ABSTRACT
The hog plum tree is a species exploited extractively, but this information is not included in official statistics, despite the socioeconomic relevance of the species in the North and Northeast of Brazil. The present study aimed to analyze physiological and biochemical processes in Spondia mombin L. plants under two water regimes. The experiment was conducted in a greenhouse at the Federal Rural University of the Amazon, Belém, PA. The experimental design was completely randomized with two water conditions: control and water deficit, with 20 repetitions totaling 40 experimental units, where each experimental unit consisted of one (1) plant/pot. Moderate/severe water deficit was simulated by suspending irrigation of the seedlings, respectively, for a 15-day period. There were reductions in the levels of water potential, nitrate, nitrate reductase and proteins, while the values for ammonium, glutamine synthetase, amino acids, proline and glycine betaine had a significant increase in plants under water stress compared with control plants. Young hog plum plants under water deficit undergo changes in nitrogen metabolic pathways. These changes are indicative of intolerance to extremely dry environments.
Key words: Nitrate content, osmoregulator, tolerance, amino acid.
The hog plum tree (Spondias mombin L.) is a fruit species, native to tropical America, belonging to the Anacardiaceae family, common in the Amazon region, where it is a wild species. Its fruits are commercialized fresh and can be consumed in the form of juice, ice cream, popsicles, custard and mousse. Important species of the genus Spondias include the hog plum (S. mombin L.), the green mombin (S. tuberosa Arruda), and the golden apple (S. dulcis Parkinson). These species are exploited extractively or in domestic orchards, but this information is not included in official statistics, despite their socioeconomic relevance in the North and Northeast of Brazil (Quadros, 2013).
Hog plums are considered to have good nutritional quality; the fruit has a pleasant aroma and a juicy pulp, as well as a distinctive bittersweet flavor (Silva et al., 2007). In addition, the fruit is composed of compounds such as carotenoids and tannins, representing a good source of this component and playing its most important nutritional role, that is, its activity as provitamin A, thus gaining prominence as a probable natural antioxidant.
More than any other environmental stress, soil water deficit is a serious global problem (Carlin and Santos, 2009). One of the great challenges currently faced by agriculture, therefore, is to increase crop yields in regions that are likely to be under water deficit. In response to the lack of water, plants perform various physiological events; the most frequent one is osmotic adjustment, whereby they adapt to keep water potential and cell turgor at more appropriate levels. Water participates as a reagent in numerous metabolic reactions; lack of water affects all aspects of plant growth and development (Pereira et al., 2012).
Various physiological and biochemical processes, such as gas exchanges between the inner leaf and the atmosphere, photosynthesis and metabolism of carbohydrates, proteins, amino acids and other organic compounds, are modified by water deficit (Silva, 2008). In this context, the aim of this study was to evaluate the metabolism of nitrogenous compounds in young hog plum plants (S. mombin L.) under water deficit conditions.
Experimental setup and location
The experiment was conducted in a greenhouse at the Institute of Agricultural Sciences (ICA) of the Federal Rural University of the Amazon (UFRA), in Belém, PA, Brazil (01° 27’ S and 48° 26’ W). Before the start of treatment, all plants were placed under 50% shade cloth, irrigated daily for a month to keep them at field capacity and acclimatization (Fernandez and Sykes, 1968).
Plants
Young hog plum plants grown from seeds were supplied by AIMEX (Federation of Timber Export Industries from the State of Pará). Hog plum seedlings were transplanted to 10 kg pots with substrate containing a mixture composed of black soil, manure and earthworm humus at a ratio of 3:1:1 (v:v:v), respectively, on a 0.02 m layer of crushed stones to facilitate soil drainage. Before transplanting, tests were made to check the field capacity of the pots; liming was performed to correct soil pH, and macro and micronutrients were supplemented, based on chemical soil analysis, by applying 600 ml of the nutrient solution of Hoagland and Arnon (1950), modified at the Laboratory of Biodiversity Studies on Higher Plants (EBPS), UFRA.
Experimental design
The experimental design was completely randomized with two water conditions: control and water deficit, with 20 repetitions totaling 40 experimental units, where each experimental unit consisted of one (1) plant/pot.
Statistics
Analysis of variance was applied to the results and when there was significant difference, the means were compared by Tukey’s test at 5% significance level. Moreover, standard deviations were calculated for each treatment, and statistical analyses were performed with the software program (SAS-Institute, 1996).
Plant training
A preliminary experiment was performed to simulate moderate/ severe water deficit by suspending irrigation on the seedlings, respectively, for 15 days. During the experimental period, the control plants were watered daily to replace water loss. Watering was performed individually for each pot, taking into account daily weighings, forming a set (pot + plant + soil); weed control was also made, weed from manual weeding without causing nutritional deficiency, pests and pathogens. The soil was sieved. Then pots were filled and the average weight of the vessels determined when the soil was at field capacity. The average weight of the plants in clod was also made from each treatment. So when planting each seedling in a respective vessel, the average weight of each pot at field capacity was known. The vessels were weighed at every turn of irrigation, and the average weight determined with the soil in the current humidity. Following irrigation, the weight of a vase with irrigated soil was made to be the same, thus maintaining the field capacity. This procedure was determined following the recommendations of Melo et al., (1998).
