ABSTRACT
To investigate drought-induced changes in morphophysiological characteristics, seedlings of two genotypes of Jatropha curcas (CNPAE 183 and CNPAE 191) were grown under two watering regimes: irrigated (-33.1 to 13.5 to kPa) and water deficit (-409.5 to 49.5 to kPa) for 55 days, followed by six days of rehydration (DAR). Withholding water led to a significant reduction (p<0.05) of leaf water potential (ΨW) and an increase in relative water content (RWC). The values of net photosynthetic rate (PN), stomatal conductance to water vapor (gS) and transpiration (E) were significantly (p<0.05) reduced 21 and 34 days after starting treatment (DAST) in plants of genotypes CNPAE 183 and CNPAE 191, respectively. After 6 DAR, only CNPAE 191 achieved a recovery of PN and E. Moreover, significantly (p<0.05) lower gS was measured in recovering plants of both genotypes, as compared to the controls. Drought stress led to reductions of 57 and 65% in whole-plant hydraulic conductance (KL) in genotypes CNPAE 183 and CNPAE 191, respectively. Full recovery of KL was observed after 6 DAR. The average water consumption was 18% lower in plants subjected to water shortage, as compared to irrigated plants. However, drought-induced reduction in growth led to lower biomass water use efficiency (WUEbiomass) in plants subjected to water deficit. The effect of water stress was more intense in CNPAE 183 than in CNPAE 191, regarding the growth variables (leaf area, height and diameter), dry mass and root volume. Moreover, a delay in the effect of water stress in genotype CNPAE 191 was also observed, which suggests a higher tolerance of this genotype as compared to CNPAE 183. Altogether, the results showed strong drought-induced stomatal limitation of carbon assimilation and growth in J. curcas. Slight genotypic differences were detected, CNPAE 191 being less sensitive to the imposed experimental conditions than CNPAE 183.
Key words: Hydraulic conductivity, root volume, tolerance, leaf gas exchange, water relations, water status.
Jatropha curcas L. (Euphorbiaceae) is an oilseed species, which, despite showing a strong capacity for survival and recovery from water stressed conditions (King et al., 2009; Wang et al., 2011; Verma et al., 2012), has shown negative responses to water deficit in reduced growth and biomass production (Fini et al., 2013; Sapeta et al., 2013). The attractive characteristics of J. curcas include its expected lifespan of 50 years and its broad climatic tolerance, covering zones with annual precipitation between 250 and 1200 mm (Achten et al., 2008). Moreover, production of J. curcas oil does not entail competition with food crops, because its oil is non-edible (Tiwari et al., 2007; Islam et al., 2014) and this is one of the effective ways to overcome the problems associated with energy crisis and environmental issues (Ong et al., 2013). Its seeds may contain 11.7 to 42.1% oil depending on soil type and environmental conditions (Kaushik and Bahrdway, 2013), which makes it very promising for the production of biodiesel (Kheira et al., 2009).
Water is one of the main limiting factors for plant production worldwide. Due to the high economic and ecological costs of irrigation and the need for plant production in increasingly arid environments, the production and use of cultivars adapted to drought is of great importance. One of the more studied mechanisms through which plants can increase water use efficiency (WUE) is the stomatal regulation of water loss by transpiration. However, drought tolerant species with such characteristics tend to exhibit reduced growth rates, due to stomatal limitations to the uptake of CO2 for photosynthesis (Verma et al., 2012).
The use of deficit irrigation has achieved promising results for Cocos nucifera (Arecaceae) (Azevedo et al., 2006) and Citrus latifolia (Rutaceae) (Sampaio et al., 2010) as a tool for increasing WUE. Through deficit irrigation regimes, three strategies could be used to increase WUE: (1) increase the capacity of water absorption; (2) increase the transpiration efficiency by drought-induced signaling and (3) modify the pattern of allocation of assimilates in favor of the economically viable structure (Condon et al., 2004), that is, augmenting the harvest index.
Regardless of some results showing a delay in the growth of J. curcas subjected to water deficit, Fini et al. (2013) concluded that the species can survive dry periods (20% of field capacity) of moderate duration (18 days). However, the effects on the economic profitability of these crops are still unknown. Thus, studies dealing with drought effects on growth and development of different genotypes are likely to be essential components in the success of breeding programs.
This experiment aimed to evaluate the initial growth and estimate the effects of water stress and post-drought recovery on the water relations of two genotypes of J. curcas (CNPAE 183 and CNPAE 191). The main hypothesis is that the effects of water stress on the morphophysiological characteristics of J. curcas vary with time and intensity of stress and are genotype dependent.
Plant material and growth conditions
The experiment was conducted under greenhouse conditions from June to September 2012, in the campus of the State University of Santa Cruz, Ilhéus, BA (14°47'00"S, 39°02'00" W). According to the Köppen climate classification, the local climate is type Af, with annual average temperatures of 22to 25°C (Koppen, 1900).
