Slags from the iron and steel industry may be used in agriculture to correct soil acidity. Current assay assesses the effect of iron and steel industry´s slag, derived from stainless steel, and compares it to limestone as soil acidity corrective and silicon source in coffee plants. The experiment was conducted between December, 2012 and January, 2014 in the municipality of Machado MG Brazil, in a 4-year-old coffee plantation, cultivar Catuaí Amarelo IAC 30. Experimental design comprised randomized blocks in a 2x4 factorial scheme, with control and four replications. Treatments combined two soil acidity correctives, namely, stainless steel slag (“Agrosilício”) and limestone; 4 corrective doses corresponding to 0.5; 1; 1.5; 2 times the amount required to raise index base saturation (V%) of the soil´s surface layer (0 to 0.2m) by 60%. Control did not contain any soil correction. Soil samples were collected during the experiment at depths 0 to 0.1m; 0.1 to 0.2m; 0.2 to 0.4m; similarly, samples of coffee leaves, at 180 and 390 days, respectively, after the application of correctives. Stainless steel slag showed the same efficiency as limestone in soil acidity correction at 0 to 0.1m layer, and in providing Ca2+ to the coffee plant. Stainless steel slag does not correct soil acidity at 0.1 to 0.2 and 0.2 to 0.4 m layers in coffee plants within a 180 day period. Stainless steel slag increases silicon rates in the soil and in the coffee leaves, and increases yield when compared to limestone.
Since high acidity rates characterize most soils in Brazil, soil acidity is one of the main causes of low yield, regardless of the production system (Corrêa et al., 2009; Nogueira et al., 2013). In areas with perennial crops, such as coffee plantation, acidity corrective is applied on the soil surface, although its incorporation to the soil is difficult and causes phytosanitary problems to the shrubs. Since limestone has low water solubility and must contact the soil to react, in systems where it is not incorporated in soil the efficiency of liming and acidity correction at deeper layers decreases (Natale et al., 2012). The same authors report that liming at the soil surface, without any incorporation, provides low movement of the corrective towards deeper layers, which depends on time, fertilization and the dose of the corrective agent.
Limestone is the main acidity corrective agent in agriculture and its employment in acid soils enhances a rise in the soil´s pH and the neutralization of Al3+, provides calcium and magnesium, and causes a greater development in the plant´s root system, with a greater efficiency in the use of nutrients and water (Raij, 2011). Certain industrial slags, such as iron and steel industry slag, may be used in agriculture to replace liming (Prado et al., 2003; Oliveira et al., 2010). Iron and steel industry slag derive from high temperature processing within the limestone and silicon (SiO2) reaction are present in the mineral iron (Deus and Büll, 2013). Approximately 6.25 million tons of slags are annually produced in Brazil but their use in agriculture is still fledging (Deus, 2014).
Since steel slags are more soluble than limestone, they may correct soil acidity faster and deeper when applied at the soil´s surface (Deus and Büll, 2013). These material contain silicon whose effects have been the object of several assays in rice (Artigiani et al., 2014), potato (Pultz et al., 2008), tomato (Marodin et al., 2014), bean (Deus and Büll, 2013), soy and corn (Castro and Crusciol, 2013) and sugarcane (Reis et al., 2013). However, the use of steel slag in agriculture is still rare in Brazil.
The acidity in the soil inhibits the full development of coffee culture. Thus, many farmers resort to techniques in order to increase the intensity of land use (Valipour et al., 2015a). Irrigation is one of the most used technology (Valipour, 2015b), and must point out that with the recent climate change producers will increase their irrigated areas and thus lead to water resources management issues (Valipour, 2014a; Valipour,2014b,2014c). Current assay evaluates the effect of iron and steel industry’s slag, obtained from stainless steel, on soil acidity and as a source of silicon in coffee plantations.
The experiment was conducted between December, 2012 and January, 2014 in the municipality of Machado MG Brazil, in a 4-year-old coffee plantation with coffee plants, cultivar Catuaí Amarelo IAC 30. Figure 1 shows climate conditions (temperature and rainfall) during the experimental period.
The soil of the experimental area was classified as Oxisol, medium texture with initial chemical routine analysis (Silva, 1999) at 0 to 0.2 m depth layer (Table 1). Following Camargo et al. (2009), results of granulometry at this layer comprised: 279 g kg-1 clay; 114 g kg-1 silt; and 607 g kg-1 sand.
Experimental design comprised randomized blocks in a 2 x 4 factorial scheme, with control and 4 replications, totaling 36 split-plots. Treatments were composed of a combination of two soil´s acidity correctives, namely, stainless steel slag (“Agrosilício”) and limestone, with four doses 0.5; 1; 1.5; 2 times the amount required to raise soil base saturation (V%) of the surface layer (0 to 0.2m) by 60%. No soil acidity corrective was used in control.
