The global quest for production of biofuel at a competitive price with fossil fuel has driven research to the exploration of organic waste as a cost-effective growth medium for microalgal biodiesel production. In this study, poultry waste extract (PWE) was explored as growth medium for efficient biomass and some intracellular content accumulation by Chlorella sp. isolate for eight days. The analysis of the poultry waste (PW) revealed high concentrations of nitrogen (5.785%) and phosphorus (6.786 mg/L) minerals alongside magnesium, iron, zinc, potassium, manganese etc. Under phototrophic culture condition, 200 g/L PWE among the various PW concentrations tested elicited higher biomass and productivities than other PWE concentrations tested. PWE (200 g/L) yielded comparable cell biomass and lipid productivity (2.61±0.002 g/L) (0.143±0.0006 g/L/day) with the control (BG 11 medium) (3.55±0.0008 g/L) (0.152±0.004 g/L/day). White light or white+yellow lights were the most efficient in stimulating cell growth and lipid production. However, yellow light triggered significantly (P < 0.05) higher accumulation of chlorophyll-a content (9959 µg/ml) than white+yellow light (6479 µg/ml) at 200 g/L PWE concentration and more than white light at 400 g/L PWE concentration. The outcome of these research holds high prospect for simultaneous production of biolipid and chlorophyll-a by Chlorella sp using a cheap growth medium for cost-effective biodiesel production.
Fossil fuels are valuable one-time energy gift to the human race and which are subject to depletion. Because energy is an indispensable need of life evidenced on world energy consumption reports (Brennan and Owende, 2010), man has resorted to finding an alternative source of energy for its continued existence. The adverse effect of fossil fuels on the environment such as greenhouse gasses emissions (Zhu et al., 2015) and climate change has triggered global concern and necessitated the search for an environmentally friendly
alternative energy resource which cannot be depleted as the fossil fuels. Therefore, renewable energy which is environmentally friendly has become the global focus in terms of energy security to replace the current fossil fuels. The renewable energy such as wind energy, solar energy and bioenergy are environmentally friendly energy sources and help to reduce the greenhouse gas emission (Lam and Lee, 2012; Huang et al., 2010). Biodiesel- a common example of bioenergy resource- has received greater attention in recent years globally. Biodiesel is non-toxic, renewable and biodegradable and is considered as one of the most alternative fuels for diesel engines. Biodiesel is prepared from various sources including edible oils, non-edible oils, animal fats, legume plants, microalgae etc. The commercialization of biodiesel has not been achieved due to high cost of production which makes the product more expensive than fossil fuel. Hence, research has focused on cost-effective biodiesel production to make it competitive with fossil fuels. One of the effective ways to reduce the cost of biodiesel production is to use inexpensive and non-edible oils as feedstock. Currently, microalgae are considered as one of the most promising feedstock for biodiesel production. This is because microalgae can reproduce rapidly due to their simple cellular structure and high lipid productivity (Lam and Lee, 2012, 2014), and potential to grow in waste-formulated medium that contains nitrates and phosphates. Cultivation of microalgae in waste-formulated medium helps to minimize the cost of energy needed and as such reduces the cost of biodiesel production by microalgae. Waste such as compost contains high concentration of nutrient such as nitrate and phosphate (Lam and Lee, 2012; Ma and Hanna, 1999), which promote the growth of microalgae and economically improve microalgae biodiesel production (Zhu et al., 2015; Zhou et al., 2014). Cultivation of microalgae cells are usually influenced by pH, amount of nutrient added and types of compost. Therefore, this research is undertaken to investigate the potential of compost nutrient derived from poultry waste to grow microalgae isolate and to optimize isolate’s biomass production as well as accumulation of some intracellular contents via batch cultivation method and varying qualities of light illumination.
