organization (KALRO) breeding programmes (Table 1). Two elite, but stem borer resistant and susceptible maize lines from CIMMYT and KALRO were included as checks. The lines were developed from the CIMMYT multiple borer resistance (MBR) population. The MBR population was developed through a recurrent selection method under artificial infestation with southern corn borer (SWCB), sugarcane borer (SCB)
Diatrae sacharalis, European corn borer (ECB)
Ostrinia nubilalis, and fall armyworm (FAW)
Spodoptera species in various locations globally (
Smith et al., 1989).
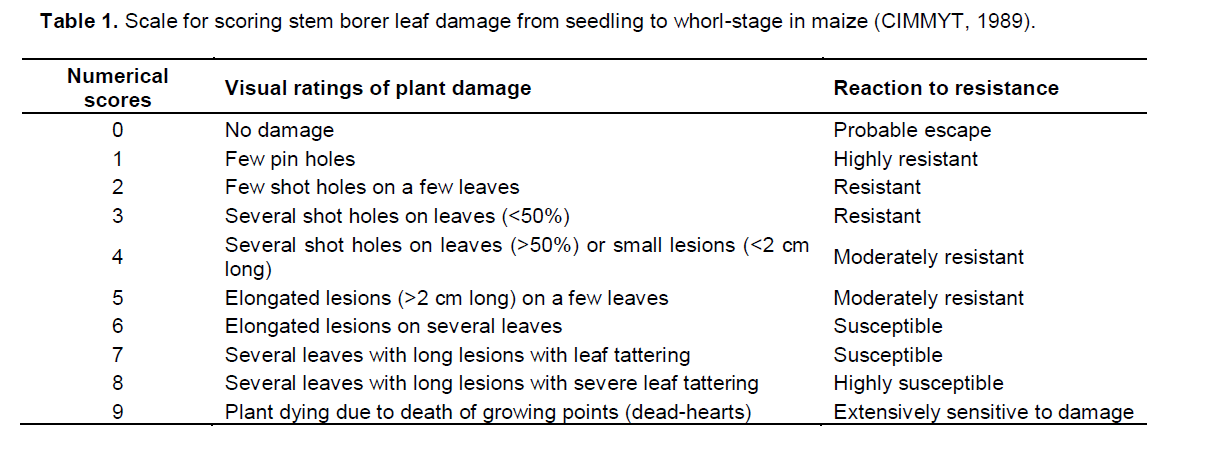
Testing locations
Experiments were established at KALRO Kakamega, KALRO Kiboko, and KALRO Embu sites in Kenya. KALRO Kakamega (37°75’E 2° 15’S, 1585 m asl) centre is located in the moist transitional mid altitude agro‑ecological zone of Western Kenya and experiences mean annual temperatures of 25°C. Kakamega lies within a high potential agro-ecological zone and receives a bimodal mean annual rainfall of approximately 1850 to 1916 mm. The soils in Kakamega are well drained, moderately deep to very deep, red to dark in colour and in some places shallow over petroplinthite (Jaetzold and Schmidt, 1982).
KALRO-Kiboko (2°15'S 37°75' E, 975 m asl) is located in the dry mid altitude agro-ecological zone of Eastern Kenya and experiences mean annual temperature ranges of 28 to 37°C, with February and October being the hottest months. Kiboko receives a mean annual rainfall of approximately 530 mm. The soils are well drained, Fluvisols, Ferralsols, and Luvisols with soil pH of about 7.9 (Jaetzold and Schmidt, 1982;
KARI Land Resources and Analytical Services, 2007).
KALRO-Embu centre (03°56' 44'S and 39°46' 00'E, 1510 m asl) is located in the moist transitional mid altitude agro-ecological zone of eastern slopes of Mt. Kenya and experiences mean annual temperature ranges of 14 to 25°C. Embu lies within a high potential agro-ecological zone. Rainfall received is bi-modal ranging between 800 and 1400 mm annually. The soils are deep (about 2 m); well weathered Humic Nitisols with moderate to high inherent fertility (Jaetzold and Schmidt,1982).
Experimental design and treatments
The maize inbred lines were evaluated in a 28 × 4 α-lattice design with three replications in each location. Each inbred line was sown to one row plot of 6.75 m each per replication. Two seeds were sown per hill and later thinned to one. Each plot consisted of one row with inter-row spacing of 0.75 m and inter-hills spacing of 0.25 m within the rows.
Fertilizers were applied to give 60 kg N and 60 kg P2O5 ha-1 as recommended for each location. Nitrogen was applied in two splits, while supplementary irrigation was applied when needed. The fields were kept free of weeds by hand weeding throughout the growth cycle.