The necessary amount of each corrective was calculated by the formula suggested by Raij (2011), which comprised base saturation required by the coffee plant, soil base saturation of the surface layer (0 to 0.2m); cation exchange capacity (CEC) potential of soil at layer 0 to 0.2 m; and the neutralization capacity of the corrective (NCC). Correctives had the following chemical characteristics, limestone: CaO = 22%; MgO = 14%; NCC = 85%; stainless steel slags: CaO = 25%; MgO = 6%; silicon = 10.5%; NCC = 60%.
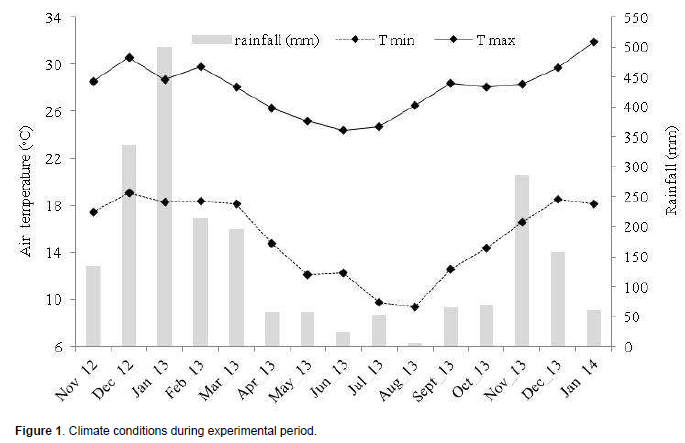
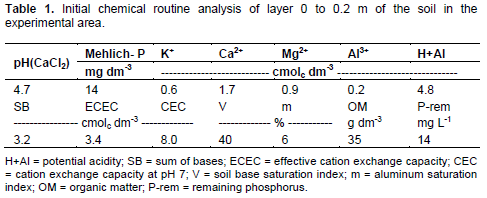
Each split-plot contained 6 coffee plants with 3.0 m spacing between the rows and 0.8 m between the plants, in a total area of 14.4 m2. Useful area (9.6 m2) of each split plot was made up of the four plants at the center of the split-plot. Soil correctives were applied manually in December, 2012, on the soil surface, within the projection of the coffee plant canopy, without any incorporation. The following doses C1 = 0.95; C2 = 1.90; C3 = 2.85; C4 = 3.80 t ha-1; stainless steel slags: E1 = 1.35; E2 = 2.70; E3 = 4.05; E4 = 5.4 t ha-1.
All the split-plots were fertilized uniformly according to the initial chemical analysis of 0 to 0.2 m layer and the intended crop yield. After 180 days from the application of acidity corretictive, soil samples at depths 0 to 0.1m; 0.1 to 0.2 m and 0.2 to 0.4 m were retrieved by a drill from the useful area of each split-plot. Each composed sample was the result of 15 simple samples from each depth. Routine chemical attributes (Silva, 1999) and silicon rates extracted by CaCl2 solution were evaluated in soil samples (Korndörfer et al., 2004).
Crop treatment (weed control and spraying of pesticides) was applied uniformly in all the split-plots according to crop requirements. In June 2013, after 210 days on the application of the acidity correctives, the first harvest of the coffee plants was undertaken, by hand, within the useful area of each split-plot. The coffee berries of each plant were harvested and weighed. The grains were then dried and yield for each split-plot was calculated, in 60-kg bags containing processed coffee grains, following the study of Carvalho et al. (2006).
On the 390th day after the acidity correctives were applied, the leaves of the fruitful branches from each split-plot were harvested, following the study of Raij et al. (1997) to calculate macro- and micro-nutrients (Carmo et al., 2000) and silicon (Korndörfer et al., 2004). Results underwent analysis of variance, Tukey´s mean test and polynomial regression analysis.
There were no significant differences (p> 0.05) between the two acidity correctives studied (limestone and stainless steel slag) with regard to changes in pH, in potential acidity (H+Al), in the base saturation (V) and in Ca2+ concentration in the 0 to 0.1 m soil layer rather, the correctives had a similar behavior in the soil when these factors were taken into consideration. Mg2+ and Si concentration in the soil at the 0 to 0.1m layer were affected differently (p<0.01) by stainless steel slag when compared to limestone. Doses of the acidity correctives (0; 0.5; 1; 1.5 and 2 times correction requirements) affected significantly (p<0.01) the chemical factors evaluated at the 0 to 0.1 m layer. There was no interaction of the factors under analysis (acidity correctives and doses) in the chemical factors pH; H+Al; V; Ca2+; Mg2+ of the soil layer at a depth of 0 – 0.1m. The interactivity correctives x doses affected the silicon concentration in the soil at a depth of 0 to 0.1m.
