ABSTRACT
The extraction of high-quality DNA from okra (Abelmoschus esculentus L. Moench) is notoriously troublesome due to the high contents of polysaccharides, polyphenols, and different secondary metabolites. We have tested seven extraction buffers on silica dried okra leaves. Here, we describe a simple, rapid and modified procedure for high-quality DNA extraction from okra, which is amenable for downstream analyses. In contrast to Cetyl-trimethyl-ammonium bromide (CTAB) methods, the described procedure is rapid, omits the use of liquid nitrogen, phenol, PVP-10, and chloroform. It also uses inexpensive and less hazardous reagents and requires only ordinary laboratory equipment. The procedure employed a high concentration of Sodium dodecyl sulphate (SDS) to rid the problems associated with polysaccharides and polyphenols. The average yield was between 36 and 45 μg of total DNA from 90 mg of dried leaf weight. The DNA is adequate for molecular analysis of okra, such as genetic mapping or marker-assisted plant breeding. This protocol can be performed in as little as 3 h and may be adapted to high-throughput DNA isolation.
Key words: PVP-10, polyvinylpyrrolidone, non cetyltrimethylammonium bromide, okra, genomic DNA, Sodium dodecyl sulphate (SDS).
Okra (Abelmoschus esculentus L. Moench), belonging to the Malvaceae family, is an edible vegetable species that is widely cultivated and distributed in the tropical, subtropical and warm temperate areas of the world (Kumar et al., 2013; Lamont, 1999; Diizyaman, 1997; Martin, 1982). The crop is believed to be originated in Ethiopia (Harlan, 1969). It is usually cultivated for its edible immature fruit in fresh or dried form. Nevertheless, the plant is also used as a source of protein (Gemede et al., 2015), fiber (Khan et al., 2017), biomass (Lee et al., 2018), oil (Wei et al., 2017), mucilage (Fronda et al., 2018), colorants (Waghela and Khan, 2018), traditional medicine (Dubey and Mishra, 2017), pharmaceuticals (Zhang et al., 2018) and as ornamental crop (Diizyaman, 1997).
Okra is a highly diversified crop (Akotkar et al., 2010). The wide economic importance of the plant is urging its improvement for different purposes. Thus, breeding elite varieties and developing different cultivars is needed. Even though okra has a wide range of importance and its center of origin and diversity is in Ethiopia (Harlan, 1969), molecular variability studies and cultivar improvement programs are still at their infancy.
For studying the molecular characteristics and genetics of okra, extraction of high-quality DNA in sufficient quantity is important. However, extraction of high quality and quantity DNA is often a limiting factor in okra (Porebski et al., 1997), since a large number of species from the Malvaceae family contain secondary metabolites like mucilage (Singh and Kumar, 2012) including alkaloids, phenolic compounds, gummy polysaccharides, terpenes and quinine (Ali et al., 2019; Amani et al., 2011; Porebski et al., 1997; Singh and Kumar, 2012).
During cell lysis, nucleic acids come into contact with these polysaccharides in the oxidized form. The polyphenols bind covalently and irreversibly to proteins and nucleic acids resulting in a brown gelatinous material (Agrawal et al., 2016). This reduces the yield and purity of DNA. Thus, the quality and quantity of DNA will interfere with the subsequent reactions such as PCR, gene cloning and restricted DNA digestion, and sequencing.
Several protocols have been developed for genomic DNA extraction for okra (Jeyaseelan et al., 2019; Seth et al., 2018; Singh and Kumar, 2012). However, these protocols involve the use of hazardous and technologically demanding chemicals like phenol, chloroform, and liquid nitrogen. These chemicals are not recommended in open laboratories with no specialized rooms and safety cabinets. For these reasons, an effective and appropriate protocol is needed for isolating genomic DNA for genotyping, PCR work and sequencing of okra with inexpensive resources while avoiding the health implications of phenol and chloroform (Mahuku, 2004).
The objective of this study was to develop a straightforward technique to isolate deoxyribonucleic acid, a way that eliminates the necessity to use phenol or chloroform to purify the DNA. The resulting SDS (Sodium dodecyl sulphate) protocol was used to isolate high quality genomic DNA subject to PCR analysis from different accession of okra with reduced cost and health concerns. The results will be used for further molecular studies. It also supports technology inaccessible countries to exclude the high cost and impact of phenol-chloroform, liquid nitrogen and enzymes like RNase.