Artificial infestation with insects
Each 6.75 m plot was divided into three parts, namely,
B. fusca and
C. partellus infested on either side of the plot at Embu and Kakamega, while the middle part was protected using insecticide Bulldock
® (active ingredient, beta cyfluthrin 25 g/L). At Kiboko, 5 m row plots were used, and were infested with
C. partellus on one half of the plot while the remaining part was protected using the insecticide. Insect larvae were obtained from the International Centre for Insect Physiology and Ecology (ICIPE) and the KALRO Katumani centres’ stem borer insect pests mass rearing facilities (Tefera et al., 2010,
2011). Plants were artificially infested in a controlled and uniform manner with the respective stem borer species by placing 10 first instar larvae in the maize whorl using a camel brush 21 days after planting.
Data collection and analysis
Plants were evaluated for leaf damage scores using a scale of 1 (resistant) to 9 (susceptible) (Table 1) (
CIMMYT, 1989) at the V3 stage of maize growth.
Other plant damage parameters were measured at harvest, namely, cumulative tunnel length (measured as the total length of tunneling along the maize stalk), tunnel length to plant height ratio, number of exit holes, number of dead-hearts, stalk strength, and number of larvae recovered per plant. Agronomic traits were measured following standard protocols used at CIMMYT (
CIMMYT, 1989). The traits measured were number of days to anthesis and to silking, plant height (cm), ear height (cm), ear position (ratio of plant height to ear placement), number of ears harvested, stem and root lodging, grain weight (kg) and moisture content (%) at harvest, plant stand (number of plants per row at harvest), number of rotten ears, plant and ear aspect (where 1=good and uniform plants/ears with the stature, colour and strength preferred in the area, 5=ugly plants/ears with the undesirable features in the area), stem diameter (measurement across the stalk) (cm), internode length (four below the upper-most ear), and leaf damage.
A rank summation index (RSI) was constructed to determine the ranking of each line within the population for suitable response. The index was obtained by the sum of the means of each of the leaf feeding damage score, number of dead-hearts, number of exit holes, and cumulative stem tunnel length for each line, to get its mean performance when compared with other lines within the same population. An entry with the least value was ranked higher for the resistance traits. The rank selection index (Mulamba and Mock et al., 1978; Mutinda et al., 2013) was determined as follows:
RSI=∑Ri’s
where Ri is the rank of mean of each of the desired traits. Rank summation index is the mean performance of each of the desired traits of each genotype using the ranking of leaf feeding damage score, number of dead-hearts, number of exit holes, and cumulative stem tunnel length.
Least square means for insect damage parameters and agronomic traits were calculated using plot data for each location separately. All analysis of variance using PROC GLM of SAS was performed for individual as well as for combined environments, considering environments as random effects and genotypes as fixed effects (SAS Institute Inc., 2012). Genotypic and phenotypic correlation coefficients were determined using variance-covariance matrix and estimates of genotypic and phenotypic variances (Falconer and Mackay, 1996).
Genotypic correlation was calculated as follows:
H2=Vg/Vp,
where Vg is the genotypic variance, while the Vp is the phenotypic variance.
Mean performance of maize inbred lines
There were highly significant differences for resistance to both B. fusca and C. partellus (p≤0.01) (Tables 2 and 3). At Embu and Kakamega, significant differences were observed for leaf feeding damage (p≤0.01), number of exit holes (p≤0.03 to 0.04), and number of dead hearts (p≤0.01) for B. fusca and C. partellus, except for cumulative stem tunnel length for both sites.
At Embu and Kakamega, the genotypic variances ranged from 0.01 to 0.36 for all sites under B. fusca and C. partellus infestation (Table 3). Mean performance of entries at Embu under B. fusca infestation showed a wide range for dead hearts (0 to 3.05), leaf feeding damage (1 to 6.76), number of exit holes (0 to 11.40), and cumulative tunnel length (0.08 to 5.48 cm). There was a varied range for heritability estimates (0.18 to 0.58) for all traits among the top 19 entries under B. fusca infestation (Table 2).
In Kakamega, the mean performance of entries under B. fusca infestation showed a wide range for dead hearts (0 to 3.31), leaf feeding damage (0.96 to 4.03), number of exit holes (2.15 to 9.08), and cumulative tunnel length (0.02 to 2.27 cm). There was a diverse range for heritability estimates (0.28 to 0.58) for all traits among the top 19 entries under B. fusca infestation (Table 3).
Mean performance of entries at Embu under C. partellus infestation showed a wide range for dead hearts (0.00 to 1.33), leaf feeding damage (1.72 to 6.65), number of exit holes (0.30 to 6.93), and cumulative tunnel length (0.02 to 0.52). There was a varied range for heritability estimates (0.31 to 0.74) for all traits among the top 19 entries under B. fusca infestation (Table 4).