Corrective doses at depths 0.1 to 0.2m and 0.2 to 0.4m did not influence the evaluated chemical factors and revealed that after 180 days of application the activity of limestone and stainless steel slag remained restricted to the 0 to 0.1 m layer. Whereas mean rates of pH, H+Al, V, Ca2+ and Mg2+ were respectively 4.6; 5.5 cmolc dm-3; 32%; 1.2 and 0.7 cmolc dm-3 at layer 0.1 to 0.2m, the mean rates of these factors at 0.2 to 0.4 m deep were 4.2; 5 cmolc dm-3; 25%; 1.0 and 0.5 cmolc dm-3.
Although silicate minerals are approximately seven times more soluble than limestone, with great mobility in the soil (Alcarde and Rodella, 2003), stainless steel slag in current assay failed to have a greater capacity in correcting acidity in the soil at a certain depth, when compared to limestone. Results may have occurred due to the interval between the application correctives and soil sampling (180 days), the NCC of stainless steel slag and soil texture of the experimental area.
Natale et al. (2012) report that the correction of acidity at the soil´s sub-surface layers with surface application of correctives in areas with perennial crops, such as fruit trees, may occur due to such factors as granulometry of the corrective agent, since the finer particles may easily move throughout the soil´s profile; the displacement of the corrective particles in the soil through canals formed by root decomposition; formation of pairs between bases (Ca2+ and Mg2+) and organic acids (RO- and RCOO-) with high solubility and low molecular mass, which carry them to the deepest layers of the soil, besides the formation of other compounds, such as Ca(HCO3)2 and Mg(HCO3)2; d) nitrogen fertilization which triggers the formation of soluble salts, such as Ca(NO3)2, that percolate through a descending movement of water within the soil profile. According to these authors, the movement of corrective particles within the soil profile depends on the dose used, the interval after application, soil type, fertilization, land slope and type of vegetal covering.
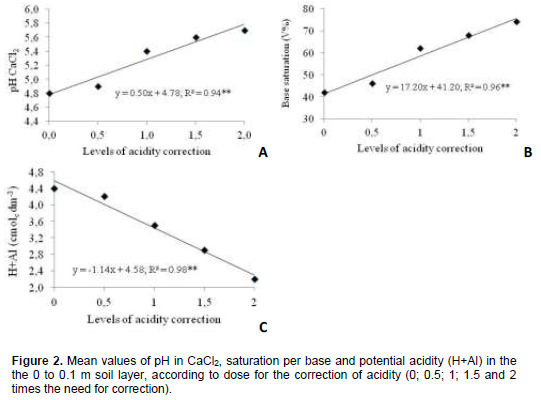
Silva et al. (2004) also reported the correction of acidic soil at 0 to 0.1 m layer with surface application of limestone in coffee crops and they insisted that correction at the sub-surface layers of the soil only occurred 34 months after the first application of the corrective agent and 13 months after the second one. Deus and Büll (2013) registered that in an area with bean tillage system, the corrective effect of stainless steel slag was limited to a depth of 5 cm whereas limestone neutralized soil acidity at the 0 to 0.2 m layer sixty days after their application on the soil surface. In an assay with coffee crops, Melo and Sartori (2013) showed that industrial slag behaved similarly to limestone when correcting soil acidity at a depth of 0 to 0.1 m. However, the two corrective agents did not correct acidity at a depth of 0.1 to 0.2 m after 90 days of application on the soil surface.
Castro and Cruciol (2013) reported that stainless steel slag (Agrosilício) in a tillage system was successful in correcting soil acidity. It was also capable of increasing the soil´s base exchange rates (Ca2+ and Mg2+) at a depth of 0.4m, when compared to limestone, in assessments 12 and 18 months after the application of correctives on the soil´s surface.
Further, pH linear increase occurred in base saturation and in Ca2+ and Mg2+ rates at 0 – 0.1 m depth with the surface application of stainless steel slag and limestone (Figures 2 and 3). According to regression equations, pH in CaCl2 values and bases saturation varied for the two corrective agents, respectively from 4.8 and 41% to 5.8 and 76% in control treatment and in treatment with the highest corrective dose (Figures 2A and 2B). In other words, pH in CaCl2 increased by one unit and V% rates practically doubled in the 0 – 0.1m layer, with the highest level in acidity correction.