The DNA extraction process was carried out at the Plant Cell Laboratory, School of Plant and Horticultural Sciences, Hawassa University. Young and healthy leaves from forty-four okra accessions grown in the field were collected from the agricultural field of Hawassa University. The leaves were divided into two batches, one batch was dried with silica gel and the fresh samples were kept in plant collection bag for temporary use.
Solutions
Seven genomic DNA extraction buffers (KCL, CTAB, and SDS) were used with and without modifications in the buffer composition (Table 1).
Protocol for SDS based DNA extraction
1. Ninety (90) mg of silica dried okra leaf tissue were ground to a fine powder with the help of three metal tungsten carbide beads over the vortex mixer supported by micropipette tip based crushing of a sample in an Eppendorf tube.
2. Eight hundred (800) µl of cell lysis buffer (0.5% SDS (w/v) in 10XTE) was added to each tube followed by vortexing at high speed for approximately 2 min until the powder was fully hydrated and mixed with buffer.
3. Samples were incubated for 10 min at room temperature (RT).
4. This step was followed by precipitation of genomic DNA with 200 μl 3 M sodium acetate (pH 5.2) and mixed by inversion of tubes.
5. The mixture was incubated on ice for 5 min.
6. Samples were centrifuged at 16,000Xg for 5 min at RT to pellet the leaf material.
7. The liquid material was transferred carefully to an empty 1.5 ml centrifuge tube.
8. An equal volume of isopropanol was added to the supernatant and completely suspended by vortexing and inverting the tubes (approximately 20 s).
9. Samples were incubated for 15 min at RT by inverting tubes every three minutes by hand.
10. The samples were centrifuged at 16,000Xg for 3 min at RT followed by removal of supernatant with a pipette.
11. 500 µl of freshly prepared wash buffer (5 M NaCl and 95% ethanol) was added to each tube and completely suspended by vortexing the tubes (approximately 20 s).
12. The step was followed by centrifuging the sample at 16,000Xg for 3 min at RT to pellet the genomic DNA.
13. The last step was the removal of the supernatant and washing the pellet with 75% cold ethanol (4°C).
14. The pellet was allowed to dry at room temperature before elution with 60 µl of 1X TE buffer.
15. The DNA was stored temporarily at 4°C before checking its quality and quantity.
Protocol for KCl based DNA extraction
1. Ninety (90) mg of silica dried okra leaf tissue was weighted.
2. Sample was placed into a 1.5 ml tube.
3. 400 ul of DNA extraction buffer (1 M KCl, 100 mM Tris-HCl, 10 mM EDTA) was added to the tube.
4. Sample was crushed by the tip of the pipette inside the Eppendorf tube.
5. Sample was incubated for 30 min (~1 h) at 65°C in a water bath and consequently centrifuged for 10 min at 15000 rpm at 25°C.
6. 100 µl of isopropanol was added to a new 1.5 ml tube or 96-well plate while waiting.
7. 100 µl of supernatant was transferred into the tube prepared in step 6.
8. Samples were mixed by pipetting or inverting tube.
9. The mixture was centrifuged at maximum speed (>2800 rpm) for 30 min at 4°C.
10. The supernatant was discarded by inverting the tube.
11. 150 µl of 70% ethanol was added to wash the pellet.
12. The mixture was centrifuged at maximum speed (>2800 rpm) for 15 min at 4°C.
13. The supernatant was discarded by inverting the tube.
14. The tube was placed upside down for about 10 (~30) min to dry.
15. 30 μl of 1xTE were added to elute the DNA.
16. Samples were kept at 4°C for at least one day to elute well.
Protocol for CTAB based DNA extraction
Protocol for CTAB was followed according to Devi et al. (2013).
Gel electrophoresis
2 μl of eluted DNA were subjected to electrophoresis on a 0.8 mg/ml ethidium bromide stained 1% (w/v) agarose (Thermo Scientific, USA) gel in 1XTris-EDTA (TE) buffer. The agarose gel was documented on a GelDoc (BIO-RAD, USA).
Qualitative and quantitative analyses of the isolated DNA
The yield and purity of extracted DNA was assessed using Nano-Drop 2000 (Thermo-Scientific, USA), by measuring the concentration and UV absorption ratios at A260/280 and A260/230. Agarose gel electrophoresis was performed to determine the level of DNA degradation and contamination by RNA.
