ABSTRACT
The efficacy of two microbial isolates, Bacillus spp. (B285) and Saccharomyces spp. yeast strain (Y833), in reducing aflatoxin concentration in poultry feeds in comparison with the commonly used commercial chemical binder, bentonite, was investigated using the VICAM ® fluorometer. The influence of the poultry feed matrix, pH (4.5 and 6.5), and temperature (room temperature, 37 and 42ºC) on the aflatoxin reducing activity by the two microorganisms was also explored. All microorganisms and bentonite reduced aflatoxins by over 74% of the original concentration. Bentonite registered the highest reduction at 93.4%; followed by Y833 (83.6%), then the combination of Y833 and B285 (77.9%); and lastly B285 (74.9%). Temperature and pH did not have significant effect on the performance of the biological agents and bentonite. The aflatoxin reducing activity was lower in presence of feeds compared to that in phosphate buffered saline except for Y833. The yeast strain was more effective than the bacterial strain in reducing the aflatoxin levels; however, both are promising strategies for countering the aflatoxin challenges in animal feeds. In response to the advocacy for use of biological control agents, there is need for more investigations to establish the safety of the microorganisms and the mechanism of aflatoxin decontamination.
Key words: Aflatoxin decontamination, Saccharomyces, Bacillus, commercial binder, animal feeds, pH, temperature.
Consumption of contaminated foods of animal origin has been linked to increasing incidences of human illnesses and deaths affecting about 1 in 10 people worldwide (FAO et al., 2019). The safety of food and feed is compromised by contamination with different materials including chemically active fungal metabolites, especially aflatoxins. Aflatoxins are a large group of secondary metabolites that are highly toxic and carcinogenic. The toxins are produced by specific species of fungi, that is, Aspergillus flavus, Aspergillus parasiticus and Aspergillus nomius; which are ubiquitous in soil, decaying vegetation, hay and grains (Coppock et al., 2018). Aflatoxins associated with major detrimental effects are B1, B2, G1, and G2; with aflatoxin B1 being the most potent hepatocarcinogen known (Rajarajan et al., 2013). Aflatoxin B1 is also reported to be mutagenic, teratogenic and estrogenic (Benkerroum, 2020).
Aflatoxins occur in most of the staple foods produced in Africa such as maize, sorghum, groundnuts, peanuts and cotton seed (Osuret et al., 2016). The contamination is associated with high moisture content, ranging from 18 to 18.5% in the grains. Unfortunately, subsequent processing steps, such as drying and boiling, do not reduce the aflatoxin levels (Benkerroum, 2020; Khaneghah et al., 2018). The European Economic Community (EEC) as well as the United States Food and Drug Authority (FDA) set the maximum permitted levels of total aflatoxins in poultry feed as 20 µg/kg (Lubna et al., 2018), while the Uganda National Bureau for Standards (UNBS) set a limit of 10 µg/kg for all foods and feeds but only those intended for export (Grace et al., 2015).
In Uganda, reports of persistent occurrence of aflatoxin contamination, beyond acceptable levels, in selected food and feedstuffs exist (Lukwago et al., 2019; Omara et al., 2020). Indeed, a previous study in Uganda revealed poultry feed contamination with aflatoxins at levels between 7.5± 0.71 and 393.5 ± 19.09 µg/kg; with only 17.9% passing the FDA limits (Nakavuma et al., 2020). Unfortunately, there is limited monitoring and enforcement of the regulations; in addition to unsuccessful strategies for management of mycotoxin contamination in the animal feed industry in Uganda.
