ABSTRACT
Phosphate solubilization by microorganisms is an important process for increasing the bioavailability of phosphorus (P) to plants. This study aimed to: (a) in solid medium, isolate CaHPO4-solubilizing and non-P-solubilizing microorganisms from the rhizosphere of guanandi (Calophyllum brasiliensis) collected from a nursery and the field; (b) in liquid media, quantify the ability of CaHPO4-solubilizing isolates from the rhizosphere of guanandi to solubilize FePO4; and (c) quantify indoleacetic acid (IAA) production by FePO4-solubilizing rhizosphere microorganisms. Two guanandi plants, one cultivated in nursery and another from the field, were used for the isolation of FePO4 and CaHPO4-solubilizing bacteria and fungi. The largest population of P-solubilizing and non-P-solubilizing microorganisms was found in the rhizosphere of guanandi cuttings cultivated in the field. The abilities to solubilize FePO4 and produce IAA are variable among guanandi rhizosphere isolates, where bacterial isolates produce higher levels of IAA than fungal isolates.
Key words: Phosphorus, auxin, phosphate solubilization, seedlings.
The rhizosphere, where the soil is in direct contact with plant roots, is the region where the majority of the interactions between plants and the microbial community occur (Luster et al., 2009). A wide variety of microorganisms can live near or are associated with the roots, due to stimulation by various root exudates, and they are able to promote greater nutrient absorption and transfer the nutrients to plants through diverse mechanisms. Such microorganisms are called plant growth-promoting rhizobacteria (PGPR). This effect is attributed to mechanisms such as biological nitrogen fixation (BNF); the production of growth-regulating substances, antibiotics and siderophores; and the solubilization of nutrients such as phosphorus (P) (Hayat et al., 2010). Plant growth promoting bacteria and fungi may have potential use as inoculants because they maximize plant development (Sahin et al., 2004; Souchie et al., 2010).
In recent years, the colonization by diazotroph has been discovered in many plants. For instance, maize plants are associated with Bulkhoderia unamae (Caballero-Mellado et al., 2004), rice with Serratia marcescens (Gyaneshwar et al., 2001), wheat with Achromobacter insolitus and Zoogloea ramigera (Sala et al., 2008) sorghum and sugarcane with Gluconacetobacter diazotrophicus (Medeiros et al., 2006; Luna et al., 2010). According to Govindarajan et al. (2007), grasses roots, such as maize, have a large community of rhizobacteria belonging to the family Enterobacteriaceae, including the genus Serratia. Prieschmann et al. (2008) isolated strains of that genus from maize roots found that these bacteria, in addition to exhibit growth-promoting characteristics, also act as antagonists against phytopathogenic fungi. Both free-living and endophytic PGPR can benefit plants by promoting plant growth through more than one mechanism (Ahmad et al., 2008).
After nitrogen (N), P is the second-most limiting element for plant growth. There are large reserves of P in insoluble forms in the soil, and its high reactivity with elements such as aluminum (Al), iron (Fe) and calcium (Ca) makes it unavailable to plants. Among the rhizosphere bacteria, some are capable of secreting organic acids and phosphatases that facilitate the conversion of P from insoluble to soluble forms, making the nutrient available for plants (Chen et al., 2006; Venieraki et al., 2011). Considering that soils from Cerrado domain are extremely weathered with high chemical adsorption, low availability of P and predominance of Fe and Al oxides (Reatto et al., 1998; Resende et al., 2010) the use of P-solubilizing microorganisms play a strategic role in plant nutrition.
Solubilizing microorganism populations are quite large in rhizospheric soil and include bacteria of the genera Rhizobium, Enterobacter, Serratia, Citrobacter, Klebsiella, Pseudomonas, Burkholderia and Achromobacter, among others (Rodríguez et al., 2006). The use of these microorganisms as inoculants for crops of interest is a viable alternative (Mamta et al., 2010; Souchie et al., 2010; Qureshi et al., 2012).
The guanandi (Calophyllum brasiliense Camb. (Clusilaceae) is a species notable because it presents some important properties that are useful for reforestation programs, primarily the ability to grow in flooded environments (Oliveira and Joly, 2010). The species is widely distributed throughout Central and South America. In Brazil, the wide distribution of guanandi ensures its presence in watersheds of several phytoecological regions. Due to its various uses and importance, guanandi is currently being exploited unsustainably and it is susceptible to local extinction (Mendonça et al., 2014). The interaction of beneficial microorganisms such as plant growth promoters and P-solubilizers may favor the propagation and survival of plant species of economic interest (Singh et al., 2011), and this can be applied to guanandi plants.