Medium composition and preparation
Blue-Green (BG) 11 medium
BG 11 growth medium was composed of (per litre): 0.25 g NaNO3; 0.04 g K2HPO4; 0.075 g MgSO4·7H2O; 0.027 g CaCl2·2H2O; 0.006 g C6H8O7 0.006 g C6H8O7.nFe.nNH3; 0.001 g EDTA; 0.02 g NaCO3; and 1.0 ml A5 + Co stock solution. The A5 + Co stock solution was prepared by dissolving (per litre): 2.860 g H3BO3; 0.222 g ZnSO4·7H2O; 1.81 g MnCl2.4H20; 0.079 g CuSO4·5H2O; 0.390 g Na2MoO4·2H2O; and 0.0494 g Co(NO3)2.6H20.
Poultry waste extract (PWE) medium
Dried chicken manure was collected from Phinomar farm at Ngwo in Enugu state. Foreign objects were removed from the sample and sun dried for easy grinding into powder. Using Han et al. (2017) protocol; A slurry of 50, 100, 200 and 400 g/L respectively of the poultry waste sample powder was made using distilled water and refrigerated at 4°C using a beaker sealed with foil for 12 h. The slurry was filtered each using Whatman no 1 filter paper and the filtrate stored at 4°C for use.
Inoculum preparation
About 10% stock culture of microalga isolate (Chlorella sp) was inoculated in 500 ml Erlenmeyer flasks containing 200 ml BG 11 medium and incubated under continuous white light illumination for 10 days.
Cultivation of microalga isolate
Effect of different poultry waste extract (PWE) concentrations and light qualities on the growth of microalga isolate
Erlenmeyer flasks (500 ml) each containing 200 ml BG-11medium (pH = 7.3) were grouped according to the poultry waste extract concentrations as follows: Group 1, 2, 3, 4, and 5 for the 50, 100, 200 and 400 g/L PWs and control (containing BG 11 medium) respectively. Each group was in triplicates and the flasks were covered with foam plugs for aeration.
Approximately 10% of the inoculum volume of isolate was aseptically transferred into the growth medium in triplicates for each of the culture groups. They were incubated in a rectangular box at 28°C under continuous illumination by white light, white light+yellow light, yellow light or without light (flasks were wrapped with aluminum foils) for eight days and agitated manually thrice a day. The distance of the culture flasks from the light source (15 Watts lamp) was about 1.5 cm. Cell growth and pH were measured in replicates using UV/VIS spectrophotometer and digital pH meter respectively for eight days. The lipid content, carotenoid content, and chlorophyll content were determined at the end of cultivation.
Determination of cell growth and dry biomass
An aliquot (5.0 ml) of culture broth was sampled on two-day intervals under sterile condition in a clean bench. The cells were washed twice in distilled water and cell optical density was determined by measuring the samples at 680 nm using UV-Vis spectrophotometer (OD680). The values were compared with standard calibration curves of optical density versus cell dry weight for the isolate.
Cell growth rate
The specific growth rate was calculated by the equation: μ (day-1) = 1/t ln (Xm/X0) Where Xm and X0 (g/L) were the dry biomass at the end and beginning of the exponential phase, t (day) was the time between the two measurements, respectively.
Dry biomass
The dry biomass accumulated after cultivation was determined by the equation: Dry biomass (g/L) = Xm-Xn where Xm and Xn (g/L) were the final biomass at the end of cultivation and initial biomass at the beginning of cultivation respectively.
pH determination
A homogenous sample was obtained using a 5 ml syringe from each flask, and transferred into a labelled glass test tube. The pH was determined using a pH meter at 2 days interval for 8 days and recorded accordingly for each sample.
Measurement of some intracellular contents
Determination of pigment content
An aliquot (5.0 ml) of culture broth was sampled and centrifuged at 5000 × g for 5 min, and rinsed twice with distilled water. The pellet was extracted with 5.0 ml 90% (v/v) methanol in a test tube wrapped with aluminum to prevent photo-oxidation at room temperature, and centrifuged at 5000 × g for 5 min. The supernatant was decanted into a separate test tube and the pigment content of the supernatant was determined by UV-VIS spectrometer (GENESYS 10S UV-Vis, Thermo Fisher Scientific, MA, USA) using the method of Lichtenthaler (1987) as follows:
Total carotenoid (mg/L) = (1000A470 – 44.76A666 / 221)
Ch-a = 16.72A645 – 9.16A645 (μg per ml solution)
Ch-b = 34.09A645 – 15.28A645 (μg per ml solution)
A = Absorbance, Ch-a = Chlorophyll a, Ch-b = Chlorophyll b. where 1000, 44.76, 221, 16.72, 9.16, 34.09 and 15.28 were constants (Lichtenthaler, 1987).