Preedawn water potential
Predawn water potential (Ψam) was determined between 4:30 and 5:30 am, by means of a pressure pump type Scholander (M670, PMS Instrument Co., Albany, USA) as described by Pinheiro et al., (2007).
Determination of gas exchange
The stomatal condutance to water vapor (gs) and the transpiration (E) were determined by a portable porometer of dynamics balance (mod. Li 1600, LiCor, Nebraska, USA). The measurements were made at 9:00 a.m. As samples, mature leaflets, completely expanded, were selected from leaves of second or third pair counted starting from the apex. After gas exchange, leaf samples were collected and immediately taken to a forced air circulation glasshouse at 65°C until drought for the flour preparation.
Determination of the biochemical variables
Determination of the concentrations of nitrate
Fifty (50 mg) were weighed of previously lyophilized leaves, adding total extract, salicylic acid 5% solution in concentrated sulfuric acid. The concentration of nitrate was obtained from a standard curve with increasing concentrations of NO3- (0, 0.5, 1.0, 2.0, 3.0, 4.0 and 5.0 μmol ml-1) according the method described by Cataldo et al., (1975).
Determination of nitrate reductase activity
200 mg were weighed of leaf disks of 0.5 cm diameter placing the samples in test tubes containing phosphate buffer isopropanol from a standard curve obtained with KNO2 p.a. (Sigma) according the method in vivo recommended by Hageman and Hucklesby (1971).
Free ammonium concentrations
Fifty (50 mg) were weighed of lyophilised leaves previously, adding in the test tubes the total extract, solution A and solution B after shaking. The free ammonium concentrations were estimated from the standard curve constructed with (NH4)2SO4 p.a. (Sigma) according to the method described by Weatherburn (1967).
Total soluble amino acid concentrations
Fifty (50 mg) were weighed of previously lyophilised leaves, by adding the total extract, buffered solution and reagent ninhydrin. The total free amino acid levels were determined based on a standard curve adjusted from increasing concentrations of a standard mixture of L-glutamine according to the method described by Peoples et al., (1989).
Determination of proline levels
Fifty (50 mg) were weighed of lyophilised leaves previously by adding in the test tubes total extract, ninhydrin acid and glacial acetic acid. It was determined through a calibration curve proline and proline results expressed in mmol g-1 dry matter (DM) were determined according to Bates et al., (1973).
Glycine betaine content determination
Twenty five (25 mg) were weighed of lyophilised leaves previously by adding H2SO4 2 N test tubes and KI-I2 iced. A standard curve was used and Glycine – Betaine was determined by the method described by Grieve and Grattan (1983).
Glutamine synthetase activity
It was determined by the “in vitro” method of Kamachi et al., (1991). The results were expressed in mmol of γ-glutamyl-hydroxamate kg1- DM of tissue h-1.
Determination of total soluble proteins concentration
The concentrations of total soluble proteins were determined by method of Bradford (1976). The results were expressed in mg protein g-1 DM.
Water potential
The results showed significant reduction in water potential (Ѱw); the results ranged from -0.28 MPa (control plants) to -2.855 MPa (plants under water deficit) (Figure 1). This represents a decrease of 919% of the water potential compared with control plants. Water potential was decreased in plants under water stress and this may be due to biochemical changes that lead to changes in cell juice concentration. However, it may also have resulted from the decrease in leaf cell volume by increasing water deficit in the soil, which prevents water supply to plants (Pallardy, 2008). This may also have occurred because the leaf transpiration rate is greater than the absorption capacity (roots) or greater than the transportation capacity throughout the trunk and branches of trees (Marenco et al., 2014). Similarly, Fernandes et al., (2015) found -2.2 MPa when working with eucalyptus plants grown in a greenhouse under water stress. Lima et al., (2015), during a 25-day experiment, found that water deficit caused a significant reduction in water potential in andiroba plants, reaching levels near -2.00 MPa, while the irrigated plants obtained values of - 0.08 MPa during the experiment.
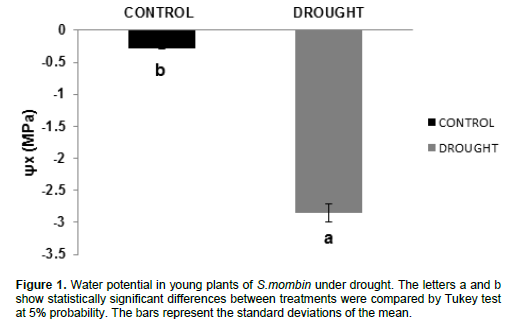
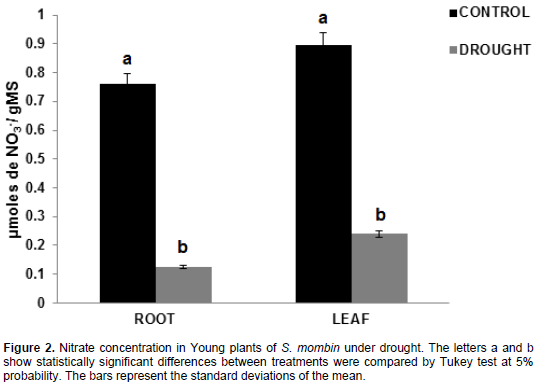
Nitrate concentration
The results obtained in hog plum plants revealed that there was a significant reduction in nitrate concentration in the roots and leaves under irrigation suspension (Figure 2). For the roots, the values ranged between 0.76 and 0.12 µmoles NO3-/g DM for control plants and plants under water deficit, accounting for a decrease by 83.64%. By contrast, the values were 0.89 and 0.24 µmoles NO3-/g DM in the leaves of control plants and plants under water deficit, with a decrease by 73.25%, respectively.