J. curcas seeds of two genotypes were used, the CNPAE 183 and the CNPAE 191, from Jaíba/MG and São Francisco of Assis/RS, respectively. The selection of these genotypes was based on the differences obtained from preliminary data from EMBRAPA Agroenergia. The CNPAE 183 is a non-toxic genotype with a low yield (500 g of seed plant1), average height of 2.4 m, and is from a tropical region. The CNPAE 191 has a high productivity (1030 g seed plant-1), 3 m of height, is toxic to animals and is from a region of subtropical climate. The J. curcas seeds were germinated in pots containing 65 kg of substrate soil : sand (2:1), to match the loamy sand textural class. Forty days after germination, the pots were covered with aluminum foil to prevent loss of water by evaporation, thereby accounting for the water lost only by leaf transpiration. Then, 22 plants were subjected to a controlled irrigation treatment (60% of field capacity) for 55 days, which led to substrate water deficit (-49.5 to -409.2 kPa) and the other 22 plants to field capacity (-13 1 to -33.1 kPa), followed by rehydration for 6 days.
Water consumption in the two treatments was measured by means of periodic weighing of pots, using load cells CSA/ZL - 100 (MK Control Instruments, Brazil) coupled with an automatic data collector. The determination of the soil water content was carried out weekly by the gravimetric method, and simultaneously the soil matric potential for each treatment was determined using a soil water retention curve. The photosynthetically active radiation (PAR) was monitored using quantum sensors S-LIA-M003. The temperature and relative humidity were recorded using Hobo H8 Pro Series data loggers (Onset, USA). A summary of the microclimatic conditions during the experiment is shown in Table 1.
Water relations
Leaf water potential (ΨW) was measured in three randomly selected leaves per genotype per treatment, using a pressure chamber model 1000 (PMS Instrument Company, USA). The measurements were made between 2 and 4 am (ΨWpredawn) on 35, 50 and 55 DAST and 6 DAR.
Whole plant hydraulic conductivity (KL) was estimated on the peak of water stress (55 DAST) using the formula KL = gS VPD/(ΨWpredawn) – ΨWmidday, where gS is the stomatal conductance to water vapor (see below), VPD is the vapor pressure deficit between the atmosphere and the leaf and ΨWmidday is the water potential measured at midday (Hubbard et al., 1999).
Leaf relative water content (RWC) was measured at 55 DAST and 6 DAR. Measurements were performed between 6 to 7 am. For this purpose, five discs were removed from mature leaves, immediately weighed to obtain fresh mass (Mf) and placed under water in the dark for 12 h until full rehydration. The discs were weighed again to obtain the turgid mass (Mt) and placed in a forced ventilation oven at 75°C until constant dry mass (Md). From these variables, the RWC was calculated as [RWC = ((Mf-Md) / (Mt-Md)) x100] (Nauš et al., 2016).
Leaf gas exchange
Leaf gas exchange variables (net photosynthesis (PN), transpiration rate (E), stomatal conductance to water vapor (gS) and the internal CO2 concentration (CI)) were measured at 21, 28, 34, 41, 49, 55 DAST and 6 DAR in fully mature leaves positioned on the stem opposite to those sampled for leaf water potential, between 7 and 10 h in all individuals of each treatment (De Santana et al., 2015). A portable gas exchange system (LI-6400, LI-Cor®, Nebraska / USA) was used. During the measurements, the LI-6400 was set to hold constant photosynthetically active radiation (PAR) at 1000 μmol photons m-2 s-1 and reference CO2 concentration at 380 μmol mol-1.
Water use efficiencies and water consumption of the plant
Instantaneous and intrinsic water use efficiencies were estimated as the ratios of PN and E and of PN and gS, respectively. Water use efficiency of biomass was calculated as the ratio of the total biomass of the last harvest and water consumed during the experiment, which was measured by sequentially weighting the pots using load cells placed beneath each pot.
Growth
The height, stem diameter, total leaf number and leaf area of all plants were evaluated weekly. Individual leaf area (LA) was estimated from sum of measurements of the length of the midrib (L) and maximum width (W) of each leaf, which were used in the equation LA = (LW)0.9660 suggested by Pompelli et al. (2012). The results were summed to obtain the total leaf area.
Root volume of all plants was measured by displacement of water equivalent units (1 mL = 1 cm3). The length of root systems was also quantified at the end of the experiment by taking the average of the three largest roots. The plants were then collected for the determination of total dry matter of root, stem and leaves, after complete dehydration in a forced ventilation oven (65 ± 5°C).
Statistical analysis and experimental design
The experiment was arranged in a completely randomized factorial (2x2) design, formed by two watering regimes and two genotypes of J. curcas, with six replicates. The data were subjected to a factorial analysis of variance and means were compared by F test (comparison between genotypes and water regimes) with a significance criterion of 0.05.
