ABSTRACT
Mass transfers pose significant challenge in fermentation due to wide diffusion gradient existing between the culture broth and the immobilized cells. In the present study, Saccharomyces cerevisiae LC 269108 was immobilized in a recently described microporous alginate beads. The new method was compared with the conventional calcium alginate gel beads in a repeated batch process for the production of ethanol. The fermentation conditions implemented were 110 rpm, pH 5.5 and temperature of 30°C for 60 h. The bead diameters were 3 mm while the calcium chloride concentration was 2.0%. In separate batch experiments conducted by the simultaneous saccharification and fermentation (SSF) method, the free cells maintained plateau at peak ethanol concentration of 7.50±0.33% after 36 h. In the conventional alginate (6.51±0.05%) and microporous beads (7.06±0.10%), ethanol dropped in concentration until reaching final volumes of 5.65±0.33 and 6.56±0.64%, respectively. In the repeated batch experiments, five fermentation batches or runs were conducted over a 12 h period each. The concentrations of ethanol produced in batches with the cells immobilized in calcium alginate were 2.91±0.34, 5.80±0.22, 5.01±0.39, 4.41±0.14 and 3.77±0.21%, respectively. Cells immobilized in microporous beads had higher ethanol output with concentrations 2.33±0.07, 6.62±0.04, 6.16±0.32, 5.90±0.2 and 4.70±0.26% obtained after five respective batches. Glucose metabolism was found to be lower with cells immobilized in alginate beads. From initial glucose concentration of 14.30±0.2%, residual glucose was detected after the first (3.61±2.11%), fourth (3.18±0.98%) and fifth (5.30±0.86%) batches of fermentation. In the batches containing microporous beads, residual glucose (5.36±0.29%) was confirmed after the first batch only. The present study demonstrates the feasibility of using microporous beads in the production of ethanol.
Key words: Mass transfer; microporous beads, immobilized cells, ethanol, calcium alginate.
The apprehension over the ecological issues generated by the wide scale appropriation of anthropogenic energy has propelled researches into the development of alternative energy resources that is cleaner, renewable and environmentally acceptable. Ethanol is an attractive option to fossil fuel because it is a renewable bio-based resource that is oxygenated and reduces emissions from compression ignition engines (Hansen et al., 2005). It is a liquid biofuel, which can be produced from different biomass feedstocks and conversion technologies (Balat et al., 2008). Apart from serving as important source of fuel for automobiles, the demand for ethanol has soared in recent times due to its extensive applications in medicine, industry and research.
Currently, advancements in fermentation biotechnology have led to the development of immobilized cell systems in bioreactors. Ethanol production using immobilized yeast cells offer several advantages over the free or suspended cell systems. It facilitates cell separation and reuse after fermentation, brings about reduction in adaptation phase and decreases inhibition caused by high concentrations of substrates and products (Lee et al., 2011; Devi and Nagamani, 2018). These attributes promote ethanol production and reduce operating costs (Duarte et al., 2013). Numerous materials such as calcium alginate, agar, polyurethane, polyvinyl alcohol etc., have been investigated as potential immobilization carriers (Rattanapan et al., 2011); but, calcium alginate beads are the most commonly used support because of good biocompatibility, low cost and ease of availability and preparation. Although gel degradation and low mechanical strength are some of the common issues associated with their use, mass transfer limitations remain by far, the most significant challenge encountered with the use of alginate beads as immobilization carriers (Bangrak et al., 2011).
Immobilization in alginate beads leads to the formation of nutrient and oxygen-deficient microenvironments at the core of the gel beads thereby restricting the cells to the periphery of the beads where they are more metabolically active to drive fermentation processes (Oyeagu et al., 2018). The described condition at the core of the beads leads to reduced cell growth rate and productivity of the entrapped cells. Researchers have been developing processes to deal with this problem for some time now. Reduction of the bead sizes (Ogbonna et al., 1991; Perego and Peratello, 1999) and adjusting packed bed bioreactors to higher flow rates (Hussain et al., 2015) are some of their reported approaches. The present study proposes the use of microporous beads as solution to mass transfer limitations in immobilized cells. The term ‘microporous’ is used to describe the occurrence of microscopic pores or micro-pores on the calcium-alginate gel beads formed by leaching out fillers into the curing solution following the induction of gel formation. The concept and methods of immobilization have been described in a previous paper (Oyeagu et al., 2018). The present study aims to demonstrate the usefulness of microporous beads in the production of bioethanol.
