ABSTRACT
Bacillus thuringiensis is a Gram-positive bacterium naturally found in soil, water and grain dust. B. thuringiensis has been used for decades as biological control for pests of economical and medical importance. The aim of this study was to test different growth media for the optimal production of vegetative insecticidal protein (Vip3) by B. thuringiensis recovered from the local environment. The local B. thuringiensis was grown under shaker conditions in commercially based media and commonly known growth based media to produce Vip3. The optimal C/N ratio for Vip3 production (3.88) was achieved with 20 g/l of glucose as carbon source and 15 g/l of soybean as nitrogen source. Different combinations of carbon and nitrogen sources were examined. Glucose and soybean were the best carbon and nitrogen source, respectively, for maximal Vip3 production. Terrific Broth supplemented with 20 g/l glucose was found to yield optimum growth significantly (P <0.01, t-test) after 12, 14, 16, 18 and 20 h compared to a formulated medium with optimized C/N ratio of 3.88. The highest vegetative cell count was achieved after 22 h, thus indicating a higher production of Vip3 protein. The method described in this study will facilitate the production of biopesticides in a cost-effective manner.
Key words: Bacillus thuringiensis, bioinsecticides, Vip3, culture media, vegetative protein.
Resistance to inorganic insecticides by many insect species and the ecological damage caused by the toxic effects of insecticides has provided the impetus to seek alternative methods for insect control. This has led to the development of bioinsecticides, which are insecticides using living organisms or their extracts. Bioinsecticides based on the insecticidal action of Bacillus thuringiensis are the most successful and environmental-harmless, commercial and natural alternative to inorganic insecticides (Osman et al., 2015; Siegwart et al., 2015) accounting for 90% of all biopesticides sold worldwide. B. thuringiensis is a Gram-positive spore-forming bacterium found naturally in soil, water, dead insects, and grain dust. This bacterium produces crystalline protein inclusions or crystals during sporulation and/or stationary growth phase. The insecticidal host range of B. thuringiensis includes Lepidoptera, Coleoptera, Diptera, Acarina, Hymenoptera, protozoa, trematodes, and nematodes (Osman et al., 2015; Nair et al., 2018). Intensive screening programs have yielded new genes that effectively produce vegetative insecticidal proteins (Vip). These are groups of insecticidal proteins that represent the second generation of insecticidal trans-genes, which will complement the novel δ-endotoxins in the future (Osman et al., 2015, 2018; Abulreesh et al., 2012). The Vip3 proteins have been reported to have broad-spectrum insecticidal properties (Osman et al., 2018; Wang et al., 2018).
Vip3 toxins exhibit broad-spectrum insecticidal activity against Lepidoptera despite that some of the insecticidal crystal proteins (ICPs) cannot display insecticidal activity against certain insects, such as Agrotis ipsilon (Abulreesh et al., 2012; Osman et al., 2018; Yu et al., 2012). Meanwhile, Vip3A transgenic crops have been developed, e.g., “corn MIR162” and cotton “COT102”, which should be valuable for resistance management (Burkness et al., 2010). It is possible for susceptible pests to develop resistance against B. thuringiensis due to its long-term use. The Vip3 proteins are suitable candidates in B. thuringiensis resistance management as these proteins recognize different receptors than those of Cry proteins (Osman et al., 2018). The homologs of the vip3A gene have been detected in approximately 15% of Bacillus strains analyzed (Estruch et al., 1996). The Vip3A proteins showed activity against lepidopteran insect larvae including black cutworm (A. ipsilon), fall armyworm (Spodoptera frugiperda), beet armyworm (Spodoptera exigua), tobacco budworm (Heliothis virescens) and corn earworm (Helicoverpa zea) (Osman et al., 2018, 2019).
It is believed that the higher production costs of commercial bioinsecticidal products of B. thuringiensis may, in part, hinder these products from being readily available for consumers at a cheaper price. Researchers worldwide have been developing culture media from cheap source material and optimizing growth conditions for cost-effective large scale growth of B. thuringiensis that subsequently yields large scale quantities of insecticidal proteins. The use of coconut cake with mineral salt showed higher production of Cry toxin by B. thuringiensis subsp. israelensis (Poopathi and Archana, 2012a). The use of corn steep liquor and glucose gave higher spore production of B. thuringiensis, thus higher delta endotoxin production (Ernandes et al., 2013). Zou et al. (2016), found that the use of food waste in 75% water content, 2% salt content, less than 5% oil content and pH 7, increased the production of delta endotoxin by B. thuringiensis chicken feather with coconut cake (Poopathi and Archana, 2012b), cactus extract in seawater (Hasanain, 2017) and wheat bran (Marzban, 2012) gave promising results in higher delta endotoxin production. Other researcher optimized currently available culture media such as Luria-Bertani broth for increased production of B. thuringiensis delta endotoxin (Valicente et al., 2010). Other studies evaluated specific nutrient sources such as soybean (Zribi et al., 2018; Navarro-Mtz et al., 2018) and common sugars (Poopathi and Kumar, 2003) as potential nitrogen and carbon sources for optimal growth of B. thuringiensis and subsequently maximal production of insecticidal proteins. The majority of the studies of developing an optimizing growth media for B. thuringiensis production were tested by examining the delta endotoxin production. However, studies concerning the optimization of culture media for the production of Vip toxins are sparse.
In this study, we attempted to evaluate the best carbon and nitrogen sources for optimal growth of B. thuringiensis, as well as the optimal C/N ratio for maximal production of insecticidal Vip3 protein. An attempt was also made to evaluate and optimize different culture media and growth conditions for the optimal growth and subsequent maximal production of Vip3 toxin. Thus the aim of this study was to evaluate different growth media and condition for the cost-effective, optimal production of vegetative insecticidal protein (Vip3) by B. thuringiensis recovered from the local environment.
Isolation of B. thuringiensis from soil and dead larvae
A total of 25 soil samples were collected from different geographical locations of Egypt; Al-Ayat at Giza province, Assiut and Tanta cities. Soil samples were collected from 2 to 5 cm below the ground surface using a shovel. Each soil sample was placed in a plastic bag at ambient temperature. A total of 5 dead larvae of the Egyptian cotton leaf worm Spodoptera littoralis (Boisd.) (Lepidoptera: Noctuidae) was collected from the Giza province. Each dead larva was picked using sterile forceps and placed in a sterile plastic screw-cap bottle. All samples were transferred to the laboratory in an ice box and away from direct sunlight, microbiological examinations begun on the same day of sampling. B. thuringiensis isolates were recovered from soil and insect samples following the method described by Assaeedi et al. (2011).