Management of aflatoxin contamination in the food/feed chain calls for improvement in the farming practices, pre- and post-harvest handling; with the latter emphasizing appropriate storage of the grains and processed feeds (Pankaj et al., 2018). These strategies require additional treatments to effectively eliminate aflatoxin contamination. Therefore, further efforts in development of safe, effective, affordable and environmentally safe aflatoxin decontamination methods are required (Mwakinyali et al., 2019). The most widely used approach in the detoxification of feed and food is the application of sorbents for selective removal of toxins by adsorption before and during passage through the gastrointestinal tract (Ismail et al., 2018). Aflatoxin binders adsorb the toxin while in-situ, in the feed and in the gut resulting in the excretion of the toxin-binder complex in the faeces (Boudergue et al., 2009). Inorganic binders, such as hydrated sodium calcium Aluminosilicates (HSCAS), bentonites, phyllosilicates, smectites, kaolinites, zeolites and activated charcoal are used especially in developed countries (Womack et al., 2014). Bentonite is among the most widely used sequestering agents and has been reported to adsorb over 85% of aflatoxins present in the feed (Gan et al., 2019; Mgbeahuruike et al., 2018; Wang et al., 2020) and was therefore included in the current analyses for comparison purposes. The effectiveness of inorganic substances varies with the toxin type; and in some cases, there is need to eliminate them from the treated feeds before presenting to the animals (Kim et al., 2017). Hence, investigations into the effectiveness and feasibility of applications of biological materials are recommended as alternative strategies for aflatoxin decontamination in feeds (Ismail et al., 2018).
Several microorganisms including bacteria, yeast, and non-toxigenic strains of A. flavus and A. parasiticus have exhibited aflatoxin detoxification capabilities and are thus promising as alternatives to chemical binders (Ismail et al., 2018). Biological detoxification, which is due to biotransformation or degradation of the toxin by the microorganism or its enzymes, yield metabolites that are either non-toxic when ingested by animals or less toxic than the parent toxin molecule (Boudergue et al., 2009). Despite considerable research on microbial detoxification and their premise on utilization in feeds and foods (Kim et al., 2017), they are not widely available, especially in developing countries, such as Uganda. Besides, given the regulatory rigour on introduction of microbes into a country and the biosecurity issues, there is need to develop products from sources obtained from the local environment. This study aimed at evaluating the efficacy of two locally isolated microbial strains in reducing aflatoxin concentration in poultry feeds in comparison to the commercial chemical binder, bentonite. The influence of the feed matrix, pH, and temperature on the aflatoxin reducing activity of the microbes and bentonite was also determined.
Study design and source of microorganisms
An experimental laboratory-based study that followed a factorial design was carried out to establish the efficacy of two microbial strains in reducing aflatoxin concentrations in poultry feeds. The microbes were obtained from previous studies that involved in vitro screening for aflatoxin reduction activity of isolates from various sources in Uganda. A commercial binder, bentonite, was included as the positive control. Bentonite, as well as the specifications for its use, was obtained from local traders in Kampala, Uganda. The microbes included a bacterial strain (Bacillus spp B285) and a yeast Saccharomyces spp strain (Y833) that were isolated from poultry droppings and brewer’s waste, respectively.
Preparation of the aflatoxin-reducing microbial test materials
Stock cultures of the microbial isolates, Bacillus spp (B285) and a Saccharomyces spp strain (Y833) were resuscitated in Trypticase Soy Broth (TSB) and Czapek Dox Broth (CDB), respectively. Purity of the isolates was confirmed by sub-culturing on Trypticase Soy Agar (TSA) and Potato dextrose agar (PDA) for the bacteria and yeast, respectively. All media were manufactured by Laboratorios Conda S.A, Spain. Working cultures were prepared by inoculation into the respective broth medium and incubated at 37ºC for 18 h for bacteria and at room temperature for 5 days for yeast.
From the overnight microbial broth culture, 2.0 ml were centrifuged at 3000 rpm (Zentrifugen, Germany) for 15 min at 10ºC. The cells were washed twice with PBS (pH 7.2) and once with double distilled water; and the cell pellet was suspended in 1.0 ml of sterile double distilled water. The microbial preparation was standardized to about 106 CFU/ml for yeast and 108 CFU/ml for bacteria by comparing with McFarland standard No.5. The viable bacterial and yeast cell concentrations were confirmed by surface spread method and expressed as colony-forming units per millilitres (CFU/ml).