This study aimed to: a) in solid medium, isolate CaHPO4-solubilizing and non-P-solubilizing microorganisms from the rhizosphere of guanandi collected in a nursery and from the field; b) in liquid medium, quantify the ability of CaHPO4-solubilizing isolates from the rhizosphere of guanandi to solubilize FePO4; c) quantify IAA production by FePO4-solubilizing rhizosphere microorganisms.
Sources of guanandi roots
Guanandi cutting was removed from the forest reserve of the “São Tomaz Olho D´agua” farm at Rio Verde city, Goiás State, Brazil, located at “Sul Goiana” Highway Km 01, Rural Zone, at the coordinates 17°48'1.692" S latitude and 50°53'57.0696"W longitude. One young guanandi plant, with three years old, was collected with its roots preserved. The plant was fully removed from the soil and carefully handled to prevent damage to the roots and to conserve a large amount of the rhizospheric soil. Then, the plant was placed in a styrofoam cooler and transported to the “Laboratório de Microbiologia Agrícola” at “IF Goiano – Câmpus Rio Verde”, where the material was processed within 24 h.
On the same day, another guanandi cutting, grown for 120 days was harvested from the nursery (tubes) for collection of its roots, which were also taken to the laboratory for the isolation of rhizosphere microorganisms.
Isolation of rhizosphere microorganisms
Rhizosphere microorganisms were isolated in GELP (glucose, yeast extract and peptone) with medium supplemented of CaHPO4 (10%), as described by Sylvester-Bradley et al. (1982). In detail, root fragments were carefully collected to ensure the adhering soil was not removed, and 10 g of sample was transferred into 90 ml of sterile saline solution (0.9% NaCl) and stirred for 40 min. The sample was then serially diluted up to 10-5 concentration. Subsequently, 10-4 and 10-5 dilutions were plated in triplicate.
Plates were incubated for 7 days at 28°C in an oven. Plates were inspected daily, bacterial and fungal isolates that exhibited a clear halo around the colony against the opaque medium were counted (indicating the ability to solubilize CaHPO4). These isolates were transferred to Petri dishes containing the same isolation medium and incubated for 4 days at 28°C. As next step, these bacterial and fungal isolates were transferred to penicillin vials containing nutrient agar and potato dextrose agar (PDA) growth media, respectively, and stored in refrigerator.
In vitro determination of phosphate solubilization ability
CaHPO4-solubilizing isolates were cultured in a liquid medium supplemented with FePO4 (1 g/L) according to Gadagi and Sa (2002). For this assay, 200 µL aliquots of pre-inoculum (108 colony-forming units (CFU)/ml) were transferred in triplicate to test tubes containing the appropriate liquid medium and incubated for 7 days at 28°C. After incubation, 1.5 ml aliquot was removed from the cultures for centrifugation at 8000 rpm for 10 min at 4ºC. Then, 1.0 ml of the supernatant was transferred to test tubes, and 1.0 ml of the working reagent [0.4 g of ascorbic acid; 100 ml of solution 725 (1.0 g of bismuth subcarbonate dissolved in 68 ml of sulfuric acid and added to 300 ml of distilled water, combined with 20 g of ammonium molybdate dissolved in 68 ml of sulfuric acid and added to 300 ml of distilled water) and 900 ml of distilled water] was added. After 20 min of reaction, the phosphate solubilization was determined using a spectrophotometer (725 nm) according to Braga and DeFelipo (1974) modified by Reis et al. (2008).
To quantify the solubilization ability, a calibration curve was created with increasing phosphoric acid concentrations, using a stock solution (20 mg ml-1). From this stock solution 0, 100, 200, 300, 500, 700 and 900 µL was added to distilled water for a final volume of 1.0 ml. Subsequently, 1.0 ml of the working reagent was added to all tubes of the standard curve.