Identification of B. thuringiensis isolates
B. thuringiensis presumptive isolates were preserved at the Department of Microbial Molecular Biology, Agricultural and Genetic Engineering Research Institute (AGERI), Egypt. The isolates were identified using biochemical methods and 16S rRNA gene sequencing as previously described (EL-Ghareeb et al., 2012; Osman et al., 2013). One isolate was used throughout this study which is registered in the GenBank as B. thuringiensis entomocidus HD110 (accession number JF811911).
Inoculum preparation
Inocula were prepared by growing B. thuringiensis in Luria-Bertani Broth (LB) medium at 28 to 30°C for 24 h. The growth was measured at OD600 and the absorbance was adjusted to a value of 0.9.
Vegetative cell count
Briefly, 100 μl of an overnight grown culture of B. thuringiensis was added to 900 μl of sterile water and mixed thoroughly. Then, l00 μl of this suspension was transferred to 900 μl of sterile water and mix thoroughly. The serial dilution was repeated until a dilution of 1010 was obtained. This is called the dilution factor. Each dilution was plated on LB agar medium and all the plates were incubated at 30°C for 48 h. The plates that showed 30 to 300 colonies are ideal for counting. Five plates were selected and the average CFUs per plate were counted. The number of viable cells per milliliter of the sample was calculated by multiplying the average number of colonies counted per plate by the reciprocal of the dilution factor (Bapat et al., 2006).
Determination of the optimum C/N ratio for the vegetative insecticidal protein (Vip3) production by B. thuringiensis
Five different media containing glucose (carbon source) and soybean meal (nitrogen source) were tested to select the optimum C/N ratio for Vip3 production media composition (Table 1). Other components such as K2HPO4, MgSO4.7H2O, MnSO4.4H2O, FeSO4.7H2O, CaCl2.9 H2O and CaCO3 were added to the growth media and finally, the pH was adjusted to 7.2 before sterilization. Flasks with 50 ml of each medium were inoculated with 1.0 ml of inoculum, incubated at 30°C, and agitated at 200 rpm for 12, 14, 18, and 24 h.
Determination of the optimum carbon source for the vegetative insecticidal protein (Vip3) production by Bt
Five different media containing different carbon sources (glucose, fructose, sucrose, lactose, and corn starch) were examined for the optimum carbon source for Vip3 production (Table 2). Soybean meal was used as the nitrogen source and other components such as K2HPO4, MgSO4.7H2O, MnSO4.4H2O, FeSO4.7H2O, CaCl2.9 H2O and CaCO3 were added to the medium. Finally, the pH of the medium was adjusted to 7.2 before sterilization. Flasks with 50 ml of each medium were inoculated with 1.0 ml of inoculum, incubated at 30°C, and agitated at 200 rpm for 12, 14, 18 and 24 h.
Determination of the optimum nitrogen source for the vegetative insecticidal protein (Vip3) production by B. thuringiensis
Three different media with different nitrogen sources (soybean meal, ammonium sulfate, and urea) were evaluated for the optimum nitrogen source for Vip3 production (Table 3). Glucose was used as the carbon source and other components such as K2HPO4, MgSO4.7H2O, MnSO4.4H2O, FeSO4.7H2O, CaCl2.9H2O, and CaCO3 were added to the growth media and finally, the pH was adjusted to 7.2 before sterilization. Flasks with 50 ml of each medium were inoculated with 1.0 ml of inoculum, incubated at 30°C, and agitated at 200 rpm for 12, 14, 18 and 24 h.
Evaluation of common growth media for optimal production of (Vip3) protein
Luria-Bertani Broth
Luria-Bertani (LB) medium (Estruch et al., 1996) was prepared by dissolving tryptone 10.0 g, yeast extract 5.0 g, sodium chloride 5.0 g in 1 L of deionized water. The final pH of the medium was adjusted to 7.0. Flasks with 50 ml of the medium were inoculated with 1.0 ml of inoculum, incubated at 30°C, and agitated at 200 rpm for 12, 14, 18 and 24 h.
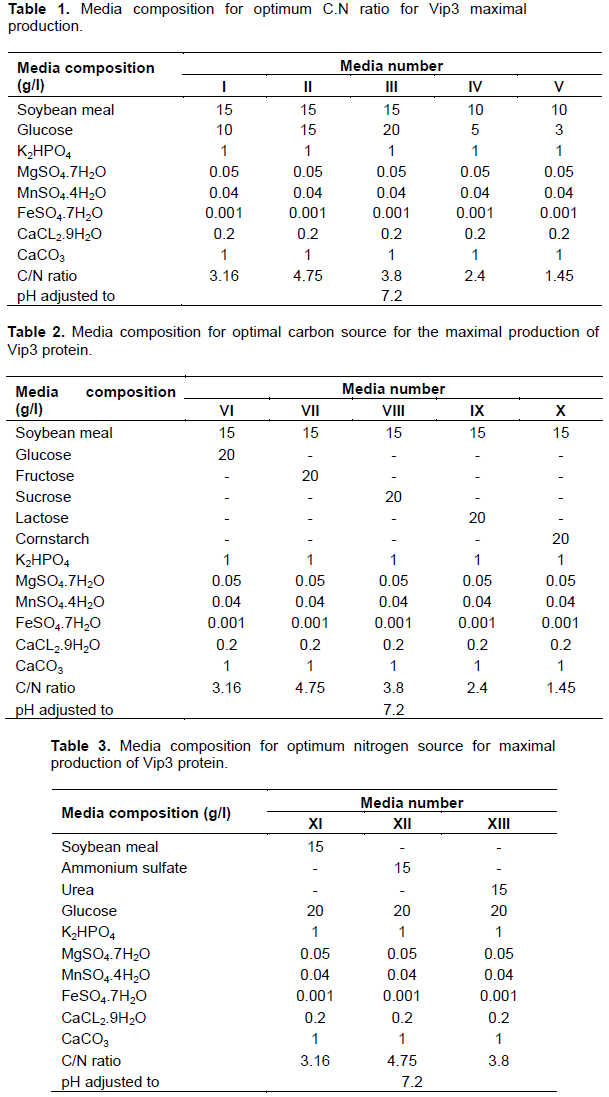
Terrific Broth
Terrific Broth (TB) medium (Estruch et al., 1996), was prepared by dissolving tryptone (12.0 g), yeast extract (24.0 g) and glycerol (4 ml) in 1 L of deionized water. After autoclaving, 100 ml of the filter-sterilized solution of 0.17 M KH2PO4 and 0.72 M K2HPO4 was added. Flasks with 50 ml of the medium were inoculated with 1.0 ml of inoculum, incubated at 30°C, and agitated at 200 rpm for 12, 14, 18 and 24 h.