Ammonium and nitrate are the main forms of nitrogen available to plants. In the processes of reduction and assimilation, nitrogen can be absorbed both in the leaves and in the roots simultaneously or between these organs. They are, therefore, essential processes for plants because they control their growth and development (Shan et al., 2012). Thus, nitrate is not only a source of nitrogen supply, but also a marker for various cellular
processes (Lemos et al., 2008).
For nitrate to be taken up by the roots, it has to be diluted with water present in the soil. However, nitrate concentration was reduced during water deficit, as a possible response to low absorption of nitrate and due to high retention of the soil solution caused by water deficit as well as low concentration of NO3- in the soil (Nobre et al., 2010). Entry of nitrate into the plant is a process involving multiple biochemical reactions, and it consumes high amounts of energy. Water deficiency decreased nutrient absorption, which provides the lowest root to shoot transport, because osmotic adjustment in the leaves occurs more slowly (Suassuna et al., 2012). As a result, there is a low shoot growth, that is, cell division was inhibited by stomatal closure, thus reducing perspiration and consequently affecting the process of photosynthesis (Galon et al., 2010).
A study conducted by Ananthi and Vijayaraghavan (2012) on cotton under water deficit, showed that the latter caused a reduction in the activity of the nitrate reductase enzyme (Figure 3), which leads to low nitrate concentration; thus, productivity was remarkably reduced when water stress was imposed, with flowering being the most sensitive stage in this process.
Nitrate reductase activity
The results obtained in hog plum plants revealed that the activity of the nitrate reductase enzyme was significantly reduced in the roots and the leaves under water deficit (Figure 3). For the root, the values were 0.33 and 0.03 µmoles NO2- /g MF/h for control plants and plants under water deficit, which represents a decrease by 89.51%. For the leaves, the values were 0.21 and 0.06 µmoles NO2-/g MF/h control plants and plants under water stress, that is, a decrease by 72.17%, respectively.
Nitrate is the main form of nitrogen assimilated by plants and its absence is the major factor limiting plant growth. For this nutrient to be absorbed by plants, an enzyme goes into action; particularly, nitrate reductase, which is a physiological indicator of the effect of the association between them in an environment under water stress (Rhein et al., 2011).
According to Valduga and Finzer (2010), when a plant has more limited access to water available in the soil for its physiological activities, there is a control in stomatal closure that will occur according to the current amount of water. In other words, if there is little water available, guard cells will not be turgid; there will be lower water potential, thereby leading to a low release of water to the environment. With this mechanism, the plant can use water more efficiently. However, as water becomes a limiting factor in stomatal closure, there is a decrease in photosynthesis and in the nitrate reductase enzyme (Marenco et al., 2014). When there are small decreases in water potential, water tends to respond quickly and decrease dramatically. A previous study by Castro et al., (2008) reported a decrease in nitrate reductase activity as a result of water deficit in Teak plants.
Another possible inhibition is the occurrence of a high synthesis-degradation ratio that, in turn, is highly dependent on its substrate, since the enzyme was induced by it (Oliveira et al., 2011). Another explanation for this reduction is the high amount of energy required by the process; as photosynthesis is consequently reduced, the energy resulting from this process will be reduced, causing this enzyme to restrict its activities (Soares et al., 2011).
A study carried out by Souza et al. (2008) showed data on hog plum plants, indicating that the nitrate reductase enzyme had lower activity in the leaves. This may have been a strategy used by the plants to lose their leaves during the dry season or during water stress. A similar result was found by Melo et al., (2014) on Coffea arabica seedlings, where nitrate reductase activity was reduced in the leaves under water deficit. Similar results were observed in another study with Açai; results indicated that, nitrate reductase activity was decreased under water deficit conditions (Freitas et al., 2008). On the contrary, Oliveira et al. (2005) found that the peach palm species showed greater reductase activity in the leaves.
Ammonium
In plants under water deficit, free ammonium concen-tration was increased by 15.48 mmol NH4+/kg DM and 8.98 mmol NH4+/kg DM compared to control plants -12.37 mmol NH4+/kg DM and 5.38 mmol NH4+/kg DM - for the root and leaf tissues, corresponding to a percentage of 25.14 and 66.91%, respectively (Figure 4). This accumulation of ammonium either in the root and in the leaves may stem from protein degradation, as can be seen in Figure 5. The high ammonium concentration may have lowered regeneration as a result of its toxic effect. The toxic effect of ammonium on tissues can be due to pH changes and acidification of the environment or toxic effect of free ammonium levels (Mukeshimana and Kelly, 2013).