Saccharomyces cerevisiae LC 269108 was immobilized in the conventional alginate beads and compared to those immobilized in microporous beads in terms of ethanol production. The study was conducted in separate cell recycling repeated-batch fermentation mode operated by simultaneous saccharification and fermentation (SSF) using milled dried pulp of decomposing Dioscorea rotundata (yam) as feedstock.
The principal raw materials for the commercial production of ethanol are agricultural products, mainly corn and sugarcane (Dias et al., 2015; Eckert et al., 2018). However, many studies reporting the use of starchy tubers like cassava and sweet potato have been published (Lareo et al., 2013; Chutima et al., 2014). Spoilt yam (an agricultural waste) was chosen as feedstock in this study as one way of converting waste to wealth. Due to its high moisture content and short shelf life, yams deteriorate and spoil after a few months of harvest. In Nigeria, for example, nearly 40% of the annual harvested volumes are lost this way. With Nigeria’s placement as the world’s leading producer and exporter of yams (FAOSTAT, 2017), spoilt yams presently constitute major disposal, public health and environmental problems but the wastes could become useful raw materials in the production of bioethanol. This strategy would help make our environment cleaner, protect the neighborhoods from vector borne pathogens, reduce ethanol production costs, allay the fears of potential food security crises and create jobs. This report presents results of the first study on ethanol production from spoilt yams tubers by repeated-batch SSF with yeast immobilized in microporous alginate beads.
Yeast strain
The isolation and characterization of the yeast strain, S. cerevisiae LC 269108 have been reported (Nwuche et al., 2018). The organism was used as earlier described.
Preparation of the conventional (normal) alginate beads
For the production of the conventional alginate beads, a 2% (w/v) sodium alginate solution was prepared and sterilized by autoclaving at 121°C for 15 min. Then, a 2% (w/v) calcium chloride (CaCl2) was prepared in like manner in a separate beaker. Approximately, 1 ml of overnight culture of the yeast strain grown in YPD10% (1% yeast extract, 2% peptone and 10% glucose) was added to equivalent volume of the cooled sodium alginate preparation and shaken gently to mix. Each milliliter of the cell concentration corresponded to 4.0× 108 cells/ml. The mixture was then added drop wise into the beaker containing the calcium chloride solution gently stirred at 70 rpm (Magnetic Stirrer OP-912/3, Radelkis, Hungary) using a 5 ml automatic pipette. The alginate droplets solidified upon contact with the solution, forming alginate gel beads. Each bead contained a cell density of approximately 5.0 × 106 cells/bead. Stirring of the resulting beads was continued for 24 h to promote cross linking and stability of the beads (Ogbonna et al., 1989). The beads were recovered and washed in a sterile distilled water to remove excess calcium ion and un-entrapped cells before using in the fermentation experiments.
Preparation of the microporous beads
For the production of microporous alginate beads, 2% (w/v) sodium alginate was dissolved with 0.4% (w/v) soluble starch in 100 ml of distilled water and sterilized. After cooling, 1 ml of the resulting solution was added to 1ml of the standard cell suspension (corresponding to 4.0×108 cells/ml) and twirled gently to homogenize. A sterile 2% (w/v) calcium chloride (CaCl2) solution was prepared in a separate beaker as before. The cells was added to the solution of the soluble starch and sodium alginate and twirled slowly to mix. Using a 1 ml automatic pipette, the resulting mixture was added drop wise into the 2% CaCl2 solution gently stirred at 70 rpm by a magnetic stirrer (OP-912/3 Radelkis, Hungary).The alginate droplets hardened upon contact with the CaCl2 solution, forming gel beads. Each bead corresponded to a cell density of approximately 5.0 × 106 cells/bead. Stirring of the resulting beads was continued (curing) for 24 h to promote cross linking and stability of the beads (Ogbonna et al., 1989). Leaching of the soluble starch occurred at this time leading to development of microscopic pores on the beads (Oyeagu et al., 2018). As described earlier, the gel beads were recovered and washed in sterile distilled water to remove excess calcium and un-entrapped cells before using them in the fermentation experiments.
Sample collection and preparation of rotten yam pulp flour
The yam samples used in this study were handpicked in sterile sample packs from a spoilt farm produce dump and taken to the Crop Science Department, University of Nigeria, Nsukka for taxonomic identification. The tubers were peeled, cut, washed and dried at 80°C for 48 h. The dried yam chips were milled before taking through a horizontally rotating sieve SKH-01 (Fujiwara Scientific Company) having a pore size of 90 µm. The harvested light brown flour was maintained at -30°C until required for use.