Compounding of the poultry feed
Freshly supplied raw materials for feed preparation were purchased from produce dealers in Kampala. The ingredients were used in compounding of about 2 kg of broiler starter feed following an in-house formulation at the Nutrition Laboratory, College of Veterinary Medicine, Animal Resources and Biosecurity (COVAB), Makerere University. The ingredients and proportions included: cotton seed cake – 300 g, silver fish meal (Rastrineobola argentea, locally known as “mukene”) – 240 g, bones – 120 g, maize – 1230 g, salt – 10 g and soya – 100 g. The ingredients were ground separately and mixed to obtain the required compounded feed. From the compounded feed, 250 g were ground further using a laboratory mill to pass No.20 and then No.14 sieve. The feed was mixed thoroughly for homogeneity and placed in paper bags and stored at refrigeration temperature. The compounded feed was used for the aflatoxin -reducing experiments.
Source of aflatoxins for feed sample spiking
In-house production of aflatoxins was carried out following the methods described by Shotwell et al. (1966) with modifications. Coarsely ground rice was used as the substrate instead of the kernels. The inoculated sterile rice slurry was incubated for 7 days at 25°C in the dark. An aflatoxigenic A. flavus strain Y20, was obtained from the National Agricultural Research Laboratory, Kawanda.
Aflatoxin extraction was made following the procedure by Kana et al. (2013); with modifications; and all procedures were carried out in a fume hood. Briefly, 50 g samples of the rice culture was blended with 250 ml of methanol:water mixture (60:40 w/v) for 5 min at a high speed. The solution was filtered through Whatman No. 1 filter paper (Sigma-Aldrich, Germany). To the filtrate, 30 ml of 2% sodium chloride solution and 500 ml n-hexane were added, placed in a separating funnel, and shaken vigorously for 5 min. The n-hexane layer was discarded and the lower methanolic layer was placed into another separating funnel to which 40 ml of chloroform was added; shaken and allowed to rest undisturbed for separation of layers. The chloroform layer was collected in a flask containing 5 g of cupric carbonate, agitated, and allowed to settle down. The extract was filtered through a bed of anhydrous sodium sulphate over a filter paper; the various portions were pooled and then evaporated to dryness on a heat block. The residue was dissolved or reconstituted in 1 ml acetonitrile, to have a crude extract that was kept in a refrigerator until further analysis or use. Presence of aflatoxins in the crude extract was confirmed using thin layer chromatography (TLC) plates (Sigma- Aldrich, Germany) alongside 0.04 µg/ml aflatoxin B1, 0.024 µg/ml aflatoxin B2, 0.03 µg/ml aflatoxin G1 and 0.04 µg/ml aflatoxin G2 (Romer Labs, UK) as the aflatoxin standards. The bands were visualised under UV light at 365 nm wavelength.
Further purification of the aflatoxin extract was achieved by passing it through an Aflatest Immunoaffinity column (VICAM, Milford-Massachusetts, USA) following the manufacturer’s instructions. The column was washed with PBS and the toxins eluted with 100% High Performance Liquid Chromatography (HPLC) grade methanol. Quantification of aflatoxin content was achieved using VICAM®, a fluorometric method following the manufacturer’s instructions.
Preparation of the aflatoxin working solution involved evaporation of the methanol by keeping the loosely closed vials at 50°C for overnight, after which the concentration was adjusted to 1 µg/ml using PBS. The working solution was kept in an amber bottle in the refrigerator.
Preparation of aflatoxin spiked feeds and non-spiked feeds
Preparation of the feeds was as described by Manafi et al. (2009), with modifications, where 10 g of compounded feed instead of 25 g were employed and pH of the feeds was not modified. Briefly, to 10 g of the compounded poultry feed, 0.8 g of sodium chloride were added and mixed properly. A slurry was prepared by adding 10 ml PBS (pH 7.2) and then autoclaved. To 10 ml of the feed slurry, the aflatoxin working solution was added to obtain a concentration of about 40 µg/L (Gul et al., 2017), that is, 400 µL of 1 µg/ml aflatoxin working solution were added. To ensure proper distribution, small amounts of the feed slurry were continuously mixed with the aflatoxin working solution and homogenised. For non-spiked feeds, instead of aflatoxin solution, 400 μL of PBS was added.