Water relations
Significant reduction (p<0.05) of ΨWpredawn was observed from 50 DAST in plants of both genotypes, growing under water stress (Figure 1). Full recovery of water status was observed 6 DAR. Hydraulic conductivity (KL) was significantly (P<0.05) lower in stressed plants of CNPAE 183 and CNPAE 191. However, after rehydration there was no significant (p<0.05) difference of KL between the control and stressed plants of either genotype (Figure 2).
Significant differences (p<0.05) between treatments (but not between genotypes) were observed 55 DAST for RWC. RWC was shown to increase in water stressed plants of both genotypes (Figure 3A). After rehydration, RWC decreased in water stressed plants of both genotypes as compared to their control but this decrease was only significant (p<0.05) in CNPAE 191 (Figure 3B).
Leaf gas exchange
Net photosynthetic rate (PN), stomatal conductance to water vapor (gS) and transpiration rate (E) were significantly (p<0.05) reduced in both genotypes by the water stress treatment. Moreover, this reduction was more delayed but greater in magnitude in CNPAE 191 than in CNPAE 183 (Figure 4). Water deficit led to PN, gS and E reductions of 34, 67 and 48% from 21 DAST on for genotype CNPAE 183 and of 49, 74 and 67% from 34 DAST on for CNPAE 191, respectively. After the six-day rehydration period, significant differences (p<0.05) between treatments for gS were still observed in both genotypes (Figure 4C and D).
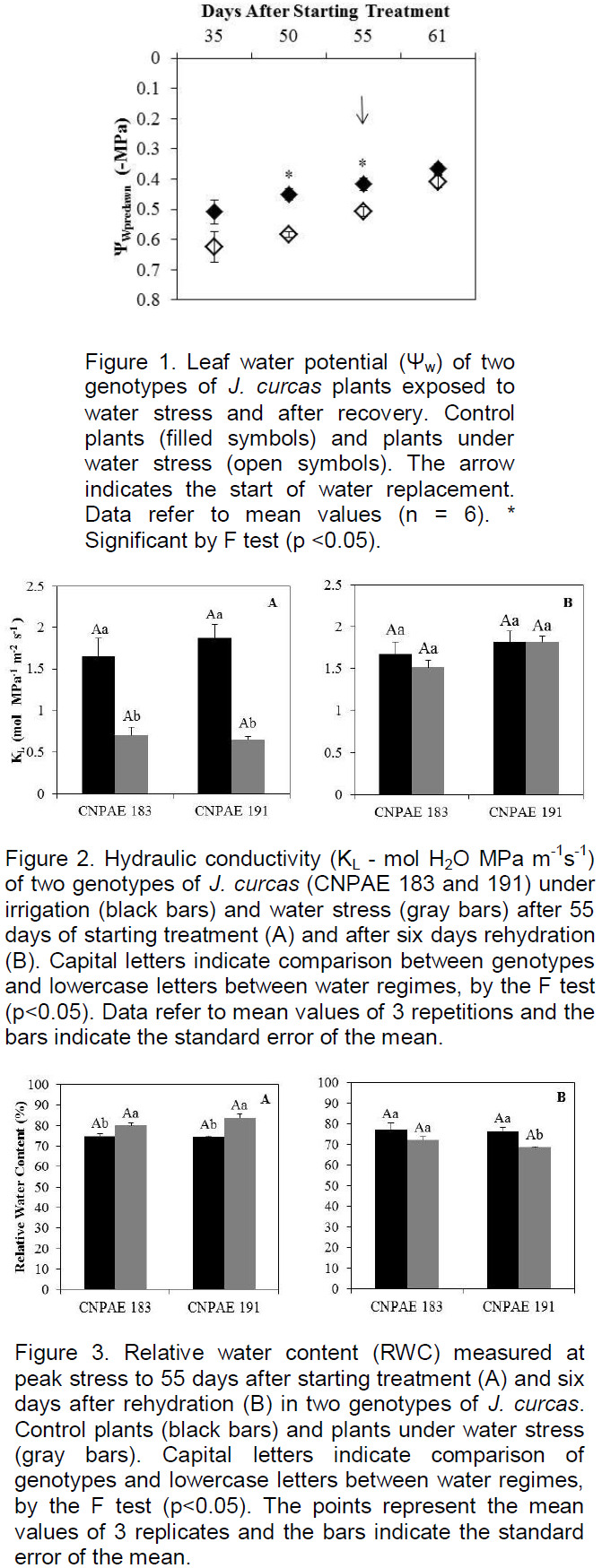
There were no significant differences (P<0.05) between genotypes for the intrinsic (PN/gS) and instantaneous (PN/E) water use efficiencies. While no significant differences were observed for PN/E in CNPAE 183 throughout the experimental period (Figure 5A), a significant (p<0.05) increase (30%) of PN/E was observed from 34 DAST in water stressed plants of CNPAE 191 (Figure 5B). The trend of PN/gS was similar for both genotypes, with a significant increase (p<0.05) from 34 DAST, which peaked at 85 and 96% increases relative to their controls at 55 DAST in CNPAE 183 and 191, respectively (Figure 5C and D). However, both measures of WUE dropped upon rewatering, but reached values comparable to control only in CNPAE 191 at 6 DAR (Figure 5).