Batch SSF of pretreated yam flour and culture conditions
The feedstock (yam flour) was initially subjected to thermal treatment in boiling water for 10 mins to enhance gelatinization/ liquefaction. When cooled to 40°C, enzymatic hydrolysis was initiated with a cocktail composed of amyloglucosidase (46.65 μl), cellulase (0.55 μl) and pectinase (4.21 μl) in order to reduce the viscosity of the paste after the liquefaction regimen. Each batch of SSF experiment comprised of 20% (w/w) pre-treated yam flour mixture in a 50 ml screw capped falcon tube. 0.5 ml of nitrogen base (1% yeast extract and 2% peptone) was added before the medium was aseptically inoculated with the immobilized (calcium alginate or microporous beads) or standardized 1 ml overnight culture of the yeast strain. The concentration of the inoculum was 4.0×108 cells/ml. The set-up was incubated at 30°C for 12 h. Approximately, 100 µl samples were withdrawn two hourly from the sealed fermentation tubes, transferred into 1.5 ml Eppendorf tubes and preserved at -30°C until analyzed for ethanol and glucose.
Repeated-batch SSF of pre-treated yam flour
In the repeated-batch SSF, after the 12 h batch fermentation described in the previous section, the immobilized cells were retrieved by filtration through a sterile mesh sieve (2 mm). The collected cells were then reused for the next batch of fermentation, and the process was repeated five times according to the method described by Watanabe et al. (2012).
Analytical methods
The proximate composition of the yam flour used was determined according to Standard Methods (2005). The maximum theoretical ethanol yield from sugar was calculated from the stoichiometric relation according to Siqueira et al. (2008): 100 g of hexose produces 51.1 g of ethanol and 48.9 g of CO2. The theoretical ethanol yield was calculated from the following equation:
Theoretical ethanol yield (%) = Ethanol Conc. (%) / Glucose Conc. (%) × 100
Glucose was monitored using High Performance Liquid Chromatography system (TOSOH, Tokyo, Japan) while ethanol was determined by a gas chromatography (Model GC-2014; Shimadzu Seisakusho, Kyoto, Japan). The operating conditions of the equipment were reported in Nwuche et al. (2018).
Statistical analysis
All reported experiments were conducted in triplicates. The standard error of mean was calculated and the mean values plotted at 95% confidence limit against the days of fermentation. Ethanol production in batch experiments was compared using one way analysis of variance (ANOVA).
Batch SSF of 20% (w/v) flour from dried yam pulp
SSF is a commonly employed microbial technique for the production of bioethanol. It is a one-step fermentation method that involves conversion of glucose to ethanol in the same vessel with reduction in fermentation time, decrease in capital cost and increase in production of ethanol (Sarkar et al., 2012; Dey et al., 2015) as some of its attractive advantages. Many fermentation systems operate a repeated fed-batch operation with SSF in a cell-recycling mode. To this extent, the use of flocculating and immobilized cells have been reported in the fermentations of cassava starch (Amuthaa and Gunasekaran, 2001; Choi et al., 2009) and lignocellulosic materials such as rice straw (Watanabe et al., 2012). Ethanol production by the free and immobilized (alginate and microporous) cells was conducted by separate batch SSF ahead of the repeated batch experiments. In Figure 1, the kinetics of ethanol concentration by the three (free cells, microporous immobilized and normal alginate immobilized cells) different cells is presented. Ethanol concentration in the batch inoculated with free cells reached 7.12±0.13% (87.2% of the theoretical yield) after 12 h and gradually increased further to 7.50±0.33% (90.73% of the theoretical yield) after 36 h before maintaining plateau for the rest duration of the experiment. In contrast, ethanol produced from the cells immobilized in alginate and microporous beads reached peak after 36 h and thereafter dropped in concentration. In the culture inoculated with cells immobilized in normal alginate, for instance, the ethanol concentration was 6.37±0.08% (76.7% of theoretical yield) after 12 h. This concentration increased to 6.51±0.05% (78.4% of theoretical yield) after 36 h before dropping to 5.65±0.33% (68% of theoretical yield) at the end of experiment. The batch containing cells immobilized in microporous beads had higher ethanol output than the batches, which had the conventional alginate beads. After 12 h, the concentration of ethanol produced was 6.49±0.49% (78.7% of theoretical yield) before it increased to 7.06±0.10% (85.6% of theoretical yield) after 36 h. The final ethanol concentration after 60 h was 6.56±0.64% (79.6% of the theoretical yield).