Potential acidity (H+Al) of the soil at a depth between 0 and 0.1m decreased linearly with doses, regardless of the corrective agent applied (Figure 2C).
Nogueira et al. (2012) reported similar results in experiments with coffee plants in vases placed in a greenhouse. They registered that slug and limestone with doses featuring 0; 25; 50; 75; 100 and 125% of correction requirements increased pH and decreased potential (H+Al) and exchangeable acidity (Al3+) of clayey latosol. They also reported that slag had a behavior similar to that of limestone in the correction of acidic soil. The effect of silicate slag in soil reaction is due to the neutralization of H+ and release of OH- by silicate anion (SiO3-2) available in the solubilization process of the materials (Prado et al., 2003).
Mean increase in Ca2+ and Mg2+ concentrations in the 0 to 0.1 m soil layer was 2.4 times when the highest slag or limestone dose was employed, when compared to control (Figures 3A and 3B). Mean concentrations of the nutrients varied according to dose of the corrective agents, or rather, from 1.2 to 2.8 cmolc dm-3 in the case of Ca2+ and from 0.8 to 1.9 cmolc dm-3 in the case of Mg2+, which CFSEMG (1999) have considered respectively average and good for Ca2+ and average and very good for Mg2+. Mg2+ rates in soil with limestone treatments averaged 20% higher than those with stainless steel slag (Figure 4A). Higher Mg2+ rates in the soil with limestone are due to a greater concentration of the nutrient in the corrective agent (14% of MgO) than in stainless steel slag (6% of MgO).
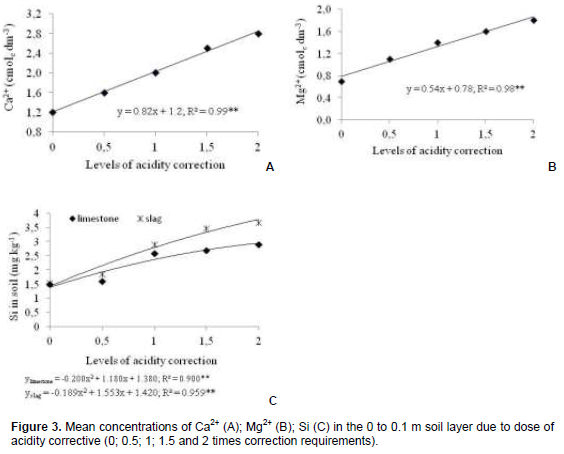
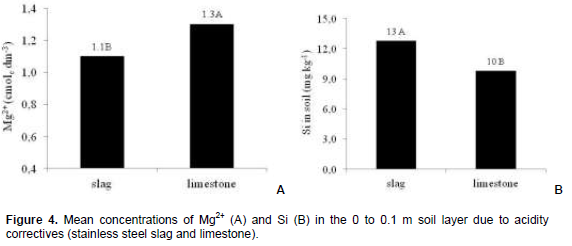
Silva and Coelho (2010) also reported a linear increase in Ca2+ and Mg2+ rates in soil when silicate slag and limestone were applied, in a field assay, in medium texture soil with eucalyptus. The authors also revealed that Ca2+ rates of the soil were similar when the two corrective agents (silicate slag and limestone) were employed, and that treatments with limestone had higher Mg2+ rates in the soil than those with silicate slag. Linear increases in Ca2+ and Mg2+ rates in clayey soil were also reported by Nogueira et al. (2012) in an experiment with coffee plants and slag.
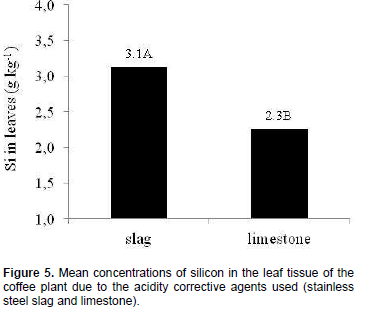
Silicon concentration of soil increased when dose of the corrective agents were applied (Figure 3C), or rather, mean rate of silicon in the soil was 1.3 times greater in treatments with stainless steel slag than in those with limestone (Figure 4B). Stainless steel slag is not only a source of silicon (10.5% of silicon). Rise in the soil´s pH due to corrective agents increases silicon availability caused by decrease of the element´s adsorption caused by the soil´s inorganic colloids (Silva and Coelho, 2010).