Growth
There were significant differences between genotypes and water regimes for the height, number of leaves and leaf area. However, the diameter was affected significantly only for the treatment of water stress (Figure 6). Although, smaller than CNPAE 183 (69 vs. 85 cm in height), CNPAE 191 exhibited a delay in the effects of water stress as indicated by measurements of growth variables. Significant reductions (p < 0.05) in height were observed at 61 and 54 DAST in CNPAE 191 (10%) and CNPAE 183 (30%), respectively (Figure 6A and B). The reduction in diameter was 10% for both genotypes. However, this effect was observed from 41 DAST in CNPAE 183 and from 47 DAST in CNPAE 191 (Figure 6C and D). The number of leaves decreased (24%) significantly (p <0.05) only for CNPAE 183 from 54 DAST (Figure 6E and F).
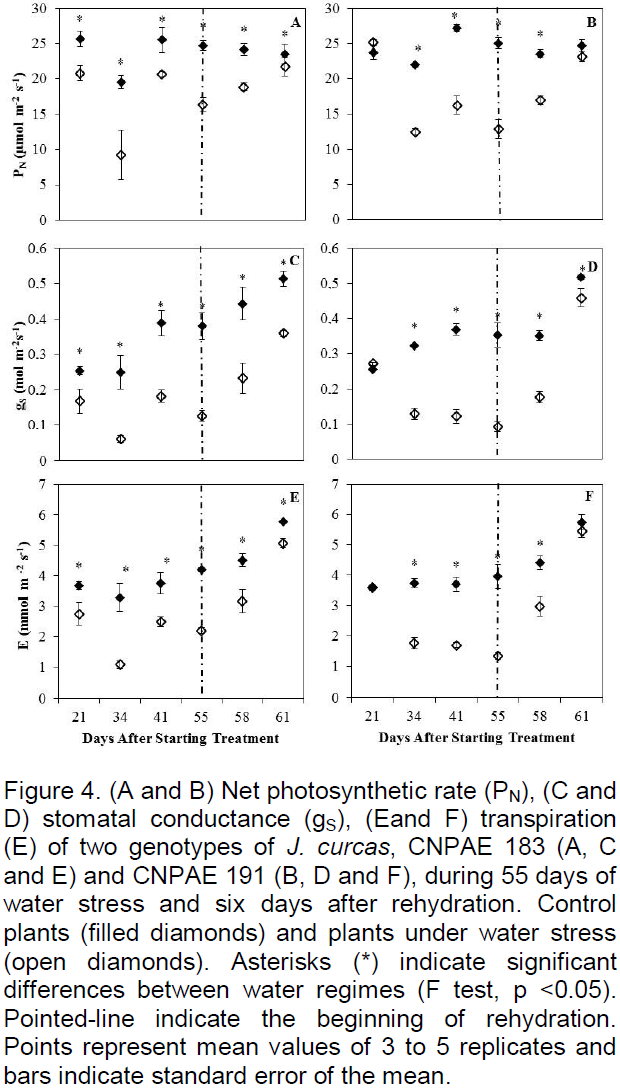
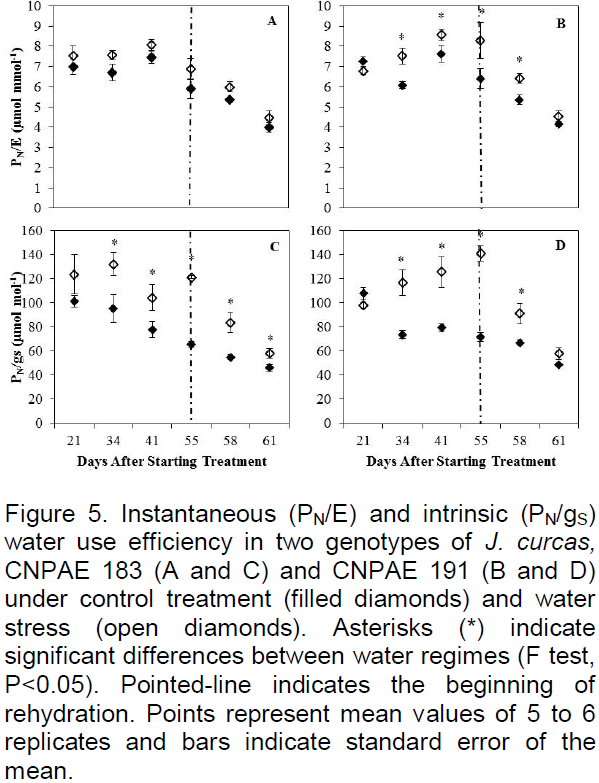
Significant differences (p<0.05) between genotypes under irrigated conditions were also observed for mean leaf area (0.67 m² in CNPAE 183 and 0.45 m² in CNPAE 191). The effect of water deficit occurred earlier (41 DAST) and was more pronounced (45% reduction in relation to control) in CNPAE 183. In CNPAE 191, a significant difference (p<0.05) between the water regimes for leaf area was observed from 47 DAST, with a reduction of 25% relative to the control (Figure 7).