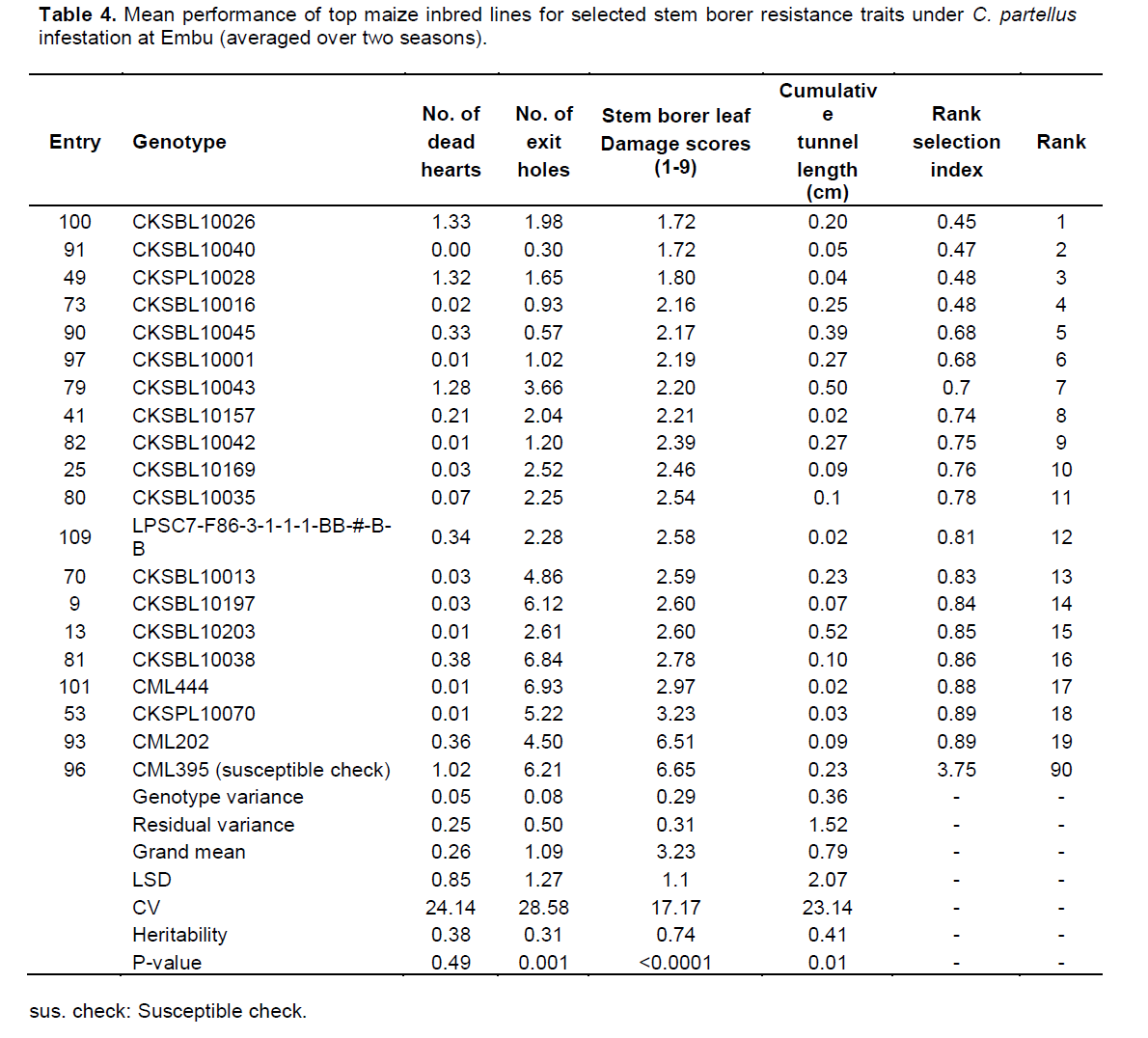
In Kakamega, the mean performance of entries under C. partellus infestation revealed a widespread range for dead hearts (0.00 to 1.32), leaf feeding damage (1.80 to 3.47), number of exit holes (0.17 to 1.79), and cumulative tunnel length (0.00 to 2.18). There was a varied range for heritability estimates (0.11 to 0.78) for all traits among the top entries under C. partellus infestation (Table 5). Similarly, in Kiboko, the mean performance of entries under C. partellus infestation revealed a wide range for dead hearts (0.00 to 1.02), leaf feeding damage (1.40 to 6.65), number of exit holes (0.10 to 6.21), and cumulative tunnel length (0.08 to 2.29). There was a diverse range for heritability estimates (0.11 to 0.78) for all traits among the top entries under C. partellus infestation (Table 6).