Determination of the effect of feed matrix, pH and temperature on aflatoxin reduction
A factorial study design was used to evaluate the aflatoxin reducing efficacy of the two selected microorganisms and the control (bentonite) in aflatoxin-contaminated feed in vitro and under simulated in situ gastrointestinal tract environment (pH and temperature) of chickens according to Manafi et al. (2009). However, in this case, evaluation of the effects of pH and temperature were carried out in PBS not the feed. For each treatment, the toxin reduction was tested in triplicates at pH levels of 4.5 and 6.5; and at 37, 42ºC and at room temperature; after which the residual aflatoxin concentrations were determined.
To the aflatoxin-spiked and non-spiked feed samples, the appropriate test reducing agents were added. For bentonite, 0.3% w/v was added according to the instructions given by the local agrochemical supplier and Magnoli et al. (2008). For the microbial agents, 5 mL of the standardized yeast and bacterial cell suspensions with concentration of 1.0 × 106 and 1.0 × 108 CFU/mL, respectively, were used. In order to determine the effect of the feed matrix, PBS instead of feeds was used. The tests were carried out in triplicates and incubated at the appropriate temperatures for four hours. The experimental set-up is detailed below and summarized in Table 1.
For comparison of the activity of the test materials, the treatment groups included aflatoxin spiked feeds with yeast (Group 1); non-spiked feed with yeast (Group 2); spiked feed with bacteria (Group 3); non-spiked feeds with bacteria (Group 4); spiked feeds with 50% yeast and 50% bacteria (Group 5); and non-spiked feeds with 50% yeast and 50% bacteria (Group 6). In order to determine the effect of the feed matrix, the same set up was used, however, PBS instead of feeds was used. For positive control, bentonite was added, while for the negative control, nothing was added to the spiked or non-spiked feeds.
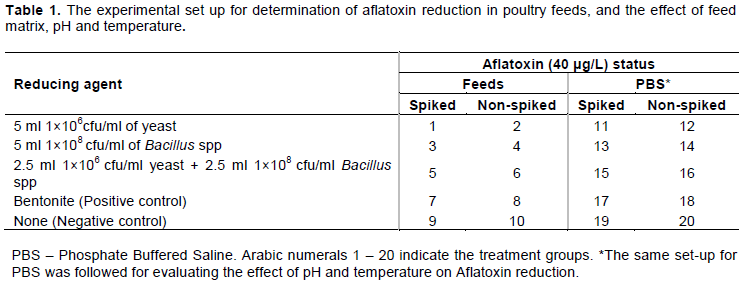
For determination of the effect of pH on aflatoxin reduction, the pH of PBS was adjusted to 4.5 and to 6.5; one portion was spiked with aflatoxins, while the other was not. The test materials were then added following the same set-up as for the feed experiments. For the effect of temperature, PBS experimental set up as described above was employed. Two sets, one for incubation at 37°C and the other at 42°C were put-up. Positive and negative controls involved addition of bentonite (positive) or nothing (negative) to the spiked and non-spiked preparations (Groups 7, 8, 9, 10; and Groups 17, 18, 19 and 20, respectively).
Residual aflatoxin concentration was determined after 4 h of exposure. For each treatment, aflatoxin extraction and quantification followed a procedure described by Kana et al. (2013), using the VICAM ® fluorometric method according to the manufacturer’s instructions. Absolute values of the aflatoxin concentrations for all the treatments were recorded.
For safety precautions, the cultures for aflatoxin production were autoclaved and later treated with 2% sodium hypochlorite before incineration. Likewise, all the plastic ware and glassware were soaked overnight in sodium hypochlorite before disposal or washing for reuse. All preparations were carried out in a Class II Biological Safety Cabinet (Faster sil, Ferrari, Italy).