PCR amplification confirmation
PCR was carried out in a thermal cycler (BIO-RAD, USA) to amplify the specific DNA sequence, in a reaction volume of 10 µl containing PCR buffer (10 mMTris-HCl, 50 mMKCl), 50 ng of the DNA, 1.5 mM MgCl2, 0.5 units of Taq polymerase (Fermentas, USA), 0.1 mM of dNTPs (Fermentas, USA), 10 pM of each ribosomal gene-specific 18S forward (5`-AACGGCTACCACATCCAAGG-3`) and reverse (5`-TCATTACTCCGATCCCGAAG-3`) primers. The amplification conditions were: initial denaturation for 5 min at 95°C, followed by 30 cycles of 45 s denaturation at 94°C, 45 s annealing at 55°C and 45 s extensions at 72°C. Final extension step was at 72°C for 10 min.
Two SSR primers (ST-1) synthesized by (Metabion international AG, Germany) were used for detecting the functionality of the extracted DNA by this developed protocol. The PCR reaction (10 µl volume) contained about 50 ng of genomic DNA, 0.1 µl of blend taq polymerase (2.5 u/µl, Top-Bio s.r.o., Czech Republic), 2 µl 10× PCR reaction buffer, 0.1 µl MgCl2 (25 mM), 0.2 µl dNTP mixture (2.0 mM), 0.2 µl each primer (25 µM), and dH2O up to 10 µl. Amplification was carried out in a T100 BIO-RAD thermal cycler. Cycling conditions consisted of 5 min initial denaturation at 95°C, followed by 1 min denaturing at 95°C, 1 min annealing at 55°C and 1 min extension at 72°C repeated for 35 cycles and 5 min extension at 72°C. PCR products were subsequently separated by electrophoresis on 2% agarose gel at 70 V for 40 min, which was then stained with ethidium bromide (0.5 mg/ml) and photographed as described above by using 1.5 µl of 1 kb DNA ladder.
Data analysis
Data were analyzed on the DNA concentration and on the 260/280 and 260/230 ratios by agricolae package (de Mendiburu, 2020) using the R software version 3.5 (R Core Team, 2018). Tukey’s pairwise comparisons with the confidence interval of 95% were used to compare the concentration between the extraction methods.
This method largely follows those already developed for other plant species in the major steps, such as cell disruption, DNA extraction and precipitation (Kalbande et al., 2016; Paterson et al., 1993); however, there were modifications to the composition to the lysis buffer, which were intended to overcome the issues of high secondary metabolite content in the plant tissue. Specifically, a phenol-binding reagent (SDS) and NaCl were used to remove polyphenols and polysaccharides, respectively.
In addition, to reduce the cost and processing time of the procedure, all buffers were prepared from chemicals available in local stores.
The analyzed results for the ratio of UV absorption A260/280 and A260/230 and the concentration of DNA extracted by the different modified KCl, CTAB and SDS methods are presented in a box plot (Figures 1 and 2). The mean concentration and quality of DNA is presented in Table 2. Comparison of quality and quantity values (Table 2) indicated that there is no significant difference between different accessions on: the concentration (F = 0.34, df = 3, P = 0.08), A 260/280 ratio (F = 0.26, df = 3, P = 0.86), and the A260/230 ratio (F = 0.18, df = 3, P = 0.91). This contradicts with the principle that plant species belonging to the same or related genera can exhibit enormous variability in their biochemical composition and this heterogeneity may not permit optimal DNA yields from one isolation protocol and this leads to the development of different isolation protocol even for closely related genera. However, significantly different results were obtained comparing the different lysis buffers (Table 2) for A260/280ratio (F = 2.78, df = 6, P = 0.04) and for A260/230ratio (F = 16.44, df = 6, P = 0.00). Intactness and quality difference of the DNA extracts using the different lysis buffers is shown in Figure 3. The assessment of the purity of the DNA is confirmed by the A260/280 ratio. For a ‘pure’ nucleic acid, this value commonly resides in the range of 1.8 to 2.0 (Sambrook et al., 1989). The A260/280 ratios below approximately 1.3 and above 2.3 are indicators of poor quality of the DNA (Seth et al., 2018). Samples with absorbance ratio at A260/280 greater than 2 indicate the presence of carbohydrates and other secondary metabolites (Wilson and Walker, 2010). Higher values of absorbance ratios are evidence of contamination by phenols while lower values indicate the presence of proteins since proteins absorb light at a wavelength of 280 nm (Wilson and Walker, 2010). The presence of RNA in the sample has been also shown to increase the A260/280 ratio. RNA contamination can be confirmed or ruled out by agarose electrophoresis, in case of RNA being present in the sample; it has to be treated by RNase (Valledor et al., 2009).