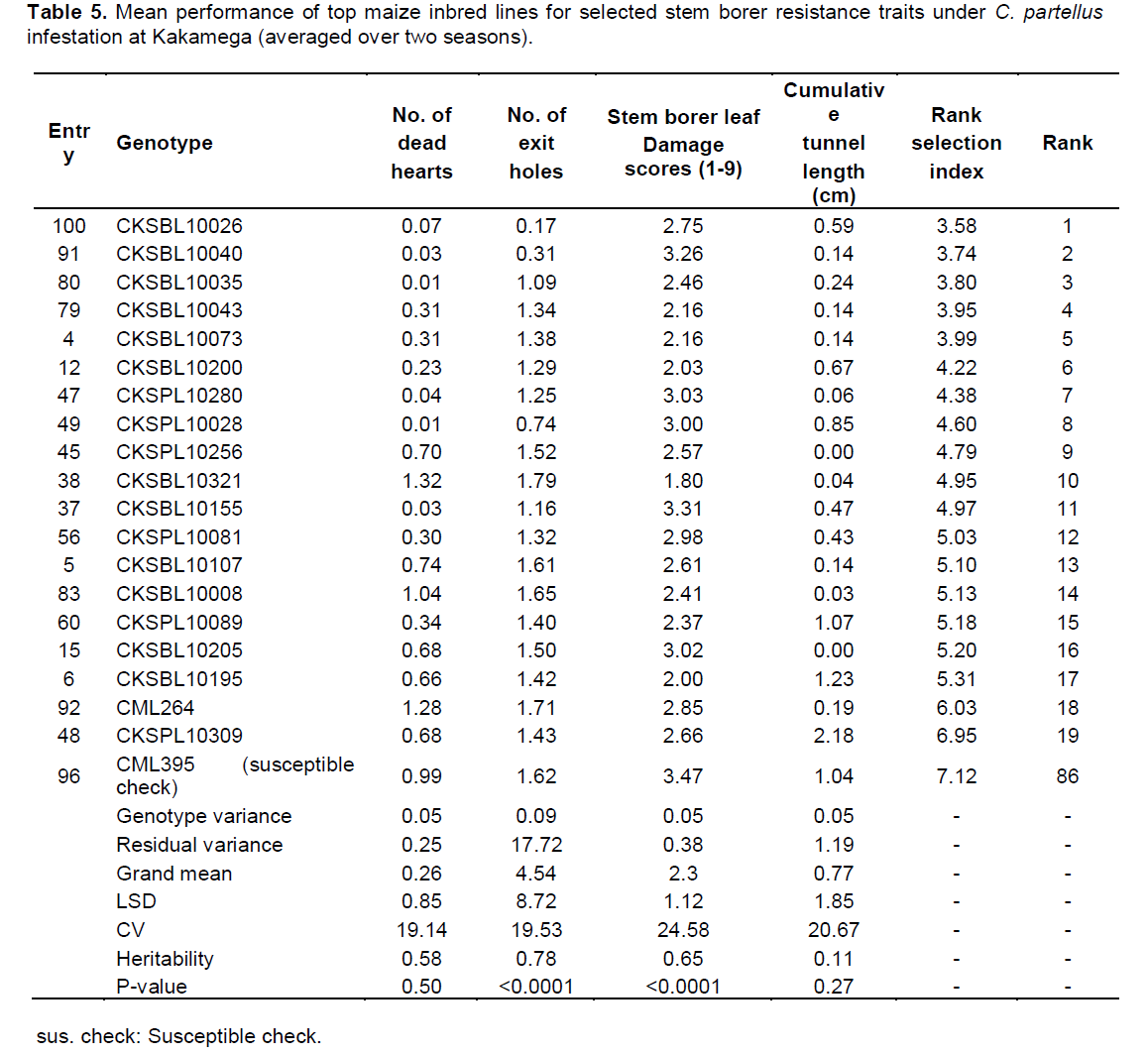
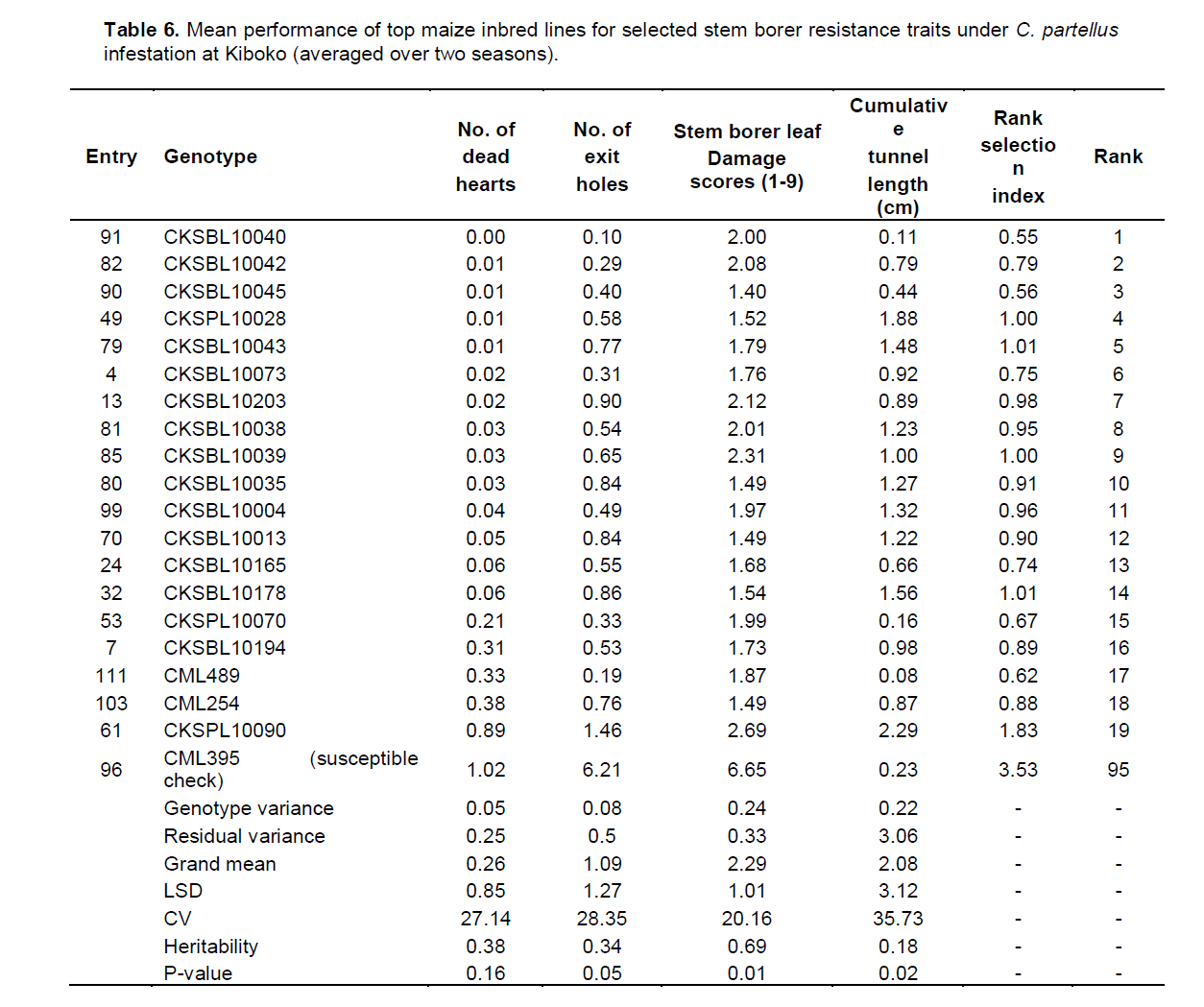
There were C. partellus only resistant entries at Embu (8), Kiboko (9), and Kakamega (4) and 6 each for B. fusca only resistant entries at Embu and Kakamega. Twenty one entries showed combined resistance to both B. fusca and C. partellus in at least two sites: entries CKSBL10026 and CKSBL10028.
Observations on the number of dead hearts and Number of exit holes may imply that trait variations for borer resistance are not completely under genetic control. The higher genotypic variances than the environmental variances suggest that selection for particular stem borer resistance trait can be carried out and that progress can be made.
The suggestions may apply to observations on the moderate to high broad sense heritability values for borer resistance traits. In both maize and sorghum, the role of leaf resistance and other traits in conferring resistance to stem borers
C. partellus (Swinhoe),
O. nubilalis (Hubner),
Sesamia nonagrioides, and
Diatreae species is well documented (
Butrón et al., 2009;
Singh et al., 2012). Even though heritability estimates indicate the relative values of selection based on the phenotypic expression, it is not definitive unless genetic gain under selection is considered together with heritability (
Akinwale et al., 2011). The low to moderate broad sense heritabilityestimates (H
2<0.50) for characters, such as number of dead hearts, number of exit holes, leaf feeding damage and cumulative stem tunneling may be due to environmental influence on the traits.
Since selection indices for stem borer resistance traits provide efficiency in the improvement of quantitatively inherited traits, such as stem borer resistance in maize (Mulamba and Mock, 1978; Mutinda et al., 2013), a rank selection index was used to identify genotypes with resistance for both B. fusca and C. partellus. In response to tropical maize inbred lines for resistance to two B. fusca and C. partellus, stem borers showed that resistance may be exclusive for B. fusca only or C. partellus only or for both borers where they exist. It was observed that five entries had resistance to B. fusca only, 26 entries showed resistance to C. partellus only, and 21 entries showed combined resistance to both B. fusca and C. partellus in at least two sites. Four entries CKSBL10025, CKSBL10039, CKSBL10040, and CKSBL10028 showed resistance to both species across the sites. Eighty four and 28 entries, respectively showed susceptibility to B. fusca and C. partellus in all test genotypes (Table 7). Most of the genotypes were found to be susceptible to B. fusca and less so for C. partellus. These may be attributed to its (B. fusca) fitness and adaptation in Africa, because it is indigenous unlike C. partellus. These findings suggest that genotypes with the specific borer resistance can be deployed directly as parent lines in the formation of hybrids with resistance to B. fusca and C. partellus to areas where these borers occur in league or exclusively.