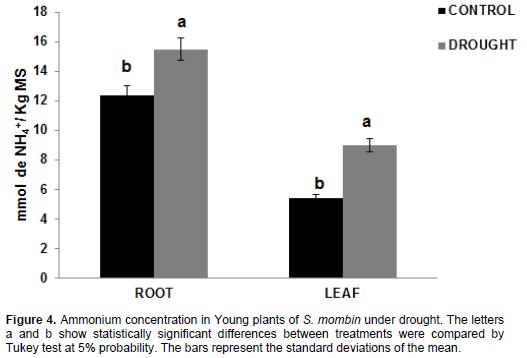
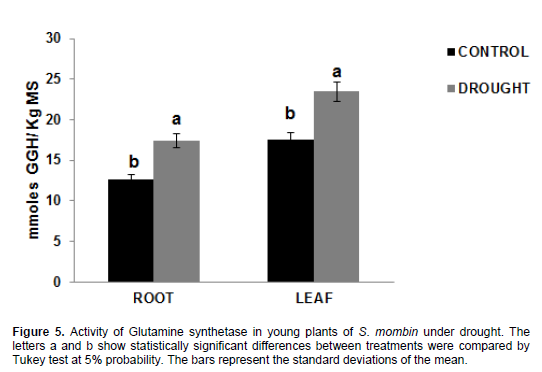
Ammonium is assimilated by the enzymes glutamine synthetase (GS) and glutamate 2-oxoglutarate aminotransferase (GOGAT), which form the GS/GOGAT cycle, or by the enzyme glutamate dehydrogenase (GDH), which participates in an alternative pathway and performs reversible catalysis for the amination of glutamate (Masclaux-Daubresse et al., 2006). Glutamate dehydrogenase (GDH) can also metabolize ammonium, especially under conditions where there is excess ammonium. However, some authors reported that this enzyme is less important than GS, and GOGAT (Shridhar, 2012).
Genetic breeding studies are focused on finding cultivars and varieties that are more resistant to environmental adversities, through genotypes with lower free ammonium accumulation in the tissue and a higher activity of the glutamine synthetase enzyme, thereby avoiding the toxicity of this ion.
Glutamine synthetase
Fifteen days after the beginning of the experiment, there was an increase in the activity of the glutamine synthetase enzyme in the range of 17.46 and 23.48 mmol GGH/kg DM in plants under water stress when compared to control plants -12.58 and 17.49 GGH mmol/kg DM for root and leaves, respectively (Figure 5). It can be seen that, even under water stress, the plants maintained the activity of this enzyme, then moving onto free ammonium incorporation in the formation of amino acids, as evidenced by the information in Figure 6. A glutamine synthetase (GS) converts ammonium into amino acids, thereby preventing its excessive accumulation, which could lead to toxicity and inhibition of biological nitrogen fixation (Prell and Poole, 2006; Bernard and Habash, 2009). This can be observed through ammonium accumulation and high GS activity in the present study.
In order to keep nitrogen balance, glutamine synthetase may be located in tissues and organs involved in the generation and transport of reduced nitrogen (Miflin and Habash, 2002). The substrate used for GOGAT, glutamine, did not limit the reaction, because there is still a high GS activity even in plants under water
stress.
In the present study, and also in the study by Masclaux-Daubresse et al., (2006), who worked with tobacco plants, GS activity was higher in the leaves than in the roots, which is probably associated with primary and secondary N assimilation. By contrast, Oliveira Neto et al. (2009) found different results when working with sorghum plants under water stress.
Amino acids
The total soluble amino acid concentrations showed a significant increase. The values were 43.38 and 59.59 µmol AA.g-1 M in roots and leaves, respectively (Figure 6) for the control plants and 65.65 and 87.49 µmol AA.g-1 M in roots and leaves, respectively, for plants under water stress. The total soluble amino acid content was increased by 51.34% in the roots and 46.82% in the leaves when under water stress for 15 days. In the present study, the amino acid accumulation recorded can be explained by the possible inactivation of protein synthesis. Another factor that may be considered for the results is the fact that proteolytic enzymes degrade proteins by breaking peptide bonds, thus increasing free amino acid content (Sousa et al., 2015). These results are in agreement with those obtained by Rivas et al. (2013) who found a significant increase in amino acid levels when working with Moringa oleifera plants under water deficit for 10 days. Moura (2010) who studied Jatropha curcas plants, found opposite results for total soluble amino acid content when the plants were under water deficit when such stress was more intense.