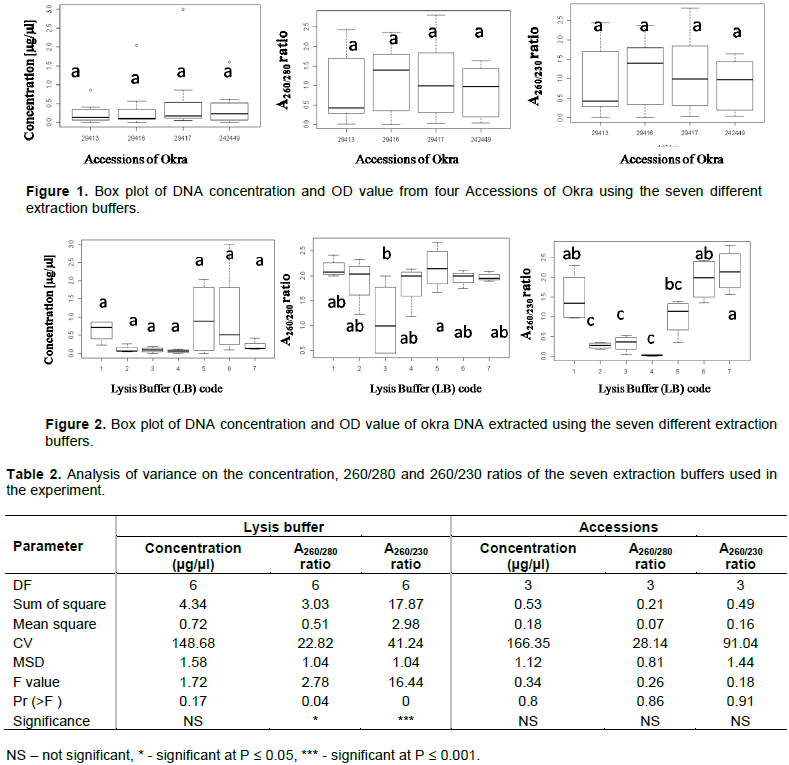
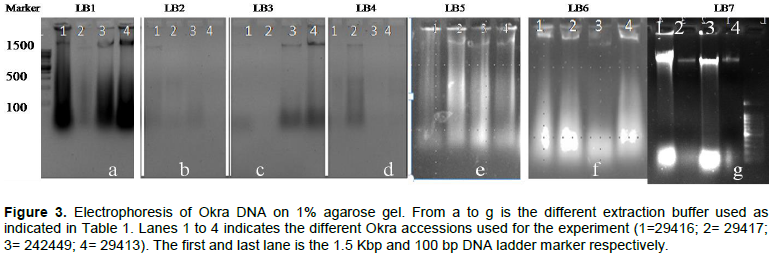
In this experiment, the majority of the lysis buffers resulted in the formation of smear on the gel, which indicated the degradation of the DNA (Figure 3). However, lysis buffer 7 (LB7) formed a relatively better band with less smear, which also hints at absence of RNA contamination (Figure 3). The absorbance ratio of A260/280 for the four tested samples ranged between 1.9 and 2.0. The values of all samples were within the accepted range, indicating a low level of contamination (ST-2). Similar work was also conducted for extracting DNA from samples with a high amount of polysaccharides and mucilage using SDS to replace CTAB extraction buffer (Sharma et al., 2018). SDS is an anionic detergent for cell and nucleus lysis to release ribonucleic and deoxyribonucleic acids by inhibiting the nucleases, ribonuclease (RNase) and deoxyribonuclease (DNase) activities (Farrell, 2011). The resulting DNA was further used for SSR marker-based analysis (Figure 4).