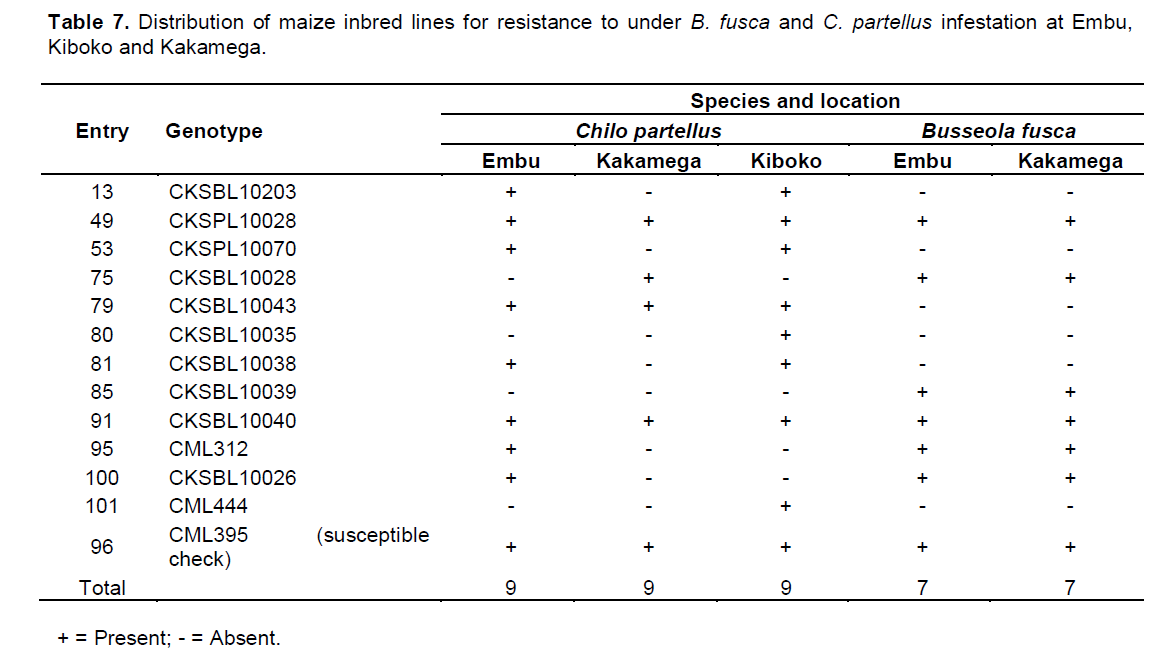
The knowledge on genetic correlations between borer resistance traits is important in creating selection criteria (
Sujiprihati et al., 2003). Since grain yield is a result of interrelationships of yield components (Schnable and Springer, 2013; Udaykumar et al., 2013), to maintain grain yield, breeding for stem borer resistance should be based on multi-trait selection. To do this, several correlations for stem borer resistance traits for
B. fusca and
C. partellus were examined to understand their relationships. There were highly significant differences for correlations among the lines for resistance to both
B. fusca and
C. partellus and agronomic traits in all sites. The correlation coefficients were positive and significant for the number of exit holes and stem diameter for
B. fusca r=0.83, (p≤0.01) while that for
C. partellus was r=0.39, (p≤0.01).
The findings from the current study, corroborate with previous studies that have shown that most cultivated grass species have large stem diameters that support a higher larval survival and more larvae have been recovered per plant unlike wild grass species (
Akinwale et al., 2011;
Hosseini et al., 2011). However, there were no significant correlations between leaf feeding damage and the number of exit holes for
B. fusca, but a negative significant correlations r=‑0.45, (p≤0.01) for
C. partellus. For both borers, besides the length of the life cycles for the two borers, morphological characteristics such as trichome density, leaf pubescence, leaf glossiness, thorns, spines, cuticles, and waxes may hinder insect development (
Munyiri et al., 2013;
Santamaria et al., 2013). These may in turn affect the observed differences in resistance traits due to leaf feeding and larval survival on hosts.