Data analysis
Using the absolute concentration values, percent aflatoxin reduction was calculated from the amount of unbound (residual) aflatoxins extracted after the experiments compared to negative control (without reducing agent). The formula below was employed.
Data were analyzed by STATA v12 and the differences between the treatments were determined using ANOVA.
Reduction of aflatoxin concentration in poultry feeds subjected to the different agents
Results of aflatoxin reducing activities in poultry feeds are presented in Table 2. It was impossible to locally obtain aflatoxin-free ingredients for feed formulation; thus, the non-spiked feed contained 1.55±0.001 µg/L total aflatoxins. Addition of 0.3% (w/v) bentonite (positive control) to aflatoxin-spiked feed yielded a 93.43% reduction of total aflatoxin concentration, while the addition of the same to a non-spiked feed sample yielded a 100% removal of the toxins present. Mean aflatoxin concentration in presence of bentonite was significantly (p<0.00001) lower compared to that in absence of the binder.
Yeast was the most effective biological agent with a mean aflatoxin reduction from 41.53 ± 0.062 to 6.80 ± 0.002 µg/L giving a percentage reduction of 83.7%. The combination of yeast and Bacillus spp produced a higher percentage reduction (77.8%) compared to use of Bacillus spp (74.9%) only. The aflatoxin reduction by biological agents followed the trend of Yeast > Yeast + Bacillus spp > Bacillus spp. In non-spiked feeds, the biological agents reduced the aflatoxins to undetectable levels and hence produced 100% reduction from 1.549±0.001 to 0.00 (undetectable levels).
All test materials reduced aflatoxin concentration in feeds by more than 74%. However, the commercial binder, bentonite, had the highest reduction percentage (93.4%) followed by the yeast isolate with 83.7%; and lower values were recorded where bacteria were added.
Effect of feed matrix on aflatoxin reduction capacities of the test agents
Results indicating the effects of feed matrix on aflatoxin reduction activity of the different test agents are presented in Table 3. Aflatoxin reducing activity was lower by 3 and 8% in the presence of feeds as compared to PBS, for bacteria and bacteria plus yeast test materials, respectively. No differences were observed in case of the yeast isolate while bentonite performed better in presence of feeds than in PBS. The bacterial strain had lower activity compared to the yeast strain, when used singly; however, higher performance was observed in PBS, when combined with the yeast. Performance of bentonite was higher than the biological materials by 9-18% and by 5-13% in presence of feeds and in PBS, respectively. There were significant differences in aflatoxin reducing activity in the presence of feed (p < 0.001) and in PBS (p < 0.0001).
Effect of pH and temperature on aflatoxin reduction capacities of the agents
Aflatoxin reduction activities of the test agents at various temperatures and pH are presented in Figure 1. The percentage reduction ranged from 74 to 96%. Generally, the aflatoxin reduction activity for all the materials at different physicochemical conditions was above 70% with the commercial binder having > 90% in all cases. The yeast strain performed second best, with 83 – 86% reduction; and the Bacillus spp showed the least activity (74 – 78%) except at 42°C, where 96% reduction was observed. The bacteria and yeast combination exhibited 77 - 80% reduction. Aflatoxin reduction increased with rise in temperature, although it was not significant (p = 0.0940).
There was no significant effect (p = 0.9817) of pH on aflatoxin reduction by the various test materials. However, bentonite had the highest activity (95%) at pH 6.5. For the other treatments, there was a 1% reduction in activity at pH 6.5 compared to that at pH 4.5.
The continuous search for effective and appropriate mycotoxin control strategies has biological control as a promising strategy due to the associated efficiency, minimal effect on feed and/or food quality and safety; and environmental friendliness. This study screened two microbial agents for in vitro aflatoxin reduction activity at a single concentration. The selected strains, one of each of bacteria (Bacillus) and yeast reduced aflatoxin concentrations in contaminated poultry feed by 75 - 83.7%. The duration of exposure used was informed by the transit time of feed on ingestion, while the total aflatoxin concentration was twice the FDA recommended levels.