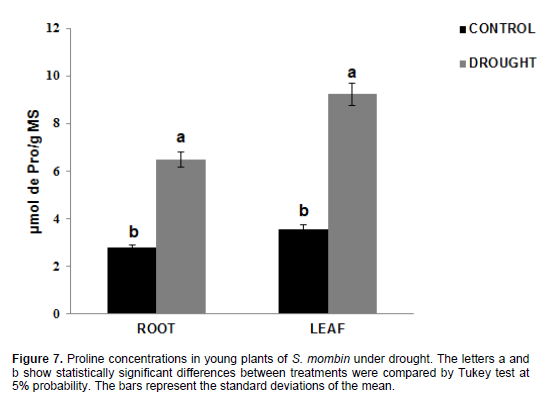
Proline
There was a significant increase in proline levels (Figure 7), which were 2.76 μmol pro/g DM in the roots and 3.56 μmol pro/g DM in the leaves for control plants, while the values for the plants under water stress were 6.47 μmol pro/g DM in the roots and 9.23 μmol pro/g DM in the leaves. There was an increase in proline content by 134.42% in the roots and 159.27% in the leaves when the plants were under water deficit. Lisar et al., (2012) showed that proline accumulation cannot be considered as a resistance factor but an indicator of tolerance as a result of osmotic adjustment by the plant when exposed to water stress. However, proline accumulation brings several advantages in addition to osmotic adjustment. This molecule can avoid damage to cell membranes and prevent protein denaturation by forming some types of reactive oxygen species (ROS), especially hydroxyl radicals (Verbruggen and Hermans, 2008). This is due to the fact that NADPH is used during proline synthesis (Jortzik et al., 2010). This finding was confirmed by Amorim et al., (2011) who researched proline content in Anacardium occidentale leaves under water deficit, and found an increase in this solute in response to such stress. Husen (2010), when working with two different teak clones, found significant increase in proline content by 82.09 and 87.09% after 20 days under water deficit.
Glycine betaine
The reported amounts of glycine betaine (Figure 8) in the leaves of plants under water stress were 12.48 mg/g DM, which represents an increase of 171.8% compared to the irrigated plants (4.59 mg/g DM), while the values found in non-irrigated roots were 10.76 mg/g DM, which accounts for 198.8% compared to the control treatment (3.6 mg/g DM). The increased levels of glycine betaine are associated with the fact that it is an effective osmotic adjuster in plant species. It is tolerant to various types of abiotic stress and its properties suggest promising strategies for developing stress-tolerant plants (Wani et al., 2013). Glycine betaine is involved in osmotic adjustment, protection of cellular structures and antioxidant protection (Silva et al., 2010). Therefore, the accumulation of this solute may have contributed to the protection of plants in the harsh conditions of osmotic potential in soil; it can act as an osmolyte and maintain water balance between the plant cell and the environment. This increase in glycine concentration was observed in Jatropha curcas plants in the treatments with irrigation and non-irrigation after 35 days of water stress (Sousa et al., 2012). However, there was no significant change in glycine betaine concentration in young plants of Khaya ivorensis A. Chev. under water deficit (Albuquerque et al., 2013).
Proteins
The values for total soluble protein levels (Figure 9) in plants under water stress were 0.031 mg protein/g DM in the roots and 0.0948 mg protein/g DM in the leaves, with a considerable decrease compared to control plants, whose values were 0.06767 mg protein/g DM in the roots and 0.2135 mg protein/g DM in the leaves. Thus, there was a decrease of 45.81% in the roots and 44.03% in the leaves. According to Maraghni et al., (2011), protein metabolism was significantly affected by water deficit, yet protein biosynthesis is one of the first metabolic events paralyzed after the perception of stress. Proteolysis then starts; it is an event that promotes an increase in soluble amino acid content, with consequent reduction of protein concentration (Brito et al., 2008). These results corroborate those found by Lechinoski et al. (2007) while working with Teak plants; therefore, water deficit reduces total soluble protein concentrations in the tissues of hog plum plants, probably as a result of increased activity of proteolytic enzymes that break down reserve proteins while reducing their synthesis. Opposite results were reported by Viana et al., (2014) when working with Eucalyptus urophylla plants after 20-day exposure to water stress.
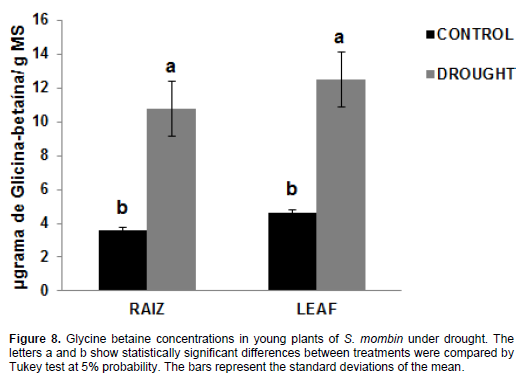
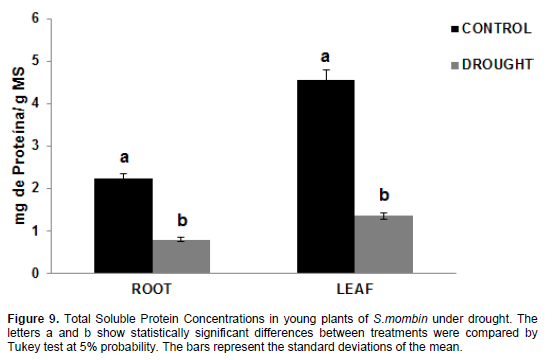
The metabolismo of taperebá plants altered nitrogen metabolism when subjected to drought, reducing the water potential, nitrate concentration, and protein concentration of nitrate reductase activity, and increasing concentration of proline, glycine betaine, amino acids, ammonium and glutamine synthetase activity. There is still a need for further research to precede this study, especially as concerns the use of more varieties of this species, the genre Spondia, which are diverse. Therefore, studies that address the physiology, biochemistry and antioxidant enzymes in such species, to indicate varieties more resistant or tolerant to water stress are necessary.