Similarly, both B. fusca and C. partellus had negative significant correlations for number of exit holes r=0.68 (p≤0.01) and plant aspect r=-0.62 (p≤0.01), plant height r=‑0.22 (p≤0.01) and leaf feeding damage r=-0.49 (p≤0.01) respectively. In addition, both B. fusca and C. partellus showed negative significant correlation for plant height r=‑0.53 (p≤0.01) and plant aspect r=‑0.53 (p≤0.01), respectively. Leaf feeding damage relative to the cumulative tunneling for both stem borers indicated no significant correlations (Table 8). Based on stem borer resistance trait rank selection indices leaf feeding damage, cumulative stem tunneling and number of exit holes were found to be reliable parameters that may be used in discriminating genotypes for resistance to the two borers. The findings may imply that both B. fusca and C. partellus affect plants negatively in a similar manner. For example, stem tunneling disrupts nutrients and water uptake, leaf feeding damage reduces the photosynthetic area, exit holes may cause weakened stems which may result in susceptible to stem lodging and other plant deformities, thus result in increased losses to grain yield.
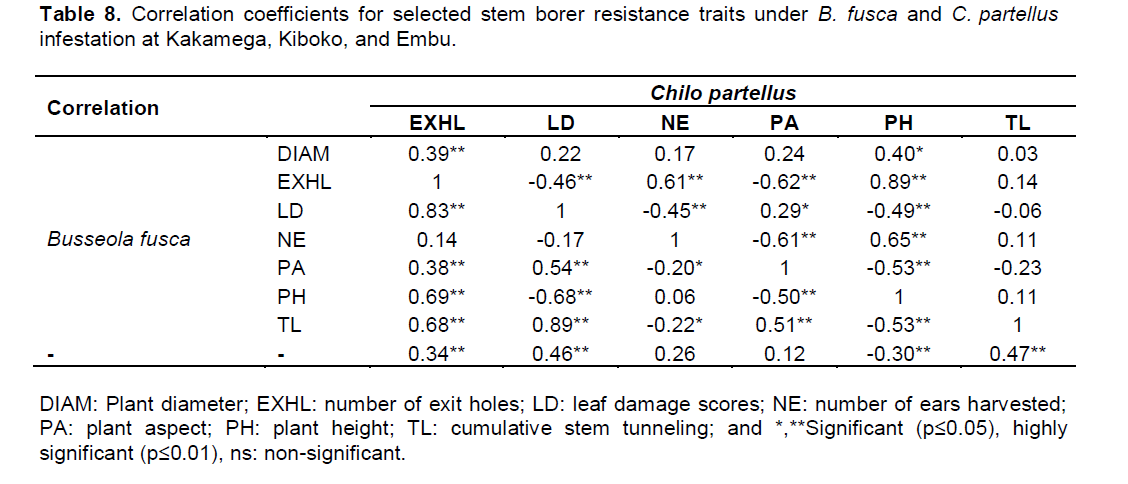
Previous studies showed that stem tunneling damage had a significant influence on maize plant growth, and that the direct effect of stem tunneling on loss in maize grain yield was greater than the effect of leaf feeding (
Kumar, 1997; Singh et al., 2012).
The results from the current study agree with the findings of Ajala et al., (
2010), Akinwale et al. (
2011), and Mailafiya et al., (
2011) reported that leaf damage and cumulative tunneling were positively correlated. These may show differences among
B. fusca and
C. partellus nature of feeding, stem tunneling, oviposition, and exit from host plants. Other studies found that
B. fusca and
C. partellus stem borer damage reduced the number of ears harvested per plant and plant height (Sujiprihati et al., 2003; Sharma et al., 2007; Akinwale et al., 2011).
Further trait correlations between B. fusca and C. partellus revealed positive and significant correlations for for both borers for number of exit holes (r=0.75, p≤0.01), leaf feeding damage score (r=0.55, p≤0.05), cumulative stem tunneling (r=0.26, p≤0.01), number of rotten ears (r=0.47, p≤0.05), number of ears harvested (r=0.72, p≤0.05), number of plants per plot (r=0.73, p≤0.05), plant aspect (r=0.99, p≤0.05), plant height (r=0.81, p≤0.01), root lodging (r=0.50, p≤0.05), and stem lodging (r=0.56, p≤0.05). However, no significant differences were observed for trait correlations between B. fusca and C. partellus for number of dead hearts, stem diameter and internode length across the sites (Table 9).