Water stress led to reductions of leaf (LDM) and total (TDM) dry mass in both genotypes, and of root (RDM) and shoot (SDM) dry mass only in CNPAE 183 (Figure 8). The total biomass yield at the end of the experiment was affected by water stress in plants of CNPAE 183, with observed reductions of 29, 50, 78 and 55% of RDM SDM, LDM and TDM, respectively. In CNPAE 191, the observed reductions in dry weights water stressed plants relative to control plants were 70% for LDM and 49% for TDM (Figure 8).
There were no significant differences between genotypes for water consumption. However, water deficit did lead to a reduction in water consumption by the plants (Figure 9A) and, consequently, a reduction in the production of biomass. There was a 20 and 15% reduction in water consumption for genotypes CNPAE 183 and 191, respectively. Nevertheless, water deficit led to a significant (p<0.05) reduction in the biomass water use efficiency in both genotypes (Figure 9B).
The root volume in well-watered plants of CNPAE 191 was significantly (p<0.05) lower than in CNPAE 183.
However, water stress induced significant reduction (25% lower than control) of root volume in CNPAE183 and significant increase of root length in CNPAE 191 (Figure 10). For CNPAE 183, there was no difference between roots lengths of water-stressed and control plants.
Although, J. curcas has been described as being adapted to arid conditions (King et al., 2009; Verma et al., 2012), it is indisputable that under adequate water availability, this species will show higher productivity. However, plants use various strategies to survive the water restriction periods, such as roots and leaves morphological changes, osmotic adjustment, increased abscisic acid content (ABA). The capacity ΨW reduction (osmotic adjustment) is also a common mechanism to avoid dryness in J. curcas, maintaining cell function through high RWC and stomatal closure (Fini et al., 2013; Tiwari et al., 2013; Fang and Xiong, 2014; Silva et al., 2015).
The continuation of drought stress caused no major decrease in ΨW, because as stomata close, there is also a decrease in leaf water loss (Figures 1 and 4), confirming the findings of Fini et al. (2013). These authors also noted that ΨW from plants that were drought stressed matched that of control plants after rehydration, thus demonstrating the species’ ability to fully recover after a period of water stress. Silva et al. (2010), by imposing water stress on plants of J. curcas observed a reduced ΨW in stressed plants as compared to the control, but the relative water content (RWC) was only affected by water stress when the substrate water content was lowered to 10% of field capacity. This led to an increase of RWC as compared to the control. The increase in RWC in this work shows that conservation of the water content in plant tissues can also be considered a strategy of this species to survive periods of water deficit in soil. Results obtained by Sapeta et al. (2013) are similar to those found in this work, with an increase in RWC at the peak of water stress and recovery after 7 days of rehydration. According to Silva et al. (2012) osmotic adjustment is responsible for maintaining a high RWC in J. curcas tissues.
The coordination of hydraulic conductance with several physiological traits has been demonstrated to be linked to water and carbon balances (Brodribb and Jordan, 2008; Brodribb et al., 2010; Martinez-Vilalta et al., 2014; Pivovaroff et al., 2014). Water stress strongly affects the leaf hydraulic system, causing a decline in water potential (Brodribb and Holbrook, 2006). Genotypic differences in J. curcas have been detected recently regarding the trade-offs between KL and growth under moderate water deficit (Santana et al., 2015). The authors demonstrated that three genotypes showed similar reductions in biomass accumulation (about 37%), although one of them (CNPAE 126) showed lower reduction in KL (62% as compared to 88% in the other two genotypes). In the present work, a similar effect of water deficit on biomass accumulation (50% of reduction as compared to their controls) was observed for both genotypes (Figure 8D), although a greater reduction of KL (down to 67% of the control value) was observed in CNPAE 191 than in CNPAE 183 (58%) (Figure 2A).
In a study by Díaz-Lopez et al. (2012), J. curcas showed higher PN/gS after 27 days under deficit irrigation (75% field capacity). This effect was corroborated in our study by 34 DAST for both genotypes. After six days of rehydration, the stressed plants of genotype CNPAE 191 matched the control, which shows once again that these plants decreased gS and E to prevent dehydration under water deficit. Similar results were found by Fini et al. (2013), where the decrease of irrigation resulted in a gain of PN/gS and PN/E, and after 12 days of rehydration plants fully recovered. This shows that J. curcas is able to use physiological mechanisms to survive periods of water deficit, resuming normal physiological function upon return to well-watered conditions.