Assessment of IAA production by guanandi rhizosphere microorganisms
A modification of the in vitro assay protocol described by Asghar et al. (2002) was established to evaluate the ability to produce IAA by the isolates. Aliquots of 500 µL of pre-inoculum (108 CFU/ml) were inoculated into flasks containing 10 ml of liquid DYGS medium (composition g L-1: 2.0 glucose; 2.0 malic acid; 2.0 yeast extract; 1.5 peptone; 0.5 K2HPO4 3H2O; 0.5 MgSO4 7H2O; 1.5 glutamic acid; pH 6.0) supplemented with 100 µg/ml of L-tryptophan (Sigma-Aldrich®). Samples were incubated in the dark for 48 h at 30°C under agitation at 80 rpm. The control consisted of non-inoculated sterile medium supplemented with L-tryptophan. After the incubation period, cultures were homogenized, 1.5 ml of each were transferred to Eppendorf tubes and centrifuged at 12,000 g for 5 min at 4°C. One milliliter of supernatant was placed in glass tubes in triplicate for each isolate, and 1 ml of Salkowski reagent was added (1 ml of 0.5 M FeCl3.6H2O in 50 ml of 35% HClO4) (Gordon and Werber, 1951). The tubes were agitated, and the solutions incubated for 30 min in the dark to allow the reaction to occur. The presence of IAA in the solution was observed by changing color, which became more intensely pink as increasing IAA amounts. The assessments had their absorbance measured in a UV-visible spectrophotometer (530 nm). The IAA concentration was estimated using a standard curve prepared with synthetic IAA (0.25, 50, 100 and 150 µg/ml, Sigma-Aldrich®) diluted from a standard solution of 300 µg/ml in sterile and non-inoculated DYGS medium.
Data were subjected to an analysis of variance, and the results of the phosphate solubilization and IAA synthesis assays were compared by Scott-Knott test (p≤0.05), using SISVAR statistical software (Ferreira, 2011).
A total of 108 isolates of P-solubilizing microorganisms (PSM) were detected in the rhizosphere of the plant collected from the field; among them, 85 were P-solubilizing bacteria (PSB) and 23 were P-solubilizing fungi (PSF). The reported population densities of P-solubilizing microorganisms are usually very variable. In this work, 85% of the P-solubilizers isolates were PSB (Table 1). It is expected the number of bacteria isolates to be higher than fungal. Generally, P-solubilizing bacteria constitute 1 to 50% of the total microbial population while PSF represent only 0.1 to 0.5% (Zaidi et al., 2009; Sharma et al., 2013). Greater number of non-P-solubilizing microorganisms was detected in the rhizosphere of the plant collected in the field compared with the isolates from the nursery-grown plant (Table 1). PSM were not detected in the rhizosphere of roots collected from the nursery (Table 1). Several biotic and abiotic factors influence the structure and functional diversity of microbial communities from the rhizosphere (Berg and Smalla, 2009), what probably explains the differences in population of P-solubilizing and non-P-solubilizing microorganisms from nursery and field plants.

Fungi and bacteria can improve plant growth and crop protection what make them of great interest for use as inoculants to maximize the production of tree cuttings.Such microorganisms are capable of solubilizing phosphates, which, although present in the soil are unavailable to the plant, as well as producing metabolites as phytohormones, antimicrobials and antibiotics (Souchie et al., 2007; Avis et al., 2008; Babana et al., 2013).
In this study, all the PSB and PSF isolates solubilized P in liquid broth supplemented with FePO4. The highest P content detected was 3.35 and 3.78 mg P ml-1 for bacteria and fungi isolates, respectively (Tables 2 and 3). Studies on phosphate solubilization typically evaluate the ability of both edaphic and endophytic microbiota to solubilize the Ca-P complex, and the results reflect only the environment of alkaline soils (Gadagi and Sa, 2002). Some microorganisms have the capacity to solubilize Fe-P, representing more relevant analysis in the context of tropical soils (Chagas Jr et al., 2010).