Korndörfer et al. (1999), Camargo et al. (2007), Silva and Coelho (2010) and Deus and Büll (2013) reported similar results and detected increase in c rate in the soil when silicate slag was applied. There were no changes in the nutrients concentrations in the leaf tissue of the coffee plant when stainless steel slag and limestone were applied. Mean concentrations of macronutrients were 34; 1.4; 25; 16; 2.9 and 1.8 g kg-1 respectively for N, P, K, Ca, Mg and S. Concentrations were within range for leaf tissues suggested by Raij et al. (1997) for the coffee plant, namely 26-32; 1.2-2.0; 18-25; 10-15; 3.0-5.0; 1.5-2.0 g kg-1 respectively for N, P, K, Ca, Mg and S.
Mean concentrations of micronutrients in the coffee´s leaf tissue were 30; 23; 85; 146 and 22 mg kg-1, respectively for B, Cu, Fe, Mn and Zn, and were within range for leaf tissues suggested by Raij et al. (1997) for the coffee plant, namely, 50 to 80, 10 to 20, 50 to 200, 50 to 200, 10 to 20 mg kg-1, respectively for B, Cu, Fe, Mn and Zn. Silva et al. (2004) did not report any decrease in micronutrient absorption in coffee plants due to the application of acidity corrective agents.
There was an increase of silicon concentrations in the plants´ leaves by simply applying stainless steel slag, even though no definite behavior (linear or quadratic) was reported in its rates in coffee plants with doses of the acidity corrective agent. Mean silicon concentrations in coffee leaves in treatments with stainless steel slag were 1.5 times greater when compared to those with limestone (Figure 5). Silicon concentrations in coffee leaves ranged between 2 and 3 g kg-1 (Reis et al., 2007), similar to those in current assay.
In their tillage experiment in which stainless steel slag and limestone were applied on the soil surface with a clayey texture to raise base saturation at 70% before the succession culture of soybean, Congo signal grass and corn, Castro and Crusciol (2013) failed to report variations in leaf rates of N, K and S of soybean and of P, K and S of corn when correctives were applied. However, the authors registered increase in P concentrations of soybean leaves, N concentrations in corn leaves and in Ca, Mg and silicon concentrations in the two crops when acidity correctives were applied.
Marques (2013) employed different percentages for calcium silicate and limestone to correct soil acidity and failed to report changes in N, P, K, Mg and Zn concentrations in corn leaves, but registered a linear increase in silicon rates in leaves with an increase in calcium silicate amounts applied to the soil. Lopes et al. (2013) did not detect any increase in silicon rates in roots, stem and leaves of coffee seedlings when doses of silicate slug were incorporated in soil used as a substrate for the formation of seedlings. Coffee plant yield from the first harvest provided a quadratic response to stainless steel slag and limestone doses (Figure 6A). According to regression equation, maximum yield (30 bags ha-1) would be obtained with the application of 1.7 times the dose necessary to correct the soil acidity on the soil´s surface. Maximum yield would be 47% greater, or rather, 10 bags ha-1 more than that obtained in control where correction for soil acidity was not performed. Yields in treatments with 1 and 1.5 times correction requirements were respectively 38 and 46% greater than those of control.
Coffee plants are tolerant to acidity but not susceptible to Al3+ toxicity in the soil (Rodrigues et al., 2006). This is the reason why sometimes response of the crop to liming fails (Raij et al., 1996) or occurs in soils with high Al3+ and Mn2+ concentrations (Mendonça et al., 2007), which was not the case in current experiment. In fact, the coffee plant´s high yield response to the application of acidity correctives was unexpected. However, response may have occurred due to the cultivar (Catuaí) employed, with lower tolerance rates to soil acidity that other coffee plant varieties, such as Icatu (Rodrigues et al., 2006; Mendonça et al., 2007).
Silva et al. (2004) also registered an increase in the coffee plant (cv Catuaí) yield during the first and second harvests, with the correction of soil acidity by limestone. Treatments with stainless steel slag as a corrective for acidity averaged a yield 20% higher, or rather, 5 bags ha-1 more when compared to limestone corrective (Figure 6B).
Although the coffee plant is a species which does not accumulate silicon (Reis et al., 2007), increase in silicon concentrations in leaves in treatments with stainless steel slag as a corrective agent against acidity may have increased the structural rigidity of the tissues and made the plants less susceptible to biotic and abiotic stress (Ribeiro et al., 2011). It may also have maintained the plants´ photosynthetic rate and stomach conductance due to a decrease in transpiration through the cuticle (Pulz et al., 2008) at drought intervals during the experiment. This may have been the cause of greater coffee yield in treatments with stainless steel slag when compared to treatments with limestone.
The concentration of some elements in high concentrations in the soil can reduce the production, so a technology to reduce the application of a higher blade is needed to dilute and percolating the excess (Valipour 2012a, 2012b; Yannopoulos et al., 2015).