Water stress reduces photosynthetic rate due to decreased stomatal conductance (Figure 4), through stomatal closure. However, with closed stomata, gas exchange and CO2 assimilation by C3 photosynthesis is negligible (Chaves et al., 2009). This may account for a delay in the growth of the plant.
Verma et al. (2012) found similar patterns in gas exchange when they subjected plants to water stress at 50% of field capacity (FC). Sousa et al. (2012), also using a drought treatment of 50% of FC, found reductions of around 70% of carbon assimilation as compared to the control. Variation in the results of such studies can be attributed to the soil and climatic conditions of each region, pot size, the vapor pressure deficit and temperature, as well as nutritional status and genetic factors that may influence the physiological characteristics of the plant.
After six days of rehydration PN and E recovered to the control values in genotype CNPAE 191. However, gS shown by rehydrated plants remained significantly different from that of control plants. Similar results show that the recovery of gS is slower than PN, because this recovery is linked to the gradual decrease in the concentration of abscisic acid (ABA), and the time required for this to occur depends on the plant species and degree of stress (Pompelli et al., 2010). On the other hand, Silva et al. (2015) recently showed a more rapid restoration (5 days) of gS than of to PN (10 days), suggesting the need for recovery of gS to facilitate the restoration of PN.
Pompelli et al. (2010) reported values of PN lower than 5 µmol CO2 m-2 s-1, when soil water content had reached 5%. However, plants recovered in at least four days, reaching values of PN and gS higher than the control plants, confirming the data presented here, which allows us to infer that these plants controlled dehydration by reducing gS. However, the decrease of gS caused by the closure of the stomata for water conservation entails an unavoidable decrease in CO2 up take, thereby limiting plant productivity.
Water stress causes negative effects on cell expansion and photosynthesis, which causes a reduction in plant growth (Zhu, 2002), as observed in this study. Drought-induced reduction of growth in J. curcas has been considered a bottleneck concerning the potential use of this species as a bioenergy crop in arid and semiarid environments worldwide, where soil water potential may become very low during long time of drought (Fini et al., 2013). Reductions of 50% in the number of leaves for genotypes J. curcas from Brazil and Tanzania and a 90% for genotypes from Suriname, after 18 days without irrigation have been demonstrated (Fini et al., 2013). In this study, drought led to reductions of 22 and 9% in the number of leaves for genotypes CNPAE 183 and CNPAE 191, respectively. However there was no shedding leaf, but a reduction in the emission of new leaves.
Sapeta et al. (2013) demonstrated significant reductions in height and number of leaves of J. curcas from the 7th day of severe water stress imposition. In this study, the lowest number of leaves on the water stressed plants of genotype CNPAE 183 was due to reduced initiation of new leaves, as a mechanism to decrease the surface area for transpiration. However, this mechanism may cause losses in crop yield, because with a reduced leaf area, there is a decrease in light interception, thus decreasing overall photosynthetic capacity. Maes et al. (2009), in subjecting J. curcas to water deficit (40% FC), found reductions in leaf area of approximately 57% as compared to control. Verma et al. (2012), after 50 days of imposing water stress at 75 and 25% of field capacity reported 11 and 55% reductions in leaf area of J. curcas.
The similarity between the RDW of control and stressed plants for CNPAE 191 explains the smaller effect of water stress on the biometric characteristics (height, diameter, number of leaves and leaf area), making a good indicator of drought tolerance (Figure 8). The stressed plants of genotype CNPAE 191, however, as a mechanism to prevent dehydration, increased the length of their roots, to explore a larger volume of soil (Figure 10), which according to Hammer et al. (2009) are characteristics associated with drought resistance. With the reduction in leaf area and maintenance of RDW, there is greater hydration of plant tissue (measured as RWC), favoring the continued growth and development of the plant (Silva et al., 2010). As experimental water stress was not imposed for a long time, the recovery of gs was faster, favoring CO2 entry into the cell, which is an interesting quality for genetic improvement, as it allows to increase the effective use of water (EUW), influencing the plant stress tolerance and avoiding reduced productivity (Blum, 2009).
Moderate and rapidly-imposed water deficit, as imposed here, negatively affect the gas exchange and biomass water use efficiency of J. curcas, despite reductions in water consumption and increased photosynthetic water use efficiency. Maintenance of high RWC and Ψw under water deficit, as well as a lack of genotypic variation in that characteristic indicate that both J. curcas genotypes are water savers. The genotype CNPAE 191 should be considered for further investigation concerning the tradeoffs between RWC and root traits, in the search for genetic material suitable for cultivation in areas subject to short periods of soil water deficit.
The authors have not declared any conflict of interests.