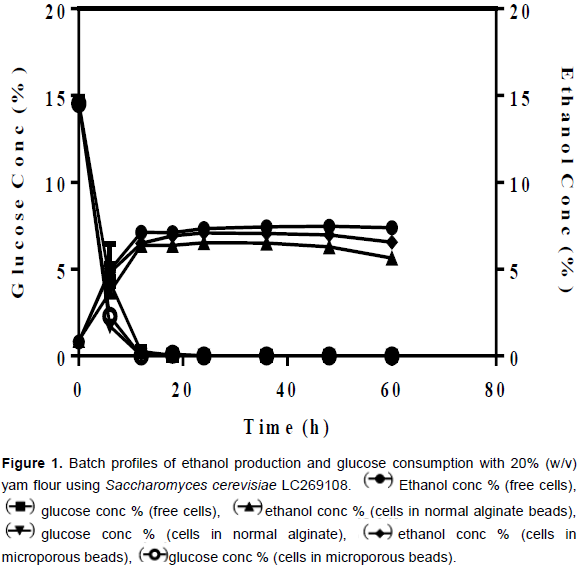
Many studies have indicated the numerous advantages of immobilized cultures in the production of ethanol. Besides the benefits of easier separation from the reaction mixture, possibility of cell reuse and higher substrate conversion, ethanol production have also been reported to be higher than when free cells were used (Puligundla et al., 2011; Yao et al., 2011). However, results obtained in the present study indicated otherwise. The concentration of ethanol produced by the free cells was higher compared to those produced by the immobilized cells. This position is shared by Duarte et al. (2013). Such difference is explainable by mass transfer limitations occurring within the immobilization matrix. Alginate beads are known to maintain two distinct spheres or regions, the core of the beads, which is characteristically anaerobic and farthest way from the culture broth and the periphery where the cells are most metabolically active due to increased access to medium materials (Oyeagu et al., 2018). During fermentation, the cells located at the core are relatively inactive and thus contributes insignificantly to fermentation due to limitations imposed by the absence of the medium resources. The microporous beads were found to be beneficial to higher ethanol production than the normal alginate beads because the micropores formed as result of the leaching of starch during preparation facilitated movement of nutrients even to the core of the beads.
This ensures net flow of materials because nutrients and oxygen are delivered to all parts of the beads while metabolic wastes are channeled back to the culture medium. The microporous beads thus tend to hold much higher concentration of metabolically active cells than those immobilized in conventional alginate beads. This explains the higher concentration of ethanol produced by the former.
Repeated batch SSF of the 20% (w/v) flour from dried yam pulp
To evaluate the adaptation of the microporous beads to prolonged fermentation, a cell recycling repeated batch SSF was carried out and compared to the alginate beads. Five repeated batches were run at 12 h intervals. The time interval was chosen because glucose was found to completely exit the system within 12 h of fermentation. Samples were withdrawn for analysis in 2 h. The kinetics of ethanol and glucose fermentation is presented in Figures 2 and 3. In the batch SSF experiment with cells immobilized in alginate beads, ethanol production reached peak of 2.91±0.34% (38.9% of the theoretical yield) in the first batch of experiment. In the second batch (B 2), ethanol increased to 5.80±0.22% (73.9% of the theoretical yield) before dropping to 5.01±0.39% (60.3% of the theoretical yield) and 4.41±0.14% (52.6% of the theoretical yield) in the third and fourth batches respectively. The concentration decreased further to 3.77±0.21% (45.4% of the theoretical yield) in the final batch of experiment. Time courses of experiment equally revealed that ethanol concentration at zero hour followed an increasing order from the first batch of experiment to the last. In the first batch, the concentration of 0.092% was observed. Subsequently, higher values were obtained in the second (0.4526%) and other batches. Values obtained before the third (0.914%) and fourth (0.983%) batches commenced were comparable. The fifth batch profile showed 1.128% ethanol concentration prior to fermentation.