Measurement of lipid production
Drying and powdering biomass
The dry biomass obtained after drying the harvested wet biomass in a hot air oven at 70°C for 24 h was pulverized using mortar and pestle. The total lipid content of the microalgae sample was determined gravimetrically using method of Bligh and Dyer (1959). The total lipid content (g/g cell) was calculated thus:
Total lipid content (g/g cell) = (weight of lipid) g / (weight of powdered cell) g
Lipid productivity
The lipid productivity is a measure of the quantity of lipid accumulated by microalgae cell per day of cultivation and calculated thus:
Lipid productivity (g/L/day) = total lipid content (g/g cell) × dry biomass (g/L) / days of cultivation.
Poultry waste elemental analysis
Elemental analysis was conducted using Varian AA240 Atomic Absorption Spectrophotometer and standard method 4500-P B.5 and 4500-PE according to the method of American Public Health Association (APHA, 2005). Phosphate was measured using standard method 4500-P B.5 and 4500-PE (APHA, 2005). Nitrogen was measured using the method of Association of Analytical Chemists (AOAC) (1984).
Statistical analysis
The data obtained from comparison of poultry waste media concentration and the qualities of light illuminations in shake flask cultures were analyzed statistically using one-way analysis of variance (ANOVA), and the means were separated using Least Significant Different methods.
The mineral contents of fresh poultry waste
The elemental analysis of poultry waste revealed high concentrations of minerals such as nitrogen, phosphorus, potassium and some trace elements as shown in Table 1.
The effect of different concentrations of poultry waste (PW) extract on the cell biomass of microalga isolate
White light illumination produced the highest cell biomass of 2.60±0.002 g/L in 200 g/L PW medium, though lower than control (3.54±0.0008 g/L), while the least cell biomass (0.31±0.002 g/L) was produced by the cultures without illumination across the PW media concentrations tested (Figure 1). Also, the white+yellow light illumination produced higher cell biomass than the yellow light illumination only (Figure 1). Each of the PWE concentrations tested elicited comparable growth rates under white light and white+yellow light illuminations except 50 g/L PWE concentration which yielded the least cell growth rate under the different light qualities tested (Figure 2). Heterotrophic condition elicited the least cell growth rates in all the different PWE concentrations tested.
The effect of different concentrations of poultry waste (PW) under different qualities of light illumination on some intracellular contents of microalga isolate
The chlorophyll-a content of cells in 200 g/L PW cultures illuminated by white light (11849±1675 µg/ml) is higher than the values produced by other quality of light illuminations across the PW concentrations and the controls (Figure 3). Yellow light illumination elicited higher chlorophyll-a content in the isolate more than white+yellow light illumination across the PW concentrations except in 50 g/L PW medium (Figure 3). The culture without illumination (heterotrophic) yielded lower chlorophyll-a content than the illuminated cultures while the control and 100 g/L PW cultures produced higher contents of chlorophyll-a than the values obtained under white+yellow light illumination (Figure 3).
The accumulation of chlorophyll-b in the isolate was higher under white light illumination than other types of illuminations across the PW concentrations (Figure 4). The content was highest in 100 g/L PW medium (24034±1376 µg/ml) across the PW concentrations, although it was lower than the value produced by the control (28089±93 µg/ml). The cell cultures without light illumination yielded the least chlorophyll-b contents across the PW concentrations and the controls (Figure 4). The carotenoid content elicited by white light was higher than the values by other qualities of light illuminations for all the concentrations of PW media (Figure 5). The values produced by white+yellow light were higher than the value produced by yellow light only.