The yeast strain Y833 exhibited similar reduction activity in both feed and PBS, which fits in aflatoxin decontamination activities of 40 - 99.3% reported by previous researchers (Kana et al., 2013; Manafi et al., 2009; Shotwell et al., 1966). Various species including Saccharomyces cerevisiae and Candida krusei were shown to bind > 60% of aflatoxins in a study that determined the binding efficacy of the yeast isolates (Gul et al., 2017). The reduction activity of Y833 was comparable to what was reported for the yeast cell wall and brewery dehydrated residues; but lower than the autolysed and the dried cane sugar yeasts at almost similar conditions as the current study (Manafi et al., 2009). Lower reduction activity was reported by some researchers, but the measurements were made at or beyond six hours post exposure, such as 60 - 72.8% AFB1 reduction by selected Saccharomyces spp. (Assaf et al., 2019; Chlebicz and Åšliżewska, 2020; Magnoli et al., 2008). The decontamination activity depends on the yeast strains, pH and temperature conditions, duration of exposure and initial aflatoxin concentration (Gonçalves et al., 2014; Mukandungutse et al., 2019; Shetty and Jespersen, 2006). The reversible binding of aflatoxins by the yeast influences the detoxification levels, which have been reported to reduce with time (Manafi et al., 2009). An alternative mode of decontamination, through both intracellular and extracellular biodegradation by yeasts, such as Candida versatilis was previously reported (Li et al., 2018). The current study did not evaluate the activity over a period of time, which could have offered a clue on the mechanism of decontamination; hence, there is need for determining the mode of action of the selected isolates.
The aflatoxin reducing activity of the Bacillus spp. strain coded B 285 from this study is in agreement with findings by previous researchers who reported activities up to 97.3%, depending on the bacterial strain as well as the conditions and duration of the experiment (Petchkongkaew et al., 2008; Siahmoshteh et al., 2017; Wang et al., 2018). Our findings are similar to those of Petchkongkaew et al. (2008); who reported Bacillus lichenformis exhibiting 74% while Bacillus subtilis had 85% reduction of aflatoxin B1 at 37°C after 48 h of exposure, although the current exposure lasted 4 h only. The mode of activity by the Bacillus spp, is more of biodegradation than adsorption, which despite the different duration of exposure, results in comparable level of activities. However, like for the yeast studies, the analyses did not explore the effect of time on the decontamination activity.
In this study, the combined microbial activity (77.8%) was lower than that exhibited by the yeast strain (83.7%) but higher than that for bacteria (75%). The lower activity of the combined microbes than yeast is likely to have been due to the reduced amount of yeast cells in the preparation, however, this contributed to a higher activity than what was experienced by the bacterial strain. A higher effect compared to that by each strain was expected but the different mode of aflatoxin decontamination, and reduced concentration of each microbe probably affected their activity. These current findings contrast those from a previous study where degradation rates of 38.38 and 21.08% when B. subtilisand Candida utilis were used singly, respectively; and a much higher rate of 45.49% by a combination of the two microbial species (Karaman et al., 2005). The lower activity compared to yeast alone, was probably due to some growth-inhibitory activity of Bacillus spp against the yeast in addition to the lower concentration, thus affecting the aflatoxins decontamination. Determining the mode of decontamination, and the level of aflatoxins against time and microbial cell population can elucidate the combined microbial activity to obtain conclusive explanations.