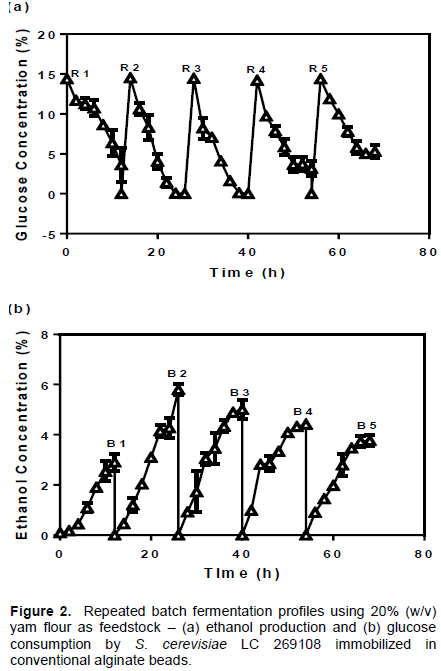
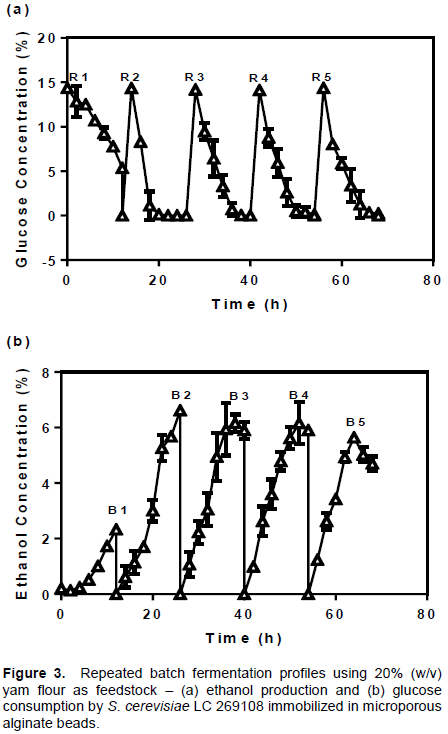
In the batches of experiment with cells immobilized in microporous beads (Figure 3b), maximum ethanol production after the first batch was 2.33±0.07% (30.6% of the theoretical yield). This value increased significantly to 6.62±0.04% (82.5% of the theoretical yield) in Batch 2 (B 2) before dropping to 6.16±0.32% (B 3) and 5.90±0.02% (B 4) respectively. The calculated theoretical yields were 72.7 and 69.7% respectively. Ethanol production in the fifth batch was lowest (4.70±0.26%) with 55.27% theoretical yield. As earlier indicated, ethanol concentration at zero hour increased progressively in concentration across the different batches of experiment. For instance, from an initial 0.213% in the first batch, the concentration increased to 0.620% (B 2), before the values for the third (1.07%) and fourth (0.99%) batches were determined. The concentration of ethanol before fermentation of the final batch was 1.23%. Such increments in the zero hour concentration of ethanol across the batches might have come from two possible sources. The first is the broth remnants adhering to the immobilizing beads from the previous fermentation run and second is the absence or reduction in adaptation phases during the successive batches of experiment (Duarte et al., 2013). From the present study, ethanol decreased subsequently after reaching peak in the second batch. This observation was common to both alginate and microporous beads (Figure 2b and 3b) and may have arisen from loss of cells due to breakage or leakages from the beads (Bangrak et al., 2011). One of the limitations of the alginate beads is their low mechanical strength (Watanabe et al., 2012). It is thought that abrasions resulting from agitations during fermentation weakens and ruptures the beads. In terms of ethanol output, the cells immobilized in microporous beads were significantly better (p<0.05) than the performance of those immobilized in alginate beads particularly from the second to the fifth batches of fermentation. Apart from improvement in mass movement of medium materials, the microporous beads were physically more stable than the normal beads. The first reason is due to increased penetration of CaCl2 to the core of the beads. This results in the formation of more cross-linkages between carboxyl groups in the alginate with the calcium ions from the chloride solution. Consequently, calcium progressively accumulates in the beads bringing about a hardening effect. Secondly, earlier report (Oyeagu et al., 2018) showed that incorporating 0.4% starch during preparation of the microporous beads adds significantly to increased stability and cell holding capacity of the resulting beads than any other concentration or fillers tested. Watanabe et al., (2012) and Duarte et al. (2013) have equally reported a variety of techniques aimed at strengthening and fortifying alginate beads with the aim of achieving longer term applications during fermentation.