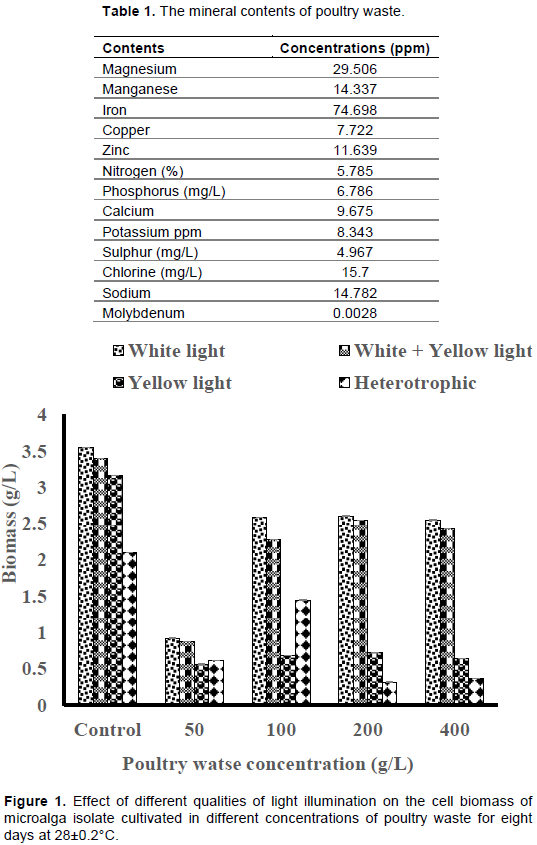
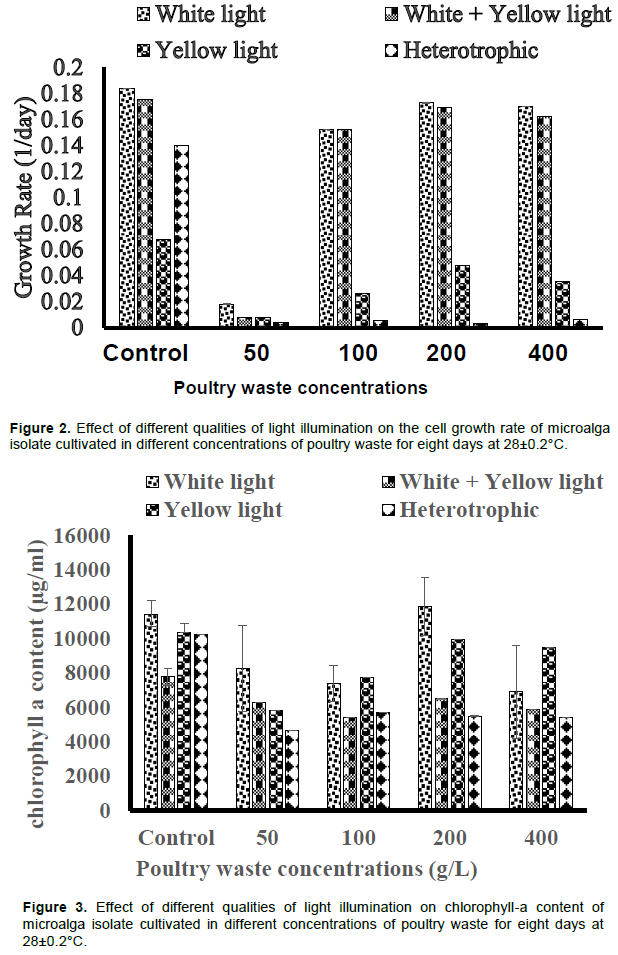
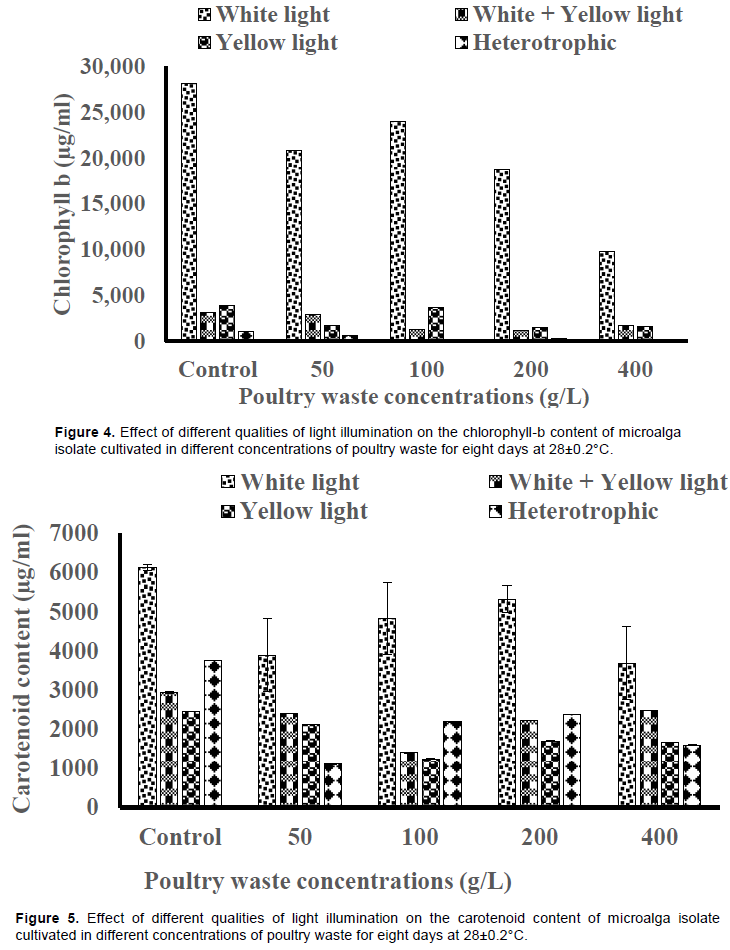
The 50 g/L PW medium without light illumination produced the least carotenoid content (1113±0.66 µg/ml) while the 200 g/L PW medium under white light illumination produced the highest carotenoid contents (5320±341 µg/ml) (Figure 5).
The lipid content varied across the different PW media concentrations and light illuminations. The value produced by white light illuminations was higher than the values produced by other type of illuminations across the PW media concentrations (Figure 6). The cultures without light produced the least lipid contents, while the values produced by white+yellow light was higher than that of yellow light only (Figure 6). The 50 g/L PW medium under white light illumination produced the highest lipid content of 47±0.60%.
The lipid productivity by white light was higher than the values by other light types. The 200 g/L PW medium yielded the highest lipid productivity of 0.143±0.0006 g/L/day although lower than the value produced by the control (0.152±0.004 g/L/day) (Figure 7). The white+yellow light yielded higher lipid productivity than yellow light only. The culture without light illumination yielded the least lipid productivity across the PW media concentrations and the control (Figure 7).
The high concentrations of nitrogen and phosphorus in the poultry waste suggest the potential of poultry waste for cultivation of microalgae. Han et al. (2017) also affirmed that Chicken manure contains rich amount of nitrogen and phosphorus and has been used as crop fertilizer. These nutrients are responsible for eutrophication in the aquatic environment following the discharge or run-off of the poultry waste into aquatic environments, and resulting in algal blooms and hypoxic conditions in aquatic ecosystems (Han et al., 2017). These nutrient elements such as nitrogen, phosphorus, magnesium, iron, copper, etc as contained in the poultry waste extract (Table 1) are known to support the growth of microalgae. Chisti (2007) reported that growth medium for algal cell cultures must constitute essential micronutrients such as nitrogen and phosphorus. According to Mobin and Alam (2014), three primary nutrients (carbon, nitrogen, and phosphorus) and a number of micronutrients such as silica, calcium, magnesium, potassium, iron, manganese, sulphur, zinc, copper, and cobalt are required for producing algal biomass.