Evaluating the effect of Terrific Broth supplemented with various concentrations of glucose on the production of Vip3 protein
This medium was prepared by adding different concentrations of glucose (5, 10, 20, 25 and 30 g/l) to the earlier-described formula of terrific broth. Flasks with 50 ml of each medium were inoculated with 1.0 ml of inoculum, incubated at 30°C, and agitated at 200 rpm for 12, 14, 18 and 24 h.
Comparison between formulated and common growth media for the Vip3 production by B. thuringiensis
Terrific Broth supplemented with 20 g/l glucose as the common growth medium (medium 3) and the formulated medium (No. III) were compared to select the optimum medium for Vip3 production by B. thuringiensis. Flasks were filled with 50 ml of each medium and sterilized by autoclaving at 120°C for 20 min. Each flask was inoculated with 1.0 ml of inoculum, incubated at 30°C, and agitated at 200 rpm for 24 h.
Glucose analysis
Glucose was analyzed using the Glucose (GOD-PAP) Vitro Scient kit (Vitro Scient, Cairo, Egypt). Each sample was mixed with the R2 buffer (phosphate buffer 100 mmol l-1, phenol 10 mmol l-1, 4-aminoantipyrine 0.3 mmol l-1, glucose oxidase 1000 U l-1 and peroxidase 700 U l-1) incubation was at 37°C for 10 min or 15 min at 20 to 25°C. The absorption spectrum was measured from 500 to 550 nm.
Bioassay
The bioassay was performed by growing the bacteria overnight in LB broth at 30°C, the growth was measured at OD600 and the absorbance was adjusted to a value of 0.9, to ensure that the bacteria do not enter the sporulation phase whereby delta endotoxin is produced, an OD equal to 0.9 ensures that the bacteria are in the vegetative growth stage by which Vip proteins are produced (Osman et al., 2015). The bioassay was performed by applying each inoculated medium samples on the top of a semi-artificial insect diet. Ten larvae (3 replicates) of the first instars of A. ipsilon were placed on each cup (5.5 cm in diameter and 3.3 cm in height), and a 100-µl aliquot of the toxin was tested. Incubation was performed at 26°C for 3 days as described earlier by Levinson and Navon (1969) and mortality was scored daily.
Statistical analysis
A nonparametric t-test was used to compare the vegetative cell counts between Luria-Bertani Broth and Terrific Broth, the same test was used to compare the vegetative cell counts between formulated medium no. III and Terrific Broth.
Determination of the optimum C/N ratio for the vegetative insecticidal protein (Vip3) production by B. thuringiensis
The final pH values of all 5-tested media ranged from 5.9 up to 6.6 after 18 h. After 24 h, the pH was decreased to 5.4 in medium II, 5.9 in medium I, and to 5.7 in medium III (Figure 1A). The highest vegetative cell count was observed in medium III (9.5 ×1010 CFU) and it became decreased to 4 × 1010 CFU in medium V after 24 h (Figure 1B). The highest mortality (100%) was recorded after 24 h in medium III, while medium V gave the lowest mortality (40%) and the lowest vegetative cell count 4 × 1010 CFU after 24 h (Figure 1C). In this respect, medium III that contained 20 g/l glucose and 15 g/l soybean (C/N ratio = 3.88) seemed to be the choice for optimum production of Vip3 proteins.
Determination of the optimum carbon source for the vegetative insecticidal protein (Vip3) production by B. thuringiensis
The pH values ranged from 5.4 to 6.5 for all 5 tested media after 24 h (Figure 2A). Medium VI (containing glucose) gave the highest vegetative cell count of 9.2×1010 CFU after 24 h, while medium X, which contained corn starch as a carbon source, gave the lowest vegetative cell count 5.1×1010 CFU after 24 h (Figure 2B). Medium VI containing glucose as a carbon source gave the highest mortality rate (100%) after 24 h. While medium VII, which contained fructose, gave a mortality rate of 85% after 14 up to 24 h. Medium VIII and IX, which contained sucrose and lactose, gave 70% mortality rate after 24 h, while medium X, which contained cornstarch, gave 50% mortality rate after 24 h (Figure 2C). The results indicated that glucose is the optimum carbon source for maximal production of Vip3 proteins.
Determination of the optimum nitrogen source for the vegetative insecticidal protein (Vip3) production by B. thuringiensis
The pH values ranged from 5 to 6.6 at all the 3 media after 24h (Figure 3A). Medium XI which contained soybean meal as the nitrogen source gave the highest vegetative cell count of 9.4 ×1010 CFU after 24 h, while medium XIII containing urea as the nitrogen source gave the lowest vegetative cell count of 6.5 ×1010 CFU after 24 h (Figure 3B). Medium XI gave the highest mortality rate (100%) after 24 h at the medium containing soybean meal as the nitrogen source; whereas medium XII, which contained ammonium sulfate as the nitrogen source, gave the mortality rate of 70% after 18 h and decreased to 60% after 24 h. The medium XIII gave 70% mortality after 24 h (Figure 3C). In general, soybean gave optimum results for the production of Vip3 proteins.
Evaluation of common growth media for optimal production of (Vip3) protein
The effect of using Luria-Bertani Broth and Terrific Broth for the growth of B. thuringiensis was expressed by changes in pH, vegetative count, and mortality. Obtained results showed that the pH of the 2 media was within the range and showed no bacterial mortality (Figure 4A and B and Table 4). However, the vegetative cell count reached its maximum only after 14 h in Terrific Broth with a 100% mortality rate (Figure 4B and Table 4). While in LB, the vegetative cell count reached the maximum after 24 h with an 80% mortality rate (Figure 4A and Table 4). Figure 4A and B and Table 4 show that the Terrific Broth was significantly (P ?0.01, t-test) better than the LB medium, which may be due to the presence of a higher amount of glucose (5, 10, 20, 25 and 30 g/l) in the Terrific Broth.