Glucose metabolism was observed to be lower with cells immobilized in normal alginate beads (Figure 2a). At the end of the first batch of experiments, the residual concentration of glucose was found to be 3.61±2.11%. This remnant might account for the adaptive or lag phase response, which normally occurs when microbial cells are acclimatizing to the physical conditions of a fermentation system. In the second and third batches, glucose was not detected in the broth. Residual glucose emerged again in the fourth (3.18±0.98%) and fifth (5.30±0.86%) batches respectively. Cell loss could also in part account for the residue glucose but it is known that the activity of cells immobilized in conventional alginate is restricted to the peripheral zones where limited concentration of cells is located. In the microporous beads, glucose consumption was faster (Figure 3a). After the unconsumed concentration of 6.36±1.12% was found at the completion of the first batch, glucose was not detected again in the other batch profiles of experiment. This confirms further that a higher and more robust consortium of metabolically active cells resided in the microporous beads than in the normal alginate.
In the present study, microporous beads was investigated as potential solution to mass transfer challenges using ethanol production as case study. The results showed improvement in mass movement as indicated by the higher product concentrations achieved using the microporous beads. However, additional studies are needed to optimize the process in order to determine the set of conditions most beneficial to the ethanol fermentation. Many factors such as incubation temperature, initial pH and sugar concentration are known to influence growth and ethanol production in yeasts. Although the addition of 0.4% starch improved stability of the resulting beads, further research is obligatory in order to develop newer options for improving the stability and strength of the beads to enable them become more adaptable to longer fermentations periods. Also, contamination of culture did not occur despite using the feedstock (prepared from putrefying yam tuber) without sterilization. The reasons for this might be due to rapid consumption of glucose by the yeast, the high concentration of ethanol produced and the adaptation of the yeast strain (S. cerevisiae LC 269108) to the high temperature of fermentation. The significance of this to savings in energy and overall cost of production is very critical for large scale production of ethanol.
The authors have not declared any conflict of interests.
This work was supported by funding from the ministry of Agriculture, Forestry and Fisheries of Japan (Development of climate change mitigation technology through effective utilization of agro-processing residues). The authors wish to appreciate the Japan International Research Centre for Agricultural Science (JIRCAS) for funding the work.
REFERENCES
Amuthaa R, Gunasekaran P (2001). Production of ethanol from liquefied cassava starch using co-immobilized cells of Zymomonasmobilis and Saccharomyces diastaticus. Journal of Bioscience and Bioengineering 92(6):560-564.
Crossref
|
|
Balat M, Balat H, Oz C (2008). Progress in Bioethanol Processing. Progress in Energy and Combustion Science 34(5): 551-573.
Crossref
|
|
|
Bangrak P, Limtong S, Phisalaphong M (2011). Continuous ethanol production using immobilized yeast cells entrapped in loofa-reinforced alginate carriers. Brazilian Journal of Microbiology 42(2):676-684.
Crossref
|
|
|
Choi GW, Kang HW, Moon SK (2009). Repeated-batch fermentation using flocculent hybrid, Saccharomyces cerevisiae CHFY0321 for efficient production of bioethanol. Applied Microbiology and Biotechnology 84(2):261-269.
Crossref
|
|
|
Chutima K, Dethoup T, Limtong S (2014). Ethanol production from cassava using a newly isolated thermotolerant yeast strain. Science Asia 40:268-277.
Crossref
|
|
|
Devi NKD, Nagamani ASS (2018). Immobilization and estimation of activity of yeast cells by entrapment technique using different matrices. International Journal of Pharmaceutical Sciences and Research 9(7):3094-3099.
|
|
|
Dey P, Lhakpa W, Singh J (2015). Simultaneous saccharification and fermentation (SSF), an efficient process for bio-ethanol production: an overview. Biosciences Biotechnology Research Asia 12(1):87-100.
Crossref
|
|
|
Dias MOS, Filho RM, Mantelatto PE, Cavalett O, Rossell CEV, Bonomi A, Leal MRLV (2015). Sugar cane processing for ethanol and sugar in Brazil. Environmental Development 15:35-51.
Crossref
|
|
|
Duarte JC, Rodrigues JAR, Moran PJS, Valenca GP, Nunhez JR (2013). Effect of immobilized cells in calcium alginate beads in alcoholic fermentation. AMB Express 3(1):31.