CTAB based DNA extraction method is the most commonly used technique for different crop species. However, in our current experiments CTAB was not satisfactory in terms of quality and quantity of DNA with/without modification and with modification of CTAB extraction components like PVP, β- mercaptoethanol (Figure 3). The extraction of DNA from fresh okra samples using CTAB resulted in a thick and sticky substance, which could not be pipetted out of the Eppendorf tubes. This can be explained by the presence of polysaccharides in the DNA sample which form a highly viscous solution (Jeyaseelan et al., 2019). Mucilage is a highly viscous secondary metabolite composed of a polar polymer of glycoprotein that can co-precipitate with DNA and inhibits the action of Taq polymerase (Menu et al., 2018). Polysaccharides are problematic as they make the DNA unreliable during pipetting and hinder the activity of polymerases and restriction endonucleases (Kumar et al., 2018).
This study compared and optimized quality and speedy protocol which will be used for routine DNA isolation from okra (A. esculentus L. Moench) and is amenable for marker-assisted breeding, and high-throughput applications. In addition, this protocol may be used for other plant species that are recalcitrant to other methods due to their high levels of polysaccharides and polyphenols.
The authors have not declared any conflict of interests.
The authors are grateful to the Plant Cell Laboratory of Hawassa University, Hawassa, Ethiopia for providing facilities during the study period. The project is supported partially by Czech Development Agency project No. DP-2018-024-DO-11420.
REFERENCES
Agrawal A, Sharma A, Shukla NP (2016). Genomic DNA Extraction Protocol for Artemisia Annua L. Without Using Liquid Nitrogen and Phenol. International Journal of Applied Sciences and Biotechnology 4:448-451.
Crossref
|
|
Akotkar PK, De DK, Pal AK (2010). Genetic variability and diversity in okra (Abelmoschus esculentus L. Moench). Electronic Journal of Plant Breeding 1:393-398.
|
|
|
Ali Q, Salisu IB, Raza A, Shahid AA, Rao AQ, Husnain T (2019). A modified protocol for rapid DNA isolation from cotton (Gossypium spp.). Methods X 6:259-264.
Crossref
|
|
|
Allen GC, Flores-Vergara MA, Krasynanski S, Kumar S, Thompson WF (2006). A modified protocol for rapid DNA isolation from plant tissues using cetyltrimethylammonium bromide. Nature Protocols 1:2320-2325.
Crossref
|
|
|
Amani J, Kazemi R, Abbasi AR, Salmanian AH (2011). A simple and rapid leaf genomic DNA extraction method for polymerase chain reaction analysis. Iranian Journal of Biotechnology 9:69-71.
|
|
|
De Mendiburu F (2020). agricolae: Statistical Procedures for Agricultural Research. R package version 1.3-2.
View
|
|
|
Diizyaman E (1997). Okra: botany and horticulture. Horticultural Reviews 21(7).
Crossref
|
|
|
Dubey P, Mishra S (2017). A review on: Diabetes and okra (Abelmoschus esculentus). The Journal of Medicinal Plants Studies 5:23-26.
|
|
|
Farrell RE (2011). RNA Methodologies. In Reviews in Cell Biology and Molecular Medicine, (American Cancer Society). P. 74.
Crossref
|
|
|
Fronda BX, Gamboa M, Zabala JD, Oasan R (2018). Development of Vegetable Marmalade Using Okra's (Abelmoschus esculentus) Mucilage. In Abstract Proceedings International Scholars Conference pp. 91-91.
|
|
|
Gemede HF, Ratta N, Haki GD, Woldegiorgis WZ, Beyene F (2015). Nutritional quality and health benefits of okra (Abelmoschu sesculentus): a review. Journal of Food Processing and Technology 25:16-25.
Crossref
|
|
|
Harlan JR (1969). Ethiopia: A center of diversity. Economic Botany 23:309-314.
Crossref
|
|
|
Jeyaseelan TC, Jeyaseelan EC, De Costa DM, Shaw MW (2019). Selecting and optimizing a reliable DNA extraction method for isolating viral DNA in okra (Abelmoschus esculentus). Vingnanam Journal of Science 14.
|
|
|
Kalbande BB, Patil AS, Chakrabarty PK (2016). An efficient, simple and high throughput protocol for cotton genomic DNA isolation. Journal of Plant Biochemistry and Biotechnology 25:437-441.
Crossref
|
|
|
Khan GA, Yilmaz ND, Yilmaz K (2017). Okra Fibers: Potential Material for Green Biocomposites. In Green Biocomposites, (Springer), pp. 261-284.