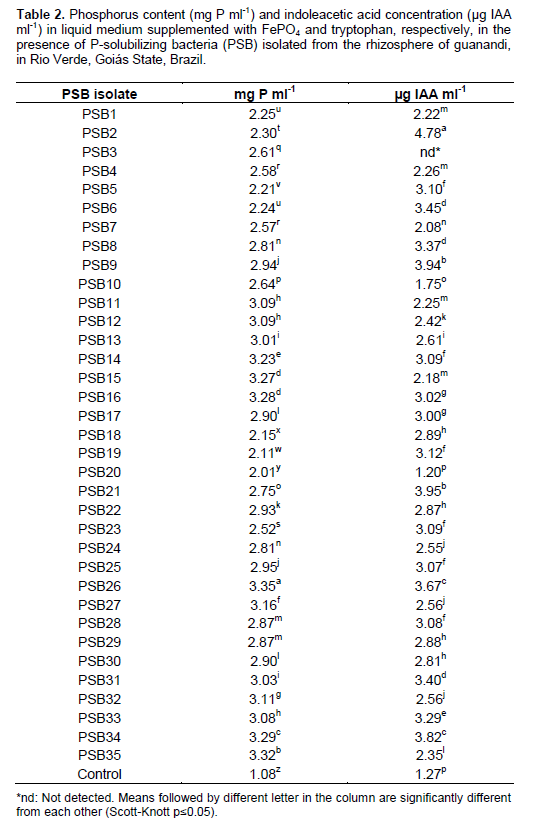
According to Marschner et al. (2011), in the rhizosphere, the mutual demand for Fe and P results in competition between plants and microorganisms with the latter being more competitive due to their ability to decompose plant-derived chelators and their proximity to the root surface;however microbial competitiveness is strongly affected by carbon availability. On the other hand, plants are able to avoid direct competition with microorganisms due to the spatial and temporal variability in the amount and composition of exudates they release into the rhizosphere. Vitorino et al. (2012) suggested, from a biotechnological perspective, screening isolates for their solubilization ability of high levels of FePO4 and abundant IAA production.
Almost all the isolates tested were also able to produce IAA in liquid broth supplement with L-tryptophan (Tables 2 and 3). The results show that the isolates may be selected for inoculation tests aiming to improve guanandi plants growth, especially in nursery. Tsavkelova et al. (2012) reported that IAA and other phytohormones production depend on the stage of colonization, host plant and fungus infection strategy. Altuhaish et al. (2014), reported the existence of plant hormones produced by rhizobacteria that improves growth and development of root cells, to become more extensive absorption, which in turn to increase plant nutrient uptake. Future studies are required to test the ability of microorganisms evaluated in this study in order to improve the growth promotion of guanandi seedlings.
i. The largest population of P-solubilizing and non-P-solubilizing microorganisms was found in the rhizosphere of guanandi cuttings cultivated in the field, and PSM were not detected in the nursery cuttings.
ii. There was no CaHPO4 and FePO4-solubilizing bacterial population in the guanandi cutting grown in the nursery.
iii. The abilities to solubilize FePO4 and produce IAA are variable among isolates of the guanandi rhizosphere.
iv. Two bacterial isolates (PSB3 and PSB20) and two fungal isolates (PSF3 and PSF4) were not able to produce IAA. On average, bacterial isolates produced higher levels of IAA than fungal isolates.
The author has not declared any conflict of interest.
REFERENCES
Ahmad F, Ahmad I, Khan MS (2008). Screening of free-living rhizospheric bacteria for their multiple plant growth promoting activities. Microbiol. Res. 163:173-181.
Crossref
|
|
Altuhaish A, Tjahjoleksono H, Tjahjoleksono A (2014). Biofertilizer effects in combination with different drying system and storage period on growth and production of tomato plant under field conditions. Emir. J. Food Agric. 26:716-722.
|
|
|
Asghar HN, Zahir ZA, Arshad M, Khalik A (2002). Relationship between in vitro production of auxins by rhizobacteria and their growth-promoting activities in Brassica juncea L. Biol. Fertil. Soils 35:231-237.
Crossref
|
|
|
Avis TJ, Gavel V, Antoun H, Tweddell RJ (2008). Multifaceted beneficial effects of rhizosphere microorganisms on plant health and productivity. Soil Biol. Biochem. 40:1733-1740.
Crossref
|
|
|
Babana AH, Dicko AH, Maiga K, Traoré D (2013). Characterization of rock phosphate-solubilizing microorganisms isolated from wheat (Triticum aestivum L.) rhizosphere in Mali. J. Microbiol. Res. 1:1-6.
|
|
|
Berg G, Smalla K (2009). Plant species and soil type cooperatively shape the structure and function of microbial communities in the rhizosphere. FEMS Microbiol Ecol. 68:1-13.
Crossref
|
|
|
Braga JM, Defelipo BV (1974). Determinação espectofotométrica de fósforo em extratos de solos e planta. Ceres 21:73-85.
|
|
|
Caballero-Mellado J, Martínez-Aquilar M, Paredes-Valdez G, Estrada de los Santos P (2004). Burkholderia unamae sp. nov. N2-fixing rhizospheric and endophytic species. Int. J. Syst. Evol. Microbiol. 54:1165-1172.