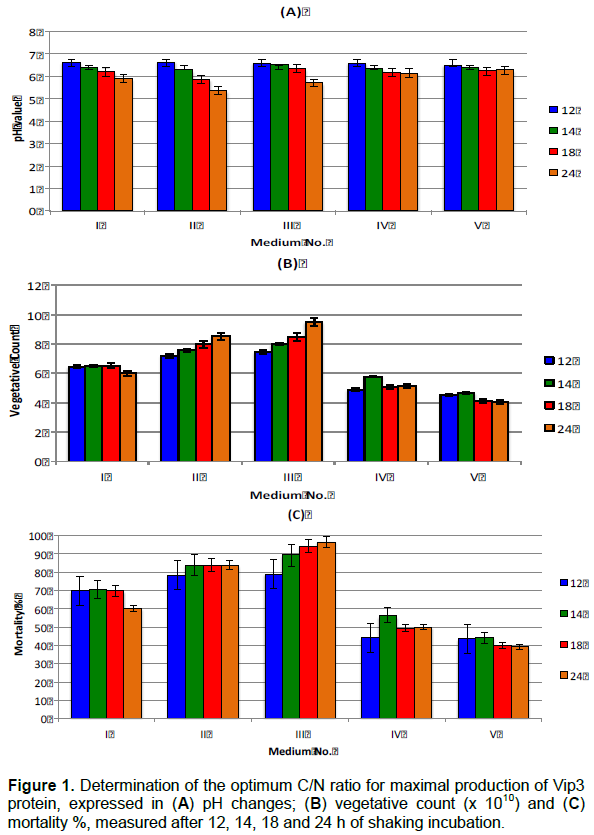
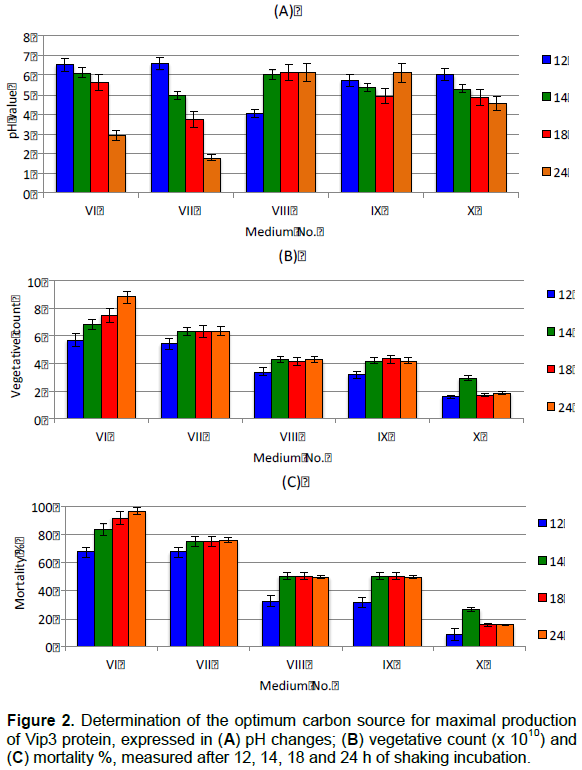
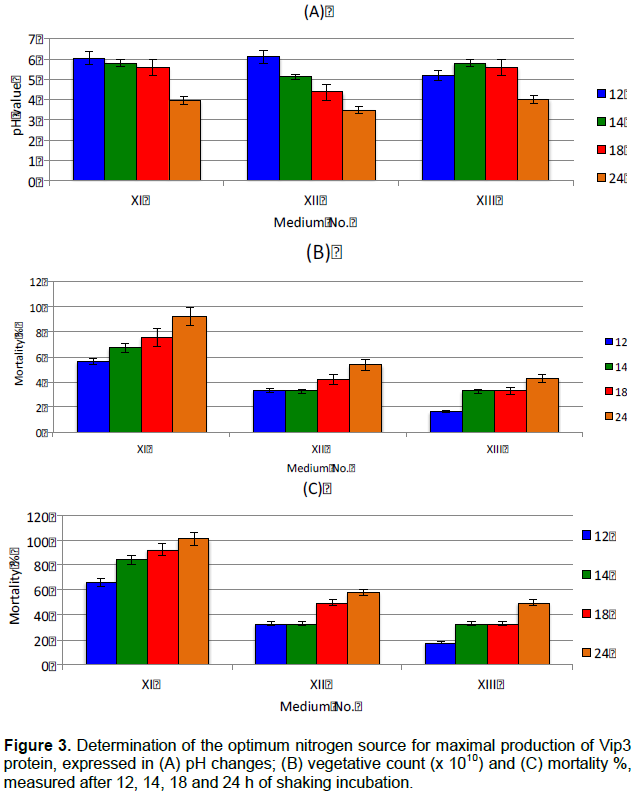
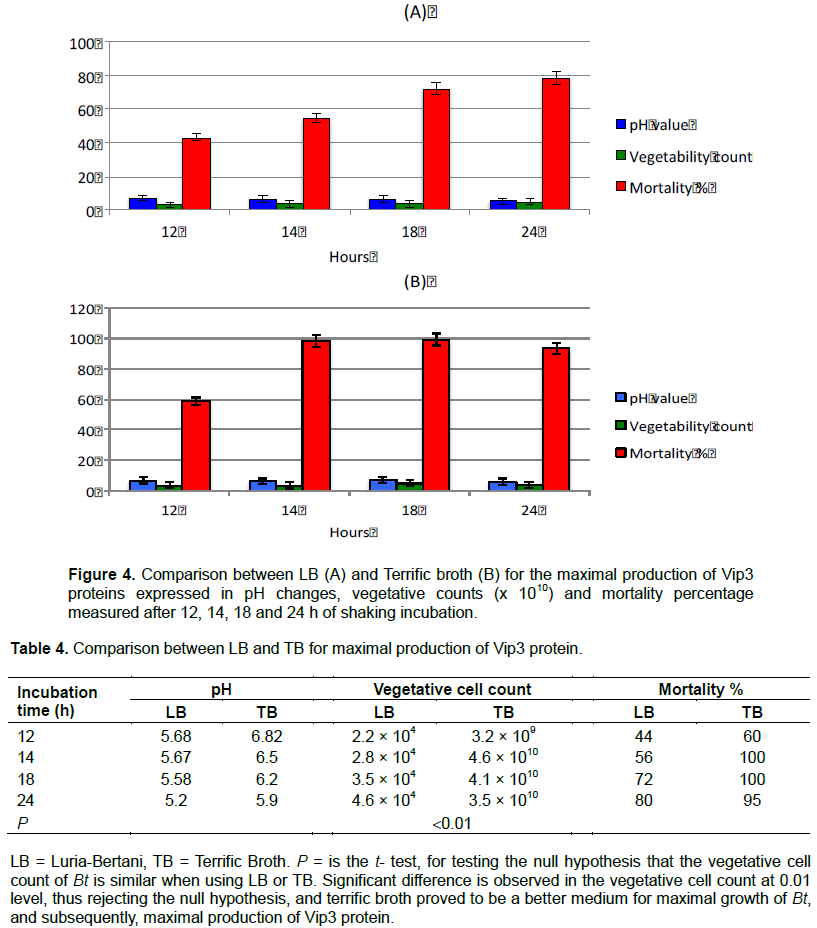
Effect of using terrific broth supplemented with different glucose concentrations on the growth of Vip3 toxin production by B. thuringiensis
The results showed that the vegetative cell count and mortality rate were increased and reached 8.0 ×1010 CFU and 100% mortality after 14 h in Terrific Broth supplemented with 20 g/l of glucose. It is interesting to note that when glucose was increased to more than 20 g/l in medium 4 and 5, the vegetative cell count decreased to 5.4×104 CFU with 50% mortality rate (Figure 5A, B and C).