Crossref
|
|
|
Kumar DS, Tony DE, Kumar AP, Kumar KA, Rao DBS, Nadendla R (2013). A review on: Abelmoschus esculentus (okra). International Research Journal of Pharmaceutical and Applied Sciences 3:129-132.
|
|
|
Kumar V, Prasad A, Roy C, Chattopadhyay T (2018). Validation of a Simple and Rapid Method for Isolating Genomic DNA from Medicinal and Aromatic Plants for Subsequent Polymerase Chain Reaction. International Journal of Current Microbiology and Applied Sciences 7:2562-2566.
Crossref
|
|
|
Lamont WJ (1999). Okra-A versatile vegetable crop. HortTechnology 9:179-184.
Crossref
|
|
|
Lee CS, Chong MF, Binner E, Gomes R, Robinson J (2018). Techno-economic assessment of scale-up of bio-flocculant extraction and production by using okra as biomass feedstock. Chemical Engineering Research and Design 132:358-369.
Crossref
|
|
|
Mahuku GS (2004). A simple extraction method suitable for PCR-based analysis of plant, fungal, and bacterial DNA. Plant Molecular Biology Reporter 22:71-81.
Crossref
|
|
|
Martin FW (1982). Okra, potential multiple-purpose crop for the temperate zones and tropics. Economic Botany 36:340-345.
Crossref
|
|
|
Menu E, Mary C, Toga I, Raoult D, Ranque S, Bittar F (2018). Evaluation of two DNA extraction methods for the PCR-based detection of eukaryotic enteric pathogens in fecal samples. BMC Research Notes 11:206.
Crossref
|
|
|
Paterson AH, Brubaker CL, Wendel JF (1993). A rapid method for extraction of cotton (Gossypium spp.) genomic DNA suitable for RFLP or PCR analysis. Plant Molecular Biology Reporter 11:122-127.
Crossref
|
|
|
Porebski S, Bailey LG, Baum BR (1997). Modification of a CTAB DNA extraction protocol for plants containing high polysaccharide and polyphenol components. Plant Molecular Biology Reporter 15:8-15.
Crossref
|
|
|
R Core Team (2018). R: A language and environment for statistical computing. R Foundation for Statistical Computing, Vienna, Austria. URL
View
|
|
|
Sambrook J, Fritsch EF, Maniatis T (1989). Molecular cloning: a laboratory manual. (Cold spring harbor laboratory press).
|
|
|
Seth T, Mishra GP, Singh B, Kashyap S, Mishra SK, Tiwari SK, Singh PM (2018). Optimization of quality DNA isolation protocol from various mucilage rich cultivated and wild Abelmoschus sp. and its validation through PCR amplification. Vegetable Science 45:1-6.
|
|
|
Sharma S, Kumar A, Dobhal S, Rawat A (2018). Standardization of protocol for genomic DNA isolation in Melia composite Benth. (SM dubia Cav.).
|
|
|
Singh V, Kumar V (2012). An optimized method of DNA isolation from highly mucilage rich okra (Abelmoschus esculentus L.) for PCR analysis. Advances in Applied Science Research 3:1809-1813.
|
|
|
Valledor L, Hasbún R, Rodríguez R and Canal MJ (2009). RNA-free DNA Extraction Protocol from Pinus Tissues for Molecular Biology or HPCE/HPLC Analyses. Journal of Plant Biochemistry andBiotechnology 18(2):229-232.
Crossref
|
|
|
Waghela M, Khan S (2018). Isolation, Characterization of Pigment Producing Bacteria from various food samples and testing of antimicrobial activity of bacterial Pigments. DAV International Journal of Science 7.
|
|
|
Wei D, Guo S, Shulan SU, Qian D, Zhu Z, Shang E, Geng Z, Duan J (2017). Protective effect of okra seed oil on acute gastric ulcer in mice. Journal of China Pharmaceutical University 48:334-342.
|
|
|
Wilson K, Walker J (2010). Principles and Techniques of Biochemistry and Molecular Biology (Cambridge University Press).
Crossref
|
|
|
Xia Y, Chen F, Du Y, Liu C, Bu G, Xin Y, Liu B (2019). A modified SDS-based DNA extraction method from raw soybean. Bioscience Reports 39.
Crossref
|
|
|
Zhang T, Xiang J, Zheng G, Yan R, Min X (2018). Preliminary characterization and anti-hyperglycemic activity of a pectic polysaccharide from okra (Abelmoschus esculentus (L.) Moench). Journal of Functional Foods 41:19-24.
Crossref
|
|