The authors have not declared any conflict of interests.
REFERENCES
Albuquerque MPF, Moraes FKC, Santos RIN, Castro GLE, Ramos EMLS, Pinheiro HA (2013). Eco physiology of young plants of African mahogany subjected to water deficit and rehydration. Braz. Agric. Res. Brasília 48(1):9-16.
|
|
Amorim AV, Gomes-Filho E, Bezerra MA, Prisco JT, de Lacerda CF (2011). Production and physiology of dwarf cashew plants under conditions of drought and irrigated. J. Agric. Environ. Eng. Campina Grande, 15(10):1014-1020.
|
|
|
Ananthi K, Vijayaraghavan H (2012). Soluble Protein, Nitrate Reductase Activity and Yield Responses in Cotton Genotypes under Water Stress. Insight Biochem, 2:1-4.
Crossref
|
|
|
Bates LS, Waldren RP, Teare ID (1976). Rapid determination of free proline for Bradford, MM. A rapid and sensitive method for the quantitation of microgram quantities of protein utilizing the principle of protein-dye binding. Anal. Biochem. 72:248-254.
Crossref
|
|
|
Bernard SM, Habash DZ (2009). The importance of cytosolic glutamine synthetase in nitrogen assimilation and recycling. New Phytol. 182:608-620.
Crossref
|
|
|
Bradford MM (1976). A rapid and sensitive method for the quantitation of microgram quantities of protein utilizing the principle of protein-dye binding. Anal. Biochem. 72:248-254.
Crossref
|
|
|
Brito LKFL de, Silveira JAG, Lima LLF de, Timóteo AR De S, Chagas RM, Macedo CEC de (2008). Changes in nitrogen fractions profile in sugarcane calluses induced by water deficit. Br. Agric. Res. Bras. 43(6):683-690.
|
|
|
Carlin SD, Santos DMM (2009). Physiological indicators of the interaction between drought and soil acidity in sugarcane. Braz. Agric. Res. Bras.4 4(9):1106-1113.
|
|
|
Castro DS, Lobato AKS, Mendes FS, Oliveira neto CF, Cunha RLM, Costa RCL (2008). Nitrate reductase activity in leaves of teak (Tectona grandis L. f) under water deficit. Braz. J. Biosci. 5(S2):936-938.
|
|
|
Cataldo DA, Haroon SLE, Yougs VL (1975). Rapid colorimetric determination of nitrate in plant tissue by nitration of salicylic acid. Commun. Soil Sci. Plant Anal. 6:(1):71-80.
Crossref
|
|
|
Fernandes ET, Cairo PAR, Novaes AB de (2015). Physiological responses of eucalyptus clones grown in a greenhouse under water deficit. Rural Sci. Santa Maria 45(1):29-34.
|
|
|
Freitas JMN, Da Silva CK, Da Silva LK, Da Silva Castro, Da Maia PSP, Oliveira NCF, Da Costa RCL (2008). Nitrate reductase activity, relative water content and total soluble chlorophyll content in leaves of açai (Euterpe edulis Mart.) Submitted to drought and flooding. Braz. J. Biosci. 5(S2):924.
|
|
|
Galon L, Tironi SP, Rocha AA, Soares ER, Concenço G, Alberto CM (2010). Influence of abiotic factors on productivity of maize. Tropica magazine: Agric. Biol. Sci. 4:3.
|
|
|
Grieve CM, Grattan SR (1983). Rapid assay for determination of water soluble quaternary ammonium compounds. Plant Soil 70:303-307.
Crossref
|
|
|
Hageman RHG, Hucklesby DP (1971). Nitrate reductase from higher plants. In: Meths. Enzymol. 17A:491-503.
Crossref
|
|
|
Hoagland DR, Arnon DI (1950). The water culture method of growing plants without soil. Berkeley: University of California, P 32.
|
|
|
Husen A (2010). Growth characteristics, physiological and metabolic responses of teak (Tectona grandis Linn. f.) clones differing in rejuvenation capacity subjected to drought stress. Silvae Genet. Berlin 59:2-3.
|
|
|
Jortzik E, Fritz-Wolf K, Stum N, Hipp M, Rahlfs S, Becker K (2010). Redox regulation of Plasmodium falciparum ornithine δ-aminotransferase. J. Mol. Biol. 402:445-459.
Crossref
|
|
|
Kamachi K, Yamaya T, Mae T, Ojima KA (1991). Role for glutamine synthetase in remobilization of leaf nitrogen during natural senescence in rice leaves. Plant Physiol. 96:411-417.