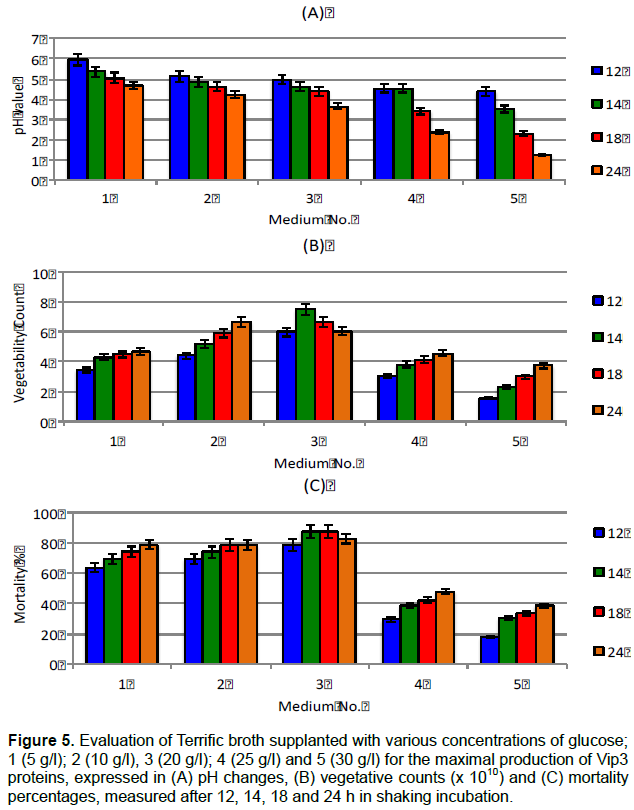
Comparison between the best formulated medium and common growth medium on the growth of Vip3 toxin production by B. thuringiensis
Obtained results showed that medium III (20 g/l glucose + 15 g/l soybean) was the best formulated medium; whereas medium 3 (Terrific Broth supplemented with 20 g/l glucose) was the best common growth medium. A comparison between the 2 media was important to select the optimum growth media for Vip3 production expressed by changes in pH (Figure 6A and Table 5), vegetative cell count, residual glucose, and mortality rate. The highest vegetative cell count of 8.4 ×1010 CFU in medium III was only reached after 24 h; however, medium 3 gave its highest vegetative cell count of 10 ×1010 after 22 h (Figure 6B and Table 5). Overall, significant differences (P < 0.01, t-test) were noted in terms of vegetative cell count between the 2 media, where medium 3 was superior in yielding 10-folds higher vegetative cell count after 12, 14, 16, 18 and 20 h (Table 5). Bacterial mortality rate of 100% was reached in medium III after 24 h, while in medium 3, the mortality rated reached 100% after 14 h (Figure 6C and Table 5). The residual glucose decreased from 20 to 12 g/l in medium III. However, medium 3, residual glucose reduced to 1.833 after 24 h (Figure 6D and Table 5). Therefore, Terrific Broth supplemented with 20 g/l glucose showed more superiority than the formulated medium III (20 g/l glucose + 15 g/l soybean) for the maximal production of Vip3 proteins.
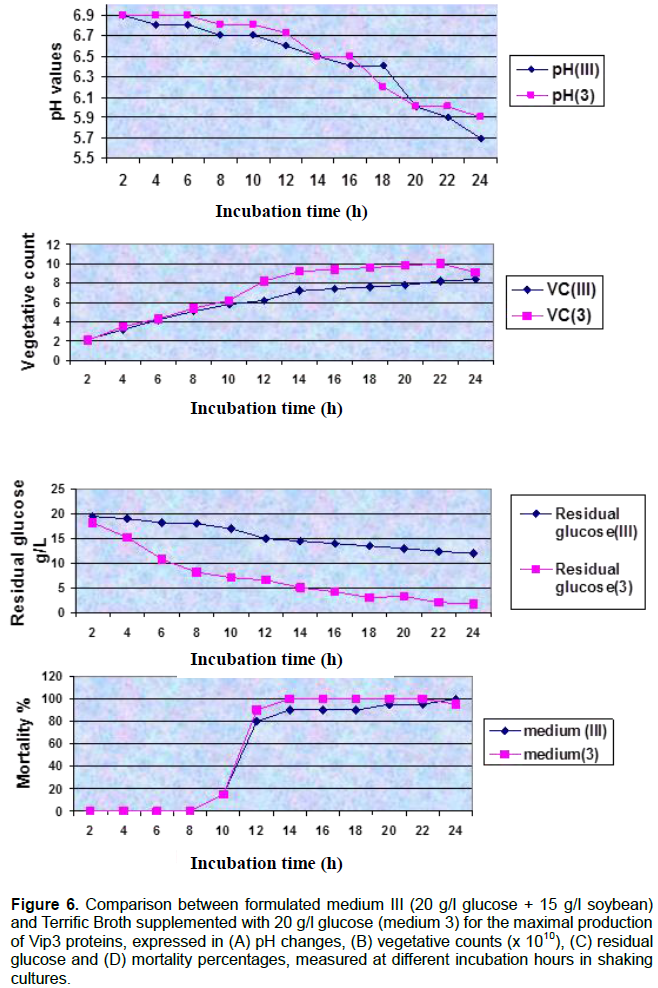
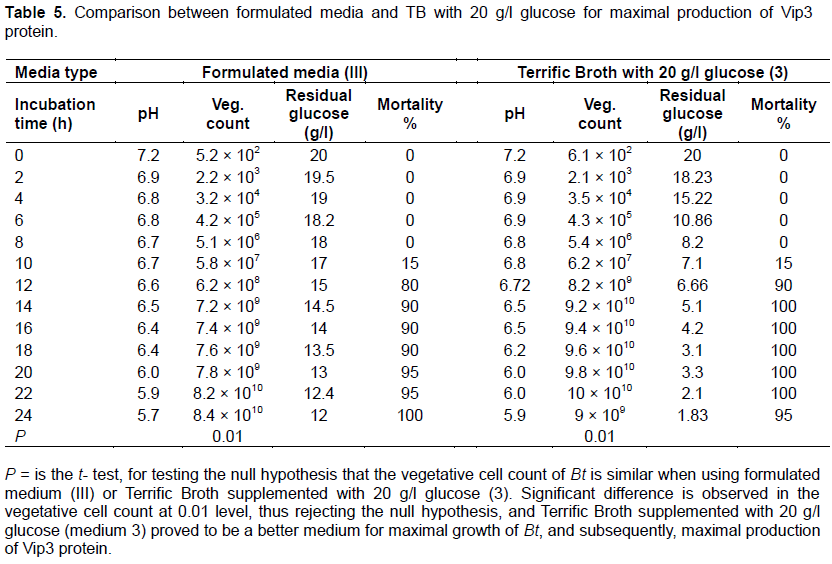
Recently the B. thuringiensis has been selected as the most successful commercial biopesticide used worldwide. It has been applied to protect crops and plants, and it is also expected to replace inorganic insecticides in several areas of application (Jouzani et al., 2017). Vip3A is a novel toxin with a broad spectrum of insecticidal activity and with a mode of action different from Cry1A toxins (Osman et al., 2015, 2018; Wang et al., 2018). Vip3 promises to be a new measure to kill insect pests.