Crossref
|
|
|
Chagas JR AF, Oliveira LA, Oliveira NA, Willerding AL (2010). Capacidade de solubilização de fosfatos e eficiência simbiótica de rizóbios isolados de solos da Amazônia. Acta Sci. Agron. 32:359-366.
|
|
|
Chen YP, Rekha PD, Arun AB, Shen FT, Lai WA, Young CC (2006). Phosphate solubilizing bacteria from subtropical soil and their tricalcium phosphate solubilizing abilities. Appl. Soil Ecol. 34:33-41.
Crossref
|
|
|
Ferreira DF (2011). Sisvar: a computer statistical analysis system. Cienc. Agrotecnol. 35:1039-1042.
|
|
|
Gadagi RS, Sa T (2002). New isolation method for microorganisms solubilizing iron and aluminum phosphates using dyes. Soil Sci. Plant Nutr. 48:615-618.
Crossref
|
|
|
Gordon SA, Weber RP (1951). Colorimetric estimation of indoleacetic acid. Plant Physiol. 26:192-195.
Crossref
|
|
|
Govindarajan M, Kwon SW, Weon HY (2007). Isolation, molecular characterization and growth-promoting activities of endophytic sugarcane diazotroph Klebsiella sp. GR9. World J. Microbiol. Biotechnol. 23:997-1006.
Crossref
|
|
|
Gyaneshwar P, James EK, Mathan N, Reddy PM, Reinhold-Rurek B, Ladha, JK (2001). Endophytic colonization of rice by a diazotrophic strain of Serratia marcescens. Int. J. Syst. Bacteriol. 183:2634-2645.
Crossref
|
|
|
Hayat R, Ali A, Amara U, Khalid R, Ahmed I (2010). Soil beneficial bacteria and their role in plant growth promotion: a review. Ann. Microbiol. 60:579-598.
Crossref
|
|
|
Luna MF, Galar ML, Aprea J, Molinari ML, Boiardi JL (2010). Colonization of sorghum and heat by seed inoculation with Gluconacetobacter diazotrophicus. Biotechnol. Lett. 32:1071-1076.
Crossref
|
|
|
Luster J, Göttlein A, Nowack B, Sarret G (2009). Sampling, defining characterizing and modeling the rhizosphere- the soil science tool box. Plant Soil 321:457-482.
Crossref
|
|
|
Mamta PR, Pathania V, Gulati A, Singh B, Bhanwra RK, Tewari R (2010). Stimulatory effect of phosphate-solubilizing bacteria on plant growth, stevioside and rebaudioside-A contents of Stevia rebaudiana Bertoni. Appl. Soil Ecol. 46:222-229.
Crossref
|
|
|
Marschner P, Crowley D, Rengel Z (2011). Rhizosphere interactions between microorganisms and plants govern iron and phosphorus acquisition along the root axis – model and research methods. Soil Biol. Biochem. 43:883-894.
Crossref
|
|
|
Medeiros AFA, Polidoro JC, Reis VM (2006). Nitrogen source effect on Gluconacetobacter diazotrophicus colonization of sugarcane (Saccharum spp.). Plant Soil 279:141-152.
Crossref
|
|
|
Mendonça EG, Souza AM, Vieira FA, Estopa RA, Reis, CAF, Carvalho D (2014). Using random amplified polymorphic DNA to assess genetic diversity and structure of natural Calophyllum brasiliense (Clusiaceae) populations in riparian forests. Int. J. Forest. Res. 8p.
|
|
|
Oliveira VC, Joly CA (2010). Flooding tolerance of Calophyllum brasiliense Camb. (Clusiaceae): morphological, physiological and growth responses. Trees 24:185-193.
Crossref
|
|
|
Prieschmann DA, Lehman RM, Christie AA, Dashiell KE (2008). Characterization of bacteria isolated from maize roots: Emphasis on Serratia and infestation with corn rootworms (Chrysomelidae: Diabrotica). Appl. Soil Ecol. 40:417-431.