Crossref
|
|
|
Lechinoski A, Freitas JMN, Castro DS, Lobato AKS, Oliveira NCF, Cunha RLM (2007). Influence of water stress on protein contents and total soluble amino acids in leaves of teak (Tectona grandis L. f.). Braz. J. Biosci. Porto Alegre, 5:927-929.
|
|
|
Lemos OL, Almeida O, Guedes P, Rebouças TNH, Seno S (2008). Relation between nitrogen metabolism and photosynthesis in fruit formation: a literature review. Dialogues & Science, 7.
|
|
|
Lima EG, Oliveira TB, Conceição SS, Ataide WL, Maltarolo BM, Nogueira GA, Oliveira NCF, Costa RCL, Okumura RS (2015). Biochemical and physiological responses of andiroba (Carapa guianensis Aubl.) seedlings subjected to water déficit. Aust. J. Crop Sci. 9(6):517-522.
|
|
|
Lisar SYS, Motafakkerazad R, Hossain MM, Rahman IMM (2012). Water stress in plants: Causes, effects and responses. In: Water Stress, Edited by: Ismail MMR, Hiroshi H. Rijeka, Croatia: In Technol. pp. 1-14.
|
|
|
Maraghni M, Gorai M, Neffati M (2011). The Influence of Water-Deficit Stress on Growth, Water Relations and Solute Accumulation in Wild Jujube (Ziziphus lotus). J. Ornam. Hortic. Plants 1(2):63-72.
|
|
|
Marenco RA, Vera-Antezana SA, Gouvêa PR, Camargo MAB, Oliveira MF, Santos JK (2014). Physiology of forest species of the Amazon: photosynthesis, respiration and water relations, Ceres Magazine, Viçosa, 61:786-799.
|
|
|
Masclaux Daubresse C, Reisdorf Cren M, Pageau K, Lelandais M, Grandjean O, Kronenberger J, Valadier M, Feraud M, Jouglet T, Suzuki A (2006). Glutamine synthetase glutamate synthase pathway and glutamate dehydrogenase play distinct roles in the sink-source nitrogen cycle in tobacco. Plant Physiol. 140:444-456.
Crossref
|
|
|
Melo WJ de, Melo GMP de, Bertipaglia LMA, Melo VP de (1998). Experimentation under controlled conditions. Jaboticabal: Funep, P 82.
|
|
|
Melo EF, Brum CNF, Pereira FJ, Castro EM de, Chalfun-Júnior A (2014). Anatomic and physiological modifications in seedlings of Coffea arabica cultivar Siriema under drought conditions. Ciênc. Agrotecnol. 38(1):25-33.
Crossref
|
|
|
Miflin BJ, Habash DZ (2002). The role of glutamine synthetase and glutamate dehydrogenase in nitrogen assimilation and possibilities for improvement in the nitrogen utilization of crops. J. Exp. Bot. 53(370):979-987.
Crossref
|
|
|
Moura AR de (2010). Morphological, physiological and biochemical aspects of pinhão manso (Jatropha curcas L.) submitted to water deficit. 81f. Dissertation (Master of Forest Science) - Rural Federal University of Pernambuco, Recife.
|
|
|
Mukeshimana G, Kelly JD (2013). Influence of basal salt sources on regeneration of common bean. A volunteer organization and informal to make the exchange of information and materials. Annual report of the, Bean Improv. Coop. 56:1-2.
|
|
|
Nobre RG, Gheyi HR, Correia KG, Soares FAL, Andrade LO de (2010). Growth and flowering of sunflower under salt stress and nitrogen fertilizer. Agron. Sci. Mag. 4(3):358-365.
|
|
|
Oliveira Neto CF, Lobato AKS, Costa RCL, Maia WJMS, Santos FBG, Alves GAR, Brinez B, Neves HKB, Santos LMJ, Cruz FJR (2009). Nitrogen compounds and enzyme activities in sorghum induced to water deficit during three stages. Plant Soil Environ. 55(6):238-244.
|
|
|
Oliveira LJde, Mariano-da-Silva S, Netto APC, Silva SM da, Mariano-da-Silva FMdeS (2011). Agronomic characteristics and nitrate reductase activity in Campomanesia sp. plants under water stress. Agrarian Mag. 4(11):43-53.
|
|
|
Oliveira MAJ De, Bovi MLA, Machado EC, Rodrigues JD (2005). Nitrate reductase activity in peach palm seedlings (Bactris gasipaes). Rural Sci. Santa Maria 35(3):515-522.
|
|
|
Pallardy SG (2008). Physiology of woody plants. 3rd ed. San Diego: Elsevier/Academic, 454 pp.
Crossref
|
|
|
Peoples MB, Faizah AW, Reakasem BE, Herridge, DF (1989) Methods for evaluating nitrogen fixation by nodulated legumes in the field. Australian Centre for International Agricultural Research Canberra. 76 pp.
|
|
|
Pereira JW de L, Melo FPde A, Albuquerque MB, Nogueira RJMC, Santos RC (2012). Biochemical changes in peanut genotypes subjected to moderate water deficit. Agronomic Sci. Mag. 43(4):766-773.
|
|
|
Pinheiro HA, Silva JV, Endres L, Ferreira VM, Câmara CA, Cabral FF, Oliveira JF, Carvalho LWT, Santos JM, Santos Filho BG (2007). Leaf gas exchange, chloroplastic pigments and dry matter accumulation in castor bean (Ricinus communis L.) seedlings subjected to salt stress conditions. Ind. Crops Prod. 27:385-392.