Optimization of a cost-effective process of Vip3A production from B. thuringiensis necessitates the development of a fermentation method that allows the production of high amounts of Vip3a protein toxin. The aim of this study was to investigate the possibilities of supplying adequate amounts of glucose and other nutrients to maximize cell growth and Vip3 production using B. thuringiensis.
Previous studies (Dulmage, 1971; Gopinathan et al., 2016) showed that the C/N ratio was the key for B. thuringiensis vegetative toxins production. Thus, we optimized the C/N ratio to optimum level for maximal production of Vip3 proteins. The following ratios (1.45-4.75) were tested and the selection of the medium components was carried out based on the following considerations: (i) soybean meal was used as the nitrogen source as it was cheap and locally available; (ii) glucose was used as the carbon source as it is easily metabolized and easily available; (iii) potassium phosphate was used as the phosphate source; (iv) calcium carbonate was used as the buffering agent; (v) magnesium sulfate, manganese sulfate, ferrous sulfate, and calcium chloride were used as sources of trace elements. By increasing time, the vegetative cell count was increased in medium III (that is, from 7.4 × 1010 CFU after 12 h to 9.5 × 1010 CFU after 24 h); whereas, in medium II, the vegetative cell count increased from 7.2 × 1010 CFU after 12 h to 8.5× 1010 CFU after 24 h (Figure 1B). In medium I, IV, and V, the total cell count was constant during the growth period (Figure 1B), which means that the C/N ratios are not suitable for Vip3 production. These were also reflected in the results of mortality assay as these media (I, IV, and V) showed the lowest mortalities (Figure 1C). Based on the results obtained, we suggest that the C/N ratio of 3.88 was the best ratio for vegetative protein production. These results were also in agreement with the results reported by Dubois et al. (2019) who stated that the carbon content should be higher than the nitrogen content to obtain a higher vegetative count, delay sporulation, and crystal formation in B. thuringiensis cultures.
Different carbon sources, including complex sugars such as cornstarch and disaccharides (e.g. lactose and sucrose) were examined for optimum growth of B. thuringiensis. The highest vegetation cell count was obtained by glucose 7.8 × 1010 CFU with the highest mortality rate of 100%, while cornstarch showed the lowest vegetative count of 5.1 ×1010 CFU and a mortality rate of 50%. This may be due to the fact that glucose is rapidly metabolized and is known to repress the formation of proteolytic and Tri Carboxylic Acid (TCA) cycle enzymes in Bacillus (Doi, 1989), which supply precursor metabolites for the formation of sporulation-phase-associated protein (Berbert et al., 2008).
Different nitrogen sources were also examined including soybean, organic soluble source like urea, and inorganic source (ammonium sulfate). The soybean meal gave the highest vegetative count when used as the nitrogen source; similar observation was made by Sadek (2000) and Smitha et al. (2019). The vegetative cell count was increased in medium I (that is from 7.4 × 1010 CFU after 12 h to 9.5 × 1010 CFU after 24 h). On the other hand, the vegetative cell counts in medium number XI and XII (Table 3) were very poor with high mortalities (Figure 3A, B and C). Morris et al. (1997) stated that among the legumes, soybean meal showed the highest relative economic yield of biomass and relative potency against bertha armyworm larvae.
The experimental pH was within 5 to 6.6 and did not show any negative effect on the growth, which is in agreement with the results by Morris et al. (1997) who suggested that the pH within 5 to 6 did not produce any recoverable spore-crystal complex. Also, Bernhard (1993) concluded that Bt was not particularly sensitive to pH and growth will occur between pH 5.5 and 8.5 with optimum growth between pH 6.5 and 7.5.
Luria-Bertani (LB) and Terrific Broth (TB) media are nutrient-rich media primarily used for the growth of bacteria. They are composed of the following ingredients: (i)
peptides and
casein peptones; (ii)
vitamin (including B vitamin); (iii) trace elements (e.g., nitrogen, sulfur, magnesium); and (iv) minerals. Peptides and proteins are provided by
tryptone. Vitamin and certain trace elements are provided by yeast extract. Sodium ions responsible for transport and osmotic balance are provided by
sodium chloride. In addition, Terrific Broth contains glycerol, which is a cheap carbon source (Estruch et al., 1996).
A comparison between LB and TB media for the growth of B. thuringiensis was expressed by changes in pH, vegetative count, and mortality rates were included in this study. Obtained results showed that the pH of the two media was within the range and showed no bacterial mortality, this in agreement with the results of Bernhard (1993). However, the vegetative count reached its maximum only after 14 h in Terrific Broth with a 100% mortality rate. While in the LB, vegetative cell count reached the maximum after 24 h with 80% mortality rate. This is, probably, because the TB contained a higher amount of glucose than the LB medium, which helped TB to reach a high vegetative count within 14 h. Thus, B. thuringiensis growing in TB may produce Vip3 proteins faster than growing in LB medium.