Financial support for the investigation was provided by National Council for Scientific and Technological Development, CNPq (Grants 486218/2011-2) and Fundação de Amparo à Pesquisa do Estado da Bahia – FAPESB (Grants APP1242/2011). The authors were supported by CAPES, CNPq and FAPESB. Fábio P. Gomes gratefully acknowledge the CNPq, Brazil, for the concession of a fellowship of scientific productivity. Finally, the authors thank Jamie Males for his helpful assistance and comments during the preparation of the manuscript.
CI, Internal CO2 concentration; DAR, days after rehydration; DAST, days after starting treatment; DW, dry weight; E, transpiration rate; FC, field capacity; gS, stomatal conductance to water vapour; KL, hydraulic conductivity; LA, leaf area; PAR, photosynthetically active radiation; PN, net photosynthesis; PN/gS, intrinsic water use efficiency; PN/E, instantaneous water use efficiency; RWC, relative water content; Ψw, leaf water potential; WS, water stress conditions; WUE, water use efficiency.
REFERENCES
Achten W, Verchot L, Franken Y, Mathijs E, Singh V, Aerts R, Muys B (2008). Jatropha bio-diesel production and use. Biomass. Bioenerg. 32:1063-1084.
Crossref
|
|
Azevedo P, Sousa I, Silva B, Silva V (2006). Water-use efficiency of dwarf-green coconut (Cocos nucifera L.) orchards in northeast Brazil. Agric. Water Manage. 84:259-264.
Crossref
|
|
|
Blum A (2009). Effective use of water (EUW) and not water-use efficiency (WUE) is the target of crop yeld improvement under drought stress. Field Crop Res. 112:119-123.
Crossref
|
|
|
Brodribb T, Holbrook N (2006). Declining hydraulic efficiency as transpiring leaves desiccate: two types of response. Plant Cell Environ. 29:2205-2215.
Crossref
|
|
|
Brodribb T, Jordan G (2008). Internal coordination between hydraulics and stomatal control in leaves. Plant Cell Environ. 31:1557-1564.
Crossref
|
|
|
Brodribb T, Bowman D, Nichols S, Delzon S, Burlett R (2010). Xylem function and growth rate interact to determine recovery rates after exposure to extreme water deficit. New Phytol. 188:533-542.
Crossref
|
|
|
Chaves M, Flexas J, Pinheiro C (2009). Photosynthesis under drought and salt stress: regulation mechanisms from whole plant to cell. Ann. Bot. London. 103:551-560.
Crossref
|
|
|
Condon A, Richards R, Rebetzke G, Farquhar G (2004). Breeding for high water-use efficiency. J. Exp. Bot. 55:2447-2460.
Crossref
|
|
|
Díaz-López L, Gimeno V, Simón I, Martínez V, Rodríguez-Ortega W, García- Sánchez F (2012). Jatropha curcas seedlings show a water conservation strategy under drought conditions based on decreasing leaf growth and stomatal conductance. Agric. Water Manage. 105:48-56.
Crossref
|
|
|
Fang Y, Xiong L (2015). General mechanisms of drought response and their application in drought resistance improvement in plants. Cell Mol. Life Sci. 72:673-689.
Crossref
|
|
|
Fini A, Bellasio C, Pollastri S, Tattini M, Ferrini F (2013). Water relations, growth, and leaf gas exchange as affected by water stress in Jatropha curcas. J. Arid Environ. 89:21-29.
Crossref
|
|
|
Hammer GL, Dong Z, McLean G, Doherty A, Messina C, Schussler J, Zinselmeier C, Paszkiewicz S, Cooper M (2009). Can changes in canopy and/or root system architecture explain historical maize yield trends in the US corn belt?. Crop Sci. 49:299-312.
Crossref
|
|
|
Hubbard R, Bond B, Ryan M (1999). Evidence that hydraulic conductance limits photosynthesis in old Pinus ponderosa trees. Tree Physiol. 19:165-172.
Crossref
|
|
|
Islam A, Yaakob Z, Ghani J, Anuar N (2014). Jatropha curcas L.: A Future Energy Crop with Enormous Potential. Biomass Bioenerg. Applications, Springer International Publishing Switzerland, 31-61.
|
|
|
Kaushik N, Bhardwaj D (2013). Screening of Jatropha curcas germplasm for oil content and fatty acid composition. Biomass Bioenerg. 58:210-218.
Crossref
|
|
|
Kheira A, Atta N (2009). Response of Jatropha curcas L. to water deficits: Yield, water use efficiency and oilseed characteristics. Biomass Bioenerg. 33:1343-1350.
Crossref
|
|
|
King A, He W, Cuevas J, Freudenberger M, Ramiaramanana D, Graham I (2009). Potential of Jatropha curcas as a source of renewable oil and animal feed. J. Exp. Bot. 60:2897-2905.