Crossref
|
|
|
Prell J, Poole P (2006). Metabolic changes of rhizobia in legume nodules. Trends Microbiol. 14(4):161e168.
|
|
|
Quadros BR (2013). Conservation of taperebá seeds (Spondias mombin l., Anacardiaceae). Thesis (Doctorate degree of Agronomy - Agriculture) - Faculty of Agricultural Sciences at Unesp, 50 f, Botucatu.
|
|
|
Rhein AdeL, Santos DMM, Carlin SD (2011). Reductase enzyme activity of nitrate and free proline content in sugarcane roots under water stress and soil acid. Semina: Agric. Sci. 32(4):1345-1360.
|
|
|
Rivas R, Oliveira MT, Santos MG (2013). Tree cycles of water déficit from seed to Young plants of Moringa oleifera Woody species improves stress tolerance. Plant Physiol. Biochem. 63:200-208.
Crossref
|
|
|
SAS/STAT (1996). User's Guide: version 6.12, SAS Institute, Cary, NC.
|
|
|
Shan AYKV, Oliveira EM de, Bonome LTdaS, Mesquita AC (2012). Metabolic nitrogen assimilation in rubber tree seedlings grown with nitrate or ammonium. Braz. Agropec. Res. Bras. 47(6):754-762.
Crossref
|
|
|
Shridhar BS (2012). Review: nitrogen fixing microorganisms. Int. J. Microbiol. Res. 3(1):46-52.
|
|
|
Silva EC da (2008). Physiological responses of umbuzeiro (Spondias tuberosa Arruda) to water and salt stress. Thesis (Doctorate degree of Botanic) - Rural Federal University of Pernambuco, 142 f, Recife, Pernambuco, 2008.
|
|
|
Silva EN da, Ferreira-Silva SL, Viégas RA, Silveira JAG (2010). The role of organic and inorganic solutes in the osmotic adjustment of drought-stressed Jatropha curcas plants. Environ. Exp. Bot. 69:279-285.
Crossref
|
|
|
Silva YC, Mata MERMC, Duarte MEM, Cavalcanti ASRRM, Oliveira CCA, Guedes MA (2007). Sensory analysis pulp and hog plum juice obtained by rehydration of cajá powder. J. Agro-Industrial Prod. Camp. Gd. 9(1):1-6.
|
|
|
Soares LHS, Fagan EB, Casaroli D, Andrade DM, Soares AL, Martins KV, Rocha FJ (2011). Application of different estrobilurinas in soybean cultivation. FZVA Mag. 18(1).
|
|
|
Sousa AEC, Silveira JAG, Gheyi HR, Lima NMC, Lacerda CF de, Soares FALA (2012). Gas exchange and content of carbohydrates and nitrogen compounds in irrigated pinhão manso with wastewater and saline water. Braz. Agropec. Res. Brasília 47(10):1428-1435..
|
|
|
Sousa CCM, Pedrosa EMR, Rolim MM, de Oliveira Filho RA, de Souza MALM, Pereira Filho JV (2015). Growth and enzymatic responses in caupi beans under water stress and nematode galls. J. Eng. Agric. Environ. 19(2):113-118.
|
|
|
Souza FXC, José TA, Raimundo LN de (2008). Morphological and phenological characteristics of cajazeira clones grown in the Apodi Plateau, Ceará. Agron. Sci. Mag. 37(2):208-215.
|
|
|
Suassuna JF, Fernandes PD, Nascimento Rdo, Oliveira ACM de, Brito KSA, Melo AS de (2012). Biomass production in citrus genotypes subjected to drought in the formation of the rootstock. J. Agric. Environ. Eng. Campina Grande 16(12):1305-1313.
|
|
|
Valduga AT, Finzer J (2010). Use of renewable natural resource ilex paraguariensis st. hil. Progeny Cambona-4-Aspects of Heat Transfer. Fazu In Review, N. 05.
|
|
|
Verbruggen N, Hermans C (2008). Proline accumulation in plants: A review. Amino Acids 35:753-759.
Crossref
|
|
|
Viana J de OF, Oliveira LS de, Antonelli P de O, Batagin-Piotto KD, Brondani GE, Gonçalves AN, Almeida M de (2014). Selection of Eucalyptus urophylla cell lineages for tolerance to hydric and thermal stress. Advances in Forestry Science, Cuiabá 1(4):107-112.
|
|
|
Wani SH, Singh NB, Haribhushan A, Mir JI (2013). Compatible Solute Engineering in Plants for Abiotic Stress Tolerance - Role of Glycine Betaine. Curr. Genom. 14(3):157-165.
Crossref
|
|
|
Weatherburn MW (1967). Phenol hipochlorite reaction for determination of ammonia. Anal. Chem. 39:971-974.
Crossref
|
|