The growth of the microalga isolate in the different concentrations of poultry waste extract for the period of eight days confirms the wide reports on the potential of organic wastewater as alternative growth medium for the cultivation of microalgae (Renuka et al., 2015; Bhatnagar et al., 2011; Frampton et al., 2013). Hence, poultry waste extract is viewed as a potential cost effective medium for cultivation of microalgae biomass. Algal companies can
save money and the environment if commercial fertilizer is replaced by Chicken manure (Han et al., 2017). Also, the growth of the microalga isolate in the poultry waste extract indicates the potential of the isolate for phycoremediation of wastewater since nutrients are removed from the medium. However, the quantities of nutrients were not assayed to determine the rate and extent of nutrient removal in this study. The variations in the cell biomass produced by different concentrations of the poultry waste (Chicken manure) extract are probably due to variations in the concentrations of nutrients contained therein. The increased concentration of PWE resulted in decreased dry cell biomass although there was no significant difference (p > 0.05) in the cell biomass produced by 100, or 400 g/L PWE. This report seems to differ from the report by Badawy (2008) that cell dry weight increased with waste concentration. However, the range of concentrations of waste tested in the studies, (0.5 - 4.0% against 0.2 - 0.6%) as well as the species of microalgae (Scenedesmus dimorphus against Chlorella sp) could account for the variation in the two reports. The control culture (BG 11) whose cell biomass was higher than the PWE concentrations tested may be due to optimum concentrations of the available nutrients (optimal N to P ratio) in the culture medium. However, this report differs from the report by Han et al. (2017) that chicken manure medium resulted in higher algal biomass than the standard medium BG11. The variation in the reports may be due to the difference in the physicochemical characteristics of the chicken manure media which directly or indirectly affect algal growth (Renuka et al., 2015). The difference in the microalgae species used as well as other environmental parameters such as light intensity may be implicated.
The reason for the significant increase in chlorophyll-a content (p < 0.05) by 200 g/L PWE above the values produced by other PWE concentrations is not known. However, it is widely known that phototrophy enhances the accumulation of pigments in microalgae (Eze et al., 2017). The significant increase in carotenoid content by the control above the test experiments (p < 0.05) is probably as a result of variations in light penetration into the cultures. The PWE media were colored which may have limited the quantity of light penetrating into the cultures thereby impacting negatively on carotenoid induction by the microalga isolate.
The different qualities of light impacted on the isolate differently in terms of cell growth and intracellular content accumulation. The reason for the non-significant difference in the cell growth rate and biomass of isolate by white light or white+yellow light is not known. However, the higher cell growth rate and biomass provided by white light (p< 0.05) agrees with the report of Wong (2016). The report of maximum dry biomass of 1353.33 mg/L at cool white light of 110 μmol m-2 s-1 during the 7th culture day (Wong, 2016), was lower than the value obtained in this study across the PWE media concentrations by white light, and white+yellow light illuminations except for 50 g/L PWE medium. However, the light intensities were not determined in this study. The non-significant difference (p > 0.05) in cell growth and intracellular contents by yellow light and no light illuminations as observed in the present study probably suggest that the yellow light intensity was very poor and was not able to penetrate the culture to impact on the algal cells. Nwoba et al. (2016) also acknowledged that the lower cell density under the blue spectra would be due to reduced irradiance from the blue filter. However, significant decrease in the cell biomass and productivities of the Chlorella sp. grown in the dark (no light) was not unexpected because all photoautotrophic microalgae would require light as a source of energy for their growth and productivities. The significant positive impact of yellow light illumination only on the chlorophyll-a content of the isolate more than yellow+white light or no light illumination probably suggest that specific productivities by microalgae could be specific to the spectral quality of illumination. The economic implication of this finding is that when yellow spectrum is filtered for the production of chlorophyll-a, the remaining portions of the spectrum can be collected and redirected to a highly efficient photovoltaic cell with little or no loss of energy (Nwoba, 2017). The report of maximum lipid productivity using white light (Wong, 2016) is in agreement with the present report although the value reported was lower than what was obtained in the present study (0.14367 g/L/day).
This study demonstrates that nutrients extracted from chicken manure (poultry waste) contain sufficient nitrogen and phosphorus to support fast growth, high biomass and lipid production by microalga Chlorella sp. The Chlorella sp. isolate has the potential for cost-effective biodiesel production using poultry waste medium. Also, white light was the most efficient in stimulating the production of biomass, carotenoid and lipid in phototrophic condition while yellow light was the most efficient in stimulating chlorophyll-a content in phototrophic condition by the Chlorella sp. isolate.