Crossref
|
|
|
Qureshi MA, Ahmad A, Akhtar N, Iqbal A, Mujeeb F, Shakir A (2012). Role of phosphate solubilizing bacteria (PSB) in enhancing P availability and promoting cotton growth. J. Anim. Plant Sci. 22:204-210.
|
|
|
Reatto A, Correia JR, Spera ST (1998). Solos do bioma cerrado: aspectos pedológicos. In: Sano SM, Almeida SP. Cerrado: ambiente e flora. Planaltina: Embrapa Cerrados. pp. 47-86.
|
|
|
Reis MR, Silva AA, Guimaraes AA, Costa MD, Massenssini AM, Ferreira EA (2008). Ação de herbicidas sobre microrganismos solubilizadores de fosfato inorgânico de solo rizosférico de cana-de-açúcar. Planta Daninha 26:333-341.
Crossref
|
|
|
Resende JCF, Markewitz D, Klink CA, Bustamante MMC, Davidson EA (2010). Phosphorus cycling in a small watershed in the Brazilian Cerrado: impacts of frequent burning. Biogeochemistry 105:105-118.
Crossref
|
|
|
Rodríguez H, Fraga R, Gonzalez T, Bashan Y (2006). Genetics of phosphate solubilization and its potential applications for improving plant growth-promoting bacteria. Plant Soil 287:15-21.
Crossref
|
|
|
Sahin F, Çakmakçi R, Kantar F (2004). Sugar beet and barley yields in relation to inoculation with N2-fixing and phosphate solubilizing bacteria. Plant Soil 265:123-129.
Crossref
|
|
|
Sala VMR, Cardoso EJBN, Garboggini FF, Nogueira NL, Silveira APD (2008). Achromobacter insolitus and Zoogloea ramigera associated with wheat plants (Triticum aestivum). Biol. Fertil. Soils 44:1107-1112.
Crossref
|
|
|
Sharma SB, Sayyed RZ, Trivedi MH, Gobi TA (2013). Phosphate solubilizing microbes: sustainable approach for managing phosphorus deficiency in agricultural soils. Springer Plus 2:587.
Crossref
|
|
|
Singh JS, Pandey VC, Singh DP (2011). Efficient soil microorganisms: a new dimension for sustainable agriculture and environmental development. Agric. Ecosyst. Environ. 140:339-353.
Crossref
|
|
|
Souchie EL, Azcón R, Barea JM, Saggin-Júnior OJ, Silva EMR (2007). Indolacetic acid production by P-solubilizing microorganisms and interaction with arbuscular mycorrhizal fungi. Acta Sci-Biol. Sci. 29:315-320.
|
|
|
Souchie EL, Azcón R, Barea JM, Silva EMR, Saggin-Júnior OJ (2010). Enhancement of clover growth by inoculation of P-solubilizing fungi and arbuscular mycorrhizal fungi. An. Acad. Bras. Cienc. 82:771-777.
Crossref
|
|
|
Sylvester-Bradley R, Askawa N, Latorraca S, Magalhães FMM, Oliveira LA, Pereira RM (1982). Levantamento quantitativo de microrganismos solubilizadores de fosfato na rizosfera de gramíneas e leguminosas forrageiras na Amazônia. Acta Amaz. 12:12-22.
|
|
|
Tsavkelova E, Oeser B, Oren-Young L, Israeli M, Sasson Y, Tudzynski B, Sharon A (2012). Identification and functional characterization of indole-3-acetamide-mediated IAA biosynthesis in plant-associated Fusarium species. Fungal Genet. Biol. 49:48-57.
Crossref
|
|
|
Venieraki A, Dimou M, Pergalis P, Kefalogianni I, Chatzipavlidis I, Katinak ISP (2011). The genetic diversity of culturable nitrogen-fixing bacteria in the rhizosphere of wheat. Microbiol. Ecol. 61:277-285.
Crossref
|
|
|
Vitorino LC, Silva FG, Soares MA, Souchie EL, Costa AC, Lima WC (2012). Solubilization of calcium and iron phosphate and in vitro production of indoleacetic acid by endophytic isolates of Hyptis marrubioides Epling (Lamiaceae). Int. Res. J. Biotechnol. 3:47-54.
|
|
|
Zaidi A, Khan MS, Ahemad M, Oves M, Wani PA (2009). Recent advances in plant growth promotion by phosphate-solubilizing microbes. In: Khan, M. S. et al (eds) Microbial strategies for crop improvement. Springer-Verlag, Berlin Heidelberg. pp. 23-50.
Crossref
|
|