Exploring the supplementation of TB with varied concentrations of glucose, showed that the vegetative cell count and mortality rates were increased and reached 8.0×1010 CFU and 100% mortality rate after 14 h in TB containing 20 g/l of glucose. It is interesting to note that when glucose was increased to more than 20 g/l in media 4 and 5 the vegetative cell count decreased to 5.4×104 CFU with 50% mortality rate. A similar result was also noted by Holmberg et al. (1980) who reported that a high concentration of nutrients inhibits the growth of B. thuringiensis var. thuringiensis by reducing sporulation and releasing of toxins. However, the opposite results were observed by Berbert-Molina et al. (2008) who stated that for the growth of B. thuringiensis var. kurstaki, a maximum glucose concentration (56 g/l) can be utilized. Most of the studies state that the substrate concentration should not exceed 23 g/l. The main problem of adding higher glucose concentrations in the medium, however, is the formation of acetate, which in un-buffered media causes a substantial drop in pH. Phosphate buffer helps control the growth rate by gradual glucose feeding rather than just putting in all the glucose at once.
As Terrific Broth supplemented with 20 g/l glucose (medium 3) showed a promising optimum growth of B. thuringiensis; and C/N ratio for Vip3 production was shown to achieve increased growth of the bacteria (medium III), thus, a comparison between the two media on the basis of pH, vegetative cell count, residual glucose and mortality rates was performed every 2 h for 24 h. In medium III, the vegetative cell count increased from 2.2 ×103 CFU (after 2 h) to 8.4 ×1010 CFU (after 24 h), while in medium 3 (terrific broth + 20 g/l glucose) vegetative cell counts were 2.1 ×103 CFU initially but reached 10 ×1010 CFU within 22 h. Bacterial mortality rate was (100%) in medium III after 14 h. An interesting thing to note was the residual glucose in both the medium after 24 h. Medium III consumed only 6.5 g of glucose, while medium 3 consumed 18.2 g of glucose. Although both the media (medium 3 and medium III) exhibited similar bacterial growth, medium 3 showed 100% bacterial mortality rate after 14 h and consumed a high amount of glucose. Therefore, medium 3 (TB with 20 g/l glucose) was better than the formulated medium III (20 g/l glucose and 15 g/l soybean) and thus we suggest to consider it as the low-cost medium of choice for maximal production of Vip3.
An optimized C/N ratio of 3.88 as glucose and soybean that served as carbon and nitrogen source, respectively, was shown to provide optimum growth conditions for increased growth of B. thuringiensis and subsequently higher yield of Vip3 protein production. However, Terrific Broth supplemented with 20 g/l glucose yielded optimum growth significantly (P <0.01, t-test) after 12, 14, 16, 18 and 20 h than the formulated medium with optimized C/N ratio of 3.8. Highest vegetative cell counts of B. thuringiensis in medium no. 3 was noted after 22 h; thus, indicating a higher production of Vip3 protein. The findings reported in this study should contribute to the low-cost production of Bt Vip3 proteins and encourage large scale production of the toxin. This is perhaps the first study to optimize the growth conditions of B. thuringiensis for the maximal production of Vip3A toxin.
The authors have not declared any conflict of interests.
REFERENCES
Abulreesh HH, Osman GEH, Assaeedi ASA (2012). Characterization of insecticidal genes of Bacillus thuringiensis strains isolated from arid environments. Indian Journal of Microbiology 52(3):500-503.
Crossref
|
|
Assaeedi ASA, Osman GEH, Abulreesh HH (2011). The occurrence and insecticidal activity of Bacillus thuringiensis in the arid environments. Australian Journal of Crop Science 5:1185-1190.
|
|
|
Bernhard K (1993). Development of Bacillus thuringiensis insecticides in Ciba-Geigy ad axemplified with CGA 237'218. In: The Biopesticide Bacillus thuringiensis and its Applications in Developing Countries. Salama HS, Morris ON, Rached E (Eds.), National Research Center, Cairo, Egypt., pp. 283-301.
|
|
|
Berbert-Molina MA, Prata AM, Pessanha LG, Silveira MM (2008). Kinetics of Bacillus thuringiensis var. israelensis growth on high glucose concentrations. Journal of Industrial Microbiology and Biotechnology 35(11):1397-1404.
Crossref
|
|
|
Burkness EC, Dively G, Patton T, Morey AC, Hutchinson WD (2010). Novel Vip3A Bacillus thuringiensis (Bt) maize approaches high-dose efficacy against Helicoverpa zea (Lepidoptera: Noctuidae) under field conditions: implications for resistance management. GM Crops 1(5):337-343.
Crossref
|
|
|
Doi RH (1989). Sporulation and germination. In: Bacillus. Harwood CR. (Ed.). Plenum, New York, USA, pp. 169-215.
Crossref
|
|
|
Dubois T, Tremblay YDN, Hamiot A, Martin-verstraete I, Deschapms J, Monot M, Briandet R, Dupuy B (2019). A microbiota-generated bile salts induces biofilm formation in Clostridium difficile. NPJ Biofilms and Microbiomes 5:14.
Crossref
|
|
|
Dulmage HT (1971). Production of-endotoxin by eighteen isolates of Bacillus thuringiensis, serotype 3, in 3 fermentation media. Journal of Invertebrate Pathology 18(3):353-358.
Crossref
|
|
|
El-Ghareeb DK, Osman GH, El Baz AF (2012). Isolation, cloning and overexpression of vip3Aa gene isolated from a local Bacillus thuringiensis. Biocontrol Science and Technology 22(1):11-21.
Crossref
|
|
|
Ernandes S, Del Bianchi VL, de Oliveria Moraes (2013). Evaluation of two different culture media for the development of biopesticides based on Bacillus thuringiensis and their application in larvae of Aedes aegypti. Acta Scientiarum-Technology 35:11-18.
Crossref
|
|
|
Estruch JJ, Warren GW, Mullins MA, Nye GJ, Craig JA, Koziel MG (1996). Vip3A, a novel Bacillus thuringiensis vegetative insecticidal protein with a wide spectrum of activities against lepidopteran insects. Proceedings of the National Academy of Sciences of the United States of America 93:5389-5394.