Crossref
|
|
|
Köppen W (1900). Attempted climate classification in relation to plant distributions. Geogr. Z. 11:593-611.
|
|
|
Maes W, Achten W, Reubens B, Raes D, Samson R, Muys B (2009). Plant water relationships and growth strategies of Jatropha curcas L. seedlings under different levels of drought stress. J. Arid Environ. 73:877-884.
Crossref
|
|
|
Martínez-Vilalta J, Poyatos R, Aguadé D, Retana J, Mencuccini M (2014). A new look at water transport regulation in plants. New Phytol. 204:105-115.
Crossref
|
|
|
Nauš J, Šmecko S, Špundová M (2016). Chloroplast avoidance movement as a sensitive indicator of relative water content during leaf desiccation in the dark. Photosynth. Res. 129:217-225.
Crossref
|
|
|
Ong H, Silitonga A, Masjuki H, Mahlia T, Chong W, Boosroh M (2013). Production and comparative fuel properties of biodiesel from non-edible oils: Jatropha curcas, Sterculia foetida and Ceiba pentandra. Energ. Convers. Manage. 73:245-255.
Crossref
|
|
|
Pivovaroff A, Sack L, Santiago L (2014). Coordination of stem and leaf hydraulic conductance in southern California shrubs: a test of the hydraulic segmentation hypothesis. New Phytol. 203:842-850.
Crossref
|
|
|
Pompelli M, Antunes W, Ferreira D, Cavalcante P, Wanderley-Filho H, Endres L (2012). Allometric models for non-destructive leaf area estimation of Jatropha curcas. Biomass Bioenerg. 36:77-85.
Crossref
|
|
|
Pompelli M, Barata-Luís R, Vitorino H, Gonçalves E, Rolim E, Santos M, Almeida-Cortez J, Ferreira V, Lemos E, Endres L (2010). Photosynthesis, photoprotection and antioxidant activity of purging nut under drought deficit and recovery. Biomass Bioenerg. 34:1207-1215.
Crossref
|
|
|
De Santana T, Oliveira P, Silva L, Laviola B, Almeida A, Gomes F (2015). Water use efficiency and consumption in different Brazilian genotypes of Jatropha curcas L. subjected to soil water deficit. Biomass Bioenerg. 75:119-125.
Crossref
|
|
|
Sampaio A, Coelho Filho M, Coelho E, Daniel R, Machado V, Carvalho G, Junior E (2010). Deficit hídrico e secamento parcial do sistema radicular em pomar de lima ácida. Pesqui. Agropecu. Bras. 45:1141-1148.
Crossref
|
|
|
Sapeta H, Costa J, Lourenco T, Maroco J, Van der Linde P, Oliveira M (2013). Drought stress response in Jatropha curcas: Growth physiol. Environ. Exp. Bot. 85:76-84.
Crossref
|
|
|
Silva E, Ferreira-Silva S, Viégas R, Silveira J (2010). The role of organic and inorganic solutes in the osmotic adjustment of drought-stressed Jatropha curcas plants. Environ. Exp. Bot. 69:279-285.
Crossref
|
|
|
Silva E, Ribeiro R, Ferreira-Silva S, Vieira S, Ponte L, Silveira J (2012). Coordinate changes in photosynthesis, sugar accumulation and antioxidative enzymes improve the performance of Jatropha curcas plants under drought stress. Biomass Bioenerg. 45:270-279.
Crossref
|
|
|
Silva E, Silveira J, Ribeiro R, Vieira S (2015). Photoprotective function of energy dissipation by thermal processes and photorespiratory mechanisms in Jatropha curcas plants during different intensities of drought and after recovery. Environ. Exp. Bot. 110:36-45.
Crossref
|
|
|
Sousa A, de Lacerda C, Gheyi H, Soares F, Uyeda C (2012). Teores de nutrientes foliares e respostas fisiológicas em pinhão manso submetido a estresse salino e adubação fosfatada. Rev. Caatinga. 25:144-152.
|
|
|
Tiwari A, Kumar A, Raheman H (2007). Biodiesel production from jatropha oil (Jatropha curcas) with high free fatty acids: an optimized process. Biomass Bioenerg. 31:569-575.
Crossref
|
|
|
Tiwari N, Purohit M, Sharma G, Nautiyal A (2013). Changes in Morpho-Physiology of Jatropha curcas grown under different water regimes. Nat. Sci. 11:76-83.
|
|
|
Verma K, Vatsal S, Gupta R (2012). Influence of water application on photosynthesis, growth and biomass characteristics in Jatropha curcas. Curr. Bot. 3:26-30.
|
|
|
Wang W, Li R, Liu B, Li L, Wang S, Chen F (2011). Effects of low nitrogen and drought stresses on proline synthesis of Jatropha curcas seedling. Acta Physiol. Plant. 33:1591-1595.
Crossref
|
|
|
Zhu J (2002). Cell signaling under salt, water and cold stresses. Curr. Opin. Plant Biol. 4:404-406.
|
|