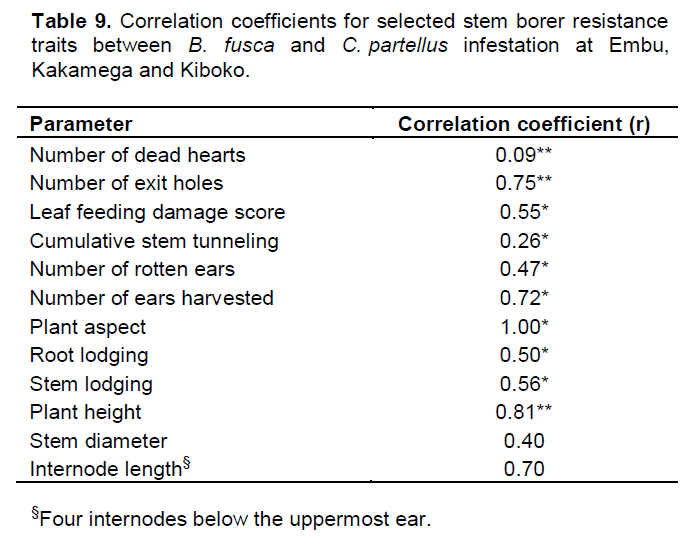
For successful selection of useful genotypes, an understanding of the genotypic and phenotypic inter‑trait correlations is essential. The magnitude of genotypic and phenotypic correlations and their use in selection has been reported in literature (Ali et al., 2008; Al Tabbal and Al-Fraihat, 2012). For example, in this study, genotypic correlations were greater for most of the traits than the phenotypic correlation coefficient values (Table 9).
Grain yield showed significant and high positive genotypic (1.13) and phenotypic (0.83) correlation coefficients and high heritability values for both
B. fusca (0.68) and
C. partellus (0.80). Similarly, high genotypic correlations were observed for number of exit holes (1.01), leaf feeding damage (1.06), and cumulative stem tunneling (1.56) for both
B. fusca and
C. partellus. These may indicate a heritable correlation of these traits (
Sahoo et al., 2011; Al Tabbal and Al-Fraihat, 2012). However, stem borer resistance traits had low heritability for number of dead hearts (0.21), leaf feeding damage (0.47), and cumulative stem tunneling (0.25), except for the number of exit holes (0.71); and correspondingly low phenotypic correlation values of less than 0.60.
Most agronomic traits had high phenotypic and genotypic correlations (0.58 to 1.68) and a wide range for heritability estimates for both B. fusca (0.18 to 0.86) and C. partellus (0.19 to 0.87). Despite the high genotypic variability revealed by the genetic coefficients of variation for the various stem borer resistance and agronomic
traits, it may not provide information on the heritable variation that is useful for genetic improvement (
Akinwale et al., 2011; Singh et al., 2012).
Expected genetic advance may be achieved through phenotypic selection when the genotypic coefficients of variation are coupled with heritable estimates (
Sahoo et al., 2011; Al Tabbal and Al-Fraihat, 2012). Correlation coefficients may be useful as indicators of trait association among the borers, for example, the high number of exit holes and cumulative tunnel length shows the probability that either may be a useful selection criterion for resistance to
B. fusca and
C. partellus in maize.
Similar results have been reported indicating that selection based on these traits may lead to improvement in stem borer resistance (
Munyiri et al., 2013). Low to moderate heritability values were observed for stem
. B. fusca and
C. partellus stem borers’ resistance traits in the test germplasm suggest that those traits are under genetic control.
Previous studies have shown low heritability for various stem borer resistance traits due to compromised experimental procedures, low frequency for resistance genes in the reference populations (
Singh et al., 2012;
Chaudhary, 2013), or due to environmental influence or due to few sites used for evaluations (Falconer and Mackay, 1996).
The overall results suggest that a high variability of germplasm for resistance to B. fusca and C. partellus stem borers exists. Since both B. fusca and C. partellus stem borers are serious insect pests of maize, the identification of germplasm with resistance to these pests is key. The high heritability, genotypic and phenotypic correlations values showed the presence of inherent association between some stem borer resistance traits for both borers. Further genetic improvement may be explored for number of exit holes, cumulative stem tunneling alongside the agronomic traits in selection for the resistance to either or both B. fusca and C. partellus in maize. Leaf feeding damage scores, cumulative stem tunnel length and number of exit holes were the most effective parameters in discriminating the test genotypes for resistance to the two borers.
Genotypes identified for resistance to C. partellus only may be deployed in breeding programmes in zones where C. partellus exclusively occurs and likewise for regions with B. fusca only. Genotypes that showed combined resistance to both borers may be deployed to areas where these borers exist in league. However, breeding for resistance to these borers should continue besides deployment of these stem borer resistant hybrids.
The observed responses to either or both B. fusca and C. partellus, stem borers where they occur exclusively or in league helped to identify resistant maize inbred lines, and showed their possible use in hybrid breeding programmes in tropical maize that emphasize stem-borer resistance especially in Eastern and Southern Africa.
Thanks to the Alliance for a Green Revolution in Africa (AGRA) for financial support during the research; CIMMYT, Kenya for hosting and providing the germplasm; the Director General, KALRO, for granting me study leave, and for providing the research facilities at Embu, Kakamega, Kiboko and Biotechnology Centre during the research period. Thanks to all KALRO and CIMMYT specified centres, especially Mr. Gabriel Ambani, David Karuri, Joel Mbithi and Patrick Gichobi for your kind support during data collection.