Crossref
|
|
|
Gopinathan C, Chaudhury A, Vivek AT (2016). Novel techniques for Bacillus thuringiensis subsp. israelensis. International Journal of Mosquito Research 3(4):17-29,
|
|
|
Hasanain AM (2017). Development of a cheap media for Bacillus thuringiensis growth. International Journal of Biotechnology and Bioengineering 3:221-229.
Crossref
|
|
|
Holmberg A, Sievanen R, Carlberg G (1980). Fermentation of Bacillus thuringiensis for exotoxin production: process analysis study. Biotechnology and Bioengineering 22(8):1707-1724.
Crossref
|
|
|
Jouzani GS, Valijanian E, Sharafi R (2017). Bacillus thuringiensis: a successful insecticide with new environmental features and tidings. Applied Microbiology and Biotechnology 101(7):2691-2711.
Crossref
|
|
|
Levinson ZH, Navon A (1969). Ascorbic acid and unsaturated fatty acids in the nutrition of the Egyptian cotton leaf worm. Journal of Insect Physiology 15(4):591-595.
Crossref
|
|
|
Marzban R (2012). Investigation on the suitable isolate and medium for production of Bacillus thuringiensis. Journal of Biopesticides 5(2):144-147.
|
|
|
Morris ON, Kanagaratnan P, Converse V (1997). Suitability of 30 agricultural products and by-products as nutrient sources for laboratory production of Bacillus thuringiensis subsp. aizawai (HD 133). Journal of Invertebrate Pathology 70(2):113-120.
Crossref
|
|
|
Nair K, Al-Thani R, Al-Thani D, Al-Yafei F, Ahmad T, Jaoua S (2018). Diversity of Bacillus thuringiensis strains from Qatar as shown by crystal morphology, δ-endotoxins and cry gene content. Frontiers in Microbiology 9:708.
Crossref
|
|
|
Navarro-Mtz AK, Martinez-Garcia R, Urazua-Valenzuela M, Roldan-Sabino C, Kakazey M, Juarez-Arellano EA (2018). High-energy ball millimg treatment of soybean for Bacillus thuringiensis culture media. Journal of Bioscience and Bioengineering 128(3):296-301.
Crossref
|
|
|
Osman G, Assaeedi ASA, Osman Y, El-Ghareeb D, Alreedy, R (2013). Purification and characterization of Bacillus thuringiensis vegetative insecticidal toxin protein(s). Letters in Applied Microbiology 57(4):310-6.
Crossref
|
|
|
Osman GEH, Alreedy R, Assaeedi ASA, Organji SR, El-Ghareeb D, Abulreesh HH, Althubiani AS (2015). Bioinsecticide Bacillus thuringiensis a comprehensive review. Egyptian Journal of Biological Pest Control 25:271-288.
|
|
|
Osman GEH, Altaf WJ, Saleh IAS, Soltane R, Abulreesh HH, Arif IA, Ramadan AM, Osman YA (2018). First report of detection of the putative receptor of Bacillus thuringiensis toxin Vip3Aa from black cutworm (Agrotis ipsilon). Saudi Journal of Biological Sciences 25(3):441-445.
Crossref
|
|
|
Osman GEH, Soltane R, Saleh, I. Abulreesh HH, Gazi KS, Arif IA, Ramadan AM, Alameldin HF, Osman YA, Idriss M (2019). Isolation, characterization, cloning and bioinformatics analysis of a novel receptor from black cutworm (Agrotis ipsilon) of Bacillus thuringiensis Vip3Aa toxins. Saudi Journal of Biological Sciences 26(5):1078-1083.
Crossref
|
|
|
Poopathi S, Kumar AK (2003). Novel fermentation media for production of Bacillus thuringiensis subsp. isrealensis. Journal of Economic Entomology 96(4):1039-1044.
Crossref
|
|
|
Poopathi S, Archana B (2012a). A novel cost-effective medium for the production of Bacillus thuringiensis subsp. israelensis for mosquito control. Tropical Biomedicine 29(1):81-91.
|
|
|
Poopathi S, Archana, B (2012b). Optimization of medium composition for the production of mosquitocidal toxins from Bacillus thuringiensis subsp. israelensis. Indian Journal of Experimental Biology 50(1):65-71.
|
|
|
Sadek KH (2000). Studies on some factors affecting growth and sporulation of Bacillus thuringiensis. M.Sc. thesis, Faculty of Science, Cairo University, Cairo, Egypt.
|
|
|
Siegwart M, Graillot B, Blachere Lopez C, Besse S, Bardin M, Nicot PC, Lopez-Ferber M (2015). Resistance to bio-insecticides or how to enhance their sustainability: a review. Frontiers in Plant Science 6:381.
Crossref
|
|
|
Smitha RB, Sailas B, Prakashkumar R (2019). Fermentation strategies for the production of a-amylase and δ-endotoxin from Bacillus thuringiensis subsp. kurstaki. Avid Science Monograph Series.2019.
Crossref
|
|
|
Valicente FH, de Souza Tuelher E, Leite MIS, Freire FL, Vieira CM (2010). Production of Bacillus thuringiensis biopesticide using commercial lab medium and agricultural by-products as nutrient sources. Revista Brasileira de Milho e Sorgo 9(1):1-11.
Crossref
|
|
|
Wang Z., Fang L, Zhou Z, Pacheco S, Gomez I, Song F, Soberon M, Zhang J, Bravo A (2018). Specific binding between Bacillus thuringiensis Cry9Aa and Vip3Aa toxins synergizes their toxicity against Asiatic rice borer (Chilo supperralis). Journal of Biological Chemistry 293(29):11447-11458.
Crossref
|
|
|
Yu X, Liu T, Sun Z, Guan P, Zhu J, Wang S, Li S, Deng Q, Wang L, Zhrng A, Li P (2012). Co-expression and synergism analysis of Vip3Aa29 and Cyt2Aa3 insecticidal proteins from Bacillus thuringiensis. Current Microbiology 64(4):326-331.
Crossref
|
|
|
Zou H, Ding S, Zhang W, Yao J, Jiang L, Liang J (2016). Study on influence factors in Bacillus thuringiensis production by semi-solid state fermentation using food waste. Procedia Environmental Sciences 31:127-135.
Crossref
|
|
|
Zribi Zghal R, Kharrat M, Rebai A, Ben Khedher S, Jallouli W, Elleuch J, Ginibre C, Chandre F, Tounsi S (2018). Optimization of bio-insecticide production by Tunisian Bacillus thuringiensis israelensis and its application in the field. Biological Control 124:46-52.
Crossref
|
|