Crossref
|
|
|
Eckert CT, Frigo EP, Albrecht LP, Albrecht AJP, Christ D, Santos WG, Berkembrock E, Egewarth VA (2018). Maize ethanol production in Brazil: characteristics and perspectives. Renewable and Sustainable Energy Reviews 82(3):3907-3912.
Crossref
|
|
|
FAOSTAT (2017). View (accessed//.05.17).
|
|
|
Hansen AC, Zhang Q, Lyne PWL (2005). Ethanol diesel fuel blends - a review. Bioresource Technology 96(3):277-285.
Crossref
|
|
|
Hussain A, Kangwa M, Abo-Elwafa AG, Fernandez-Lahore M (2015). Influence of operational parameters on the fluid-side mass transfer resistance observed in a packed bed bioreactor. AMB Express 5:25.
Crossref
|
|
|
Lareo M, Ferrari MD, Guigou M, Fajardo L, Larnaudie V, Ramirez MB, Garreiro JM (2013). Evaluation of sweet potato for fuel bioethanol production: hydrolysis and fermentation. Springer plus 2:493.
Crossref
|
|
|
Lee KH, Choi IS, Kim YG, Yang DJ, Bae HJ (2011). Enhanced production of bioethanol and ultrastructure characteristics of reused Saccharomyces cerevisiae immobilized calcium alginate beads. Bioresource Technology 102(17):8191-8198.
Crossref
|
|
|
Nwuche CO, Murata Y, Nweze JE, Ndubuisi IA, Omae H, Saito U, Ogbonna JC (2018). Bioethanol production under multiple stress condition by a new acid and temperature tolerant Saccharomyces cerevisiae strain LC 269108 isolated from rotten fruits. Process Biochemistry 67:105-112.
Crossref
|
|
|
Ogbonna JC, Amano Y, Kazuo N (1989). Elucidation of optimum conditions for immobilization of viable cells by using calcium alginate. Journal of Fermentation and Bioengineering 67(2):92-96.
Crossref
|
|
|
Ogbonna JC, Matsumura M, Kataeka H (1991). Effective oxygenation of immobilized cells through reduction in bead diameter. Process Biochemistry 26(2):109-121.
Crossref
|
|
|
Oyeagu U, Nwuche CO, Ogbonna CN, Ogbonna JC (2018). Addition of fillers to sodium alginate solution improves stability and immobilization capacity of the resulting calcium alginate beads. Iranian Journal of Biotechnology 16(1):67-73.
Crossref
|
|
|
Perego C, Peratello S (1999). Experimental methods in catalytic kinetics. Catalysis Today 52(2-3):133-145.
Crossref
|
|
|
Puligundla P, Poludasu RM, Rai JK, Obulan VSR (2011). Repeated batch ethanolic fermentation of very high gravity medium by immobilized Saccharomyces cerevisiae. Annals of Microbiology 61(4):863-869.
Crossref
|
|
|
Rattanapan A, Limtong S, Phisalaphong M (2011). Ethanol production by repeated batch and continuous fermentations of blackstrap molasses using immobilized yeast cells on the shell silk cocoons. Applied Energy 88(12):4400-4404.
Crossref
|
|
|
Sarkar N, Ghosh SK, Bannerjee S, Aikat K (2012). Bioethanol production from agricultural wastes: an overview. Renewable Energy 37(1):19-27.
Crossref
|
|
|
Siqueira PF, Krap SG, Carvalho JC, Sturm W, Rodrigues - Leon JA, Tholozan JL, Singhania RR, Pandey A, Soccol CR (2008). Production of bioethanol from soybean molasses by Saccharomyces cerevisiae at laboratory, pilot and industrial scales. Bioresource Technology 99(17):8156-8163.
Crossref
|
|
|
Standard Methods for the Examination of Water and Waste Water (2005). 21st edition, American Public Health Association (APHA), American Water Works Association (AWWA) and Water Environment Federation (WEF), Washington, D.C., USA.
|
|
|
Watanabe I, Miyata N, Ando A, Shiroma R, Tokuyasu K, Nakamura T (2012). Ethanol production by repeated batch simultaneous saccharification and fermentation (SSF) of alkali-treated rice straw using immobilized Saccharomyces cerevisiae cells. Bioresource Technology 123:695-698.
Crossref
|
|
|
Yao W, Wu X, Zhu J, Sun B, Zhang YY, Miller C (2011). Bacterial cellulose membrane - a new support carrier for yeast immobilization for ethanol fermentation. Process Biochemistry 46(10):2054-2058.
Crossref
|
|