Bentonite exhibited highest reduction, followed by yeast, then a combination of yeast and Bacillus spp, while the latter was the least active. Compared to bentonite, the microbes exhibited lower reduction activity. Vekiru et al. (2015) reported similar findings where sodium bentonite showed the strongest binding capacity for aflatoxin B1. Bentonite is one of the inorganic toxin binders that are more effective in binding aflatoxin B1 than others, which is due to the purification of the inorganic clays (EFSA et al., 2017). The surface area and chemical affinities between adsorbent and mycotoxins have been reported to increase the process of binding (Romoser et al., 2013). The latter explains why bentonite had higher aflatoxin reduction activity than the microbes. The performance of yeast was next to that of bentonite, probably because of the similar mode of action, that is, adsorption of the aflatoxins. The lower performance of bacteria is most likely due to the differences in mode of action; the biodegradation effect, where enzyme induction and production needs more time than what was used in this study. The experimental feed contained some aflatoxins because it was impossible to obtain toxin-free ingredients. Removal of the basal aflatoxins in the non-spiked feed sample to undetectable levels by both the bentonite and the microbes; is probably an indication that the efficiency depends on the levels of contamination. However, the decontamination activity at different aflatoxin levels calls for further evaluation. Previous researchers used aflatoxin B1 for the decontamination experiments, while the current study did not purify the aflatoxin by type. Similar reduction activities probably point to presence of the aflatoxin B1 as the major type in the in-house aflatoxin extract used during the current study.
Generally, in-feed aflatoxin decontamination was lower than in vitro activity in PBS for the bacteria; and for the yeast and bacteria combination. This could have been influenced by the substrate, the feed that did not offer a conducive environment, which probably affects the growth and therefore aflatoxin biodegradation process. The current finding contrasts with that by Siahmoshteh et al. (2017) where B. subtilis had 85.66% reduction in aflatoxin B1 toxin in broth culture compared to 95% in the pistachio nuts. The latter was probably because the bacteria can grow luxuriantly in presence of the nuts, as the substrate. Bentonite exhibited higher activity in feed than in PBS and there was no difference in case of yeast, which was probably influenced by the mode of action.
Shetty and Jespersen (2006) reported that live yeast strains and heat-treated preparations were efficient at binding aflatoxin B1 on mixing as feed additives, thus emphasizing that adsorption was not affected by the media where the toxin was suspended. Significant differences on the effect of feed matrix between the test agents were observed, but not on the decontamination activity of each test item, this attribute needs to be evaluated further before availing the product to the industrial processors.
There were no significant differences in the aflatoxin decontamination at various pH. The same trend of reduction of bentonite being the highest, followed by yeast, then a combination of yeast and bacteria; and lastly bacteria, was observed. Bentonite performed better at pH 6.5 than at pH 4.5; which contrasted to the microbial agents’ activity. Yeast, just like other fungi, prefer acidic pH for growth, but the adsorption mode of action, which depends on the cell wall components does and not on cell viability, is probably reason for the observed activity. Similar findings were reported by Gonçalves et al. (2014). Dogi et al. (2011) demonstrated three yeast strains that survived under gastrointestinal conditions and effectively adsorbed aflatoxin B1 at different pH levels, which is similar to the findings of the current study. The bacterial agent was also not affected by pH since Bacillus spp have a wide range of pH at which they survive or grow. It is likely that the enzyme that is involved in biodegradation is resistant to the low pH, hence the comparable activity observed in this study. The 1% decrease in aflatoxin reducing activity at pH 6.5 compared to that at pH 4.5 contrasts Jouany et al. (2005) findings of greatest degradation at pH 5 – 6.5, probably due to differences in the microorganisms analyzed, since the previous researcher evaluated Lactobacillus spp.
The trends of decontamination by the tested microbial agents were similar at the same temperature, except at 42°C where B285 performed better than the others, including bentonite. Alteration of temperatures in the present study did not have any significant effect (p>0.05) on the efficacy of bentonite on aflatoxin reduction; similar to findings by Ramos and Hernandez (1996). However, our findings contrast some earlier reports, where a significant decrease in the adsorbed aflatoxin B1 by bentonite was recorded when the temperature was elevated from 25 to 45°C (Wongtangtintan et al., 2016). The ability of the Bacillus spp to grow at high temperatures could have resulted in increased cell populations and/or their enzymatic activity; hence exhibition of the highest aflatoxin reduction activity was at 42°C. Having been isolated from chicken droppings and given the body temperature of the birds, the Bacillus spp strain B285 was already adapted to such thermal conditions. The current findings agreed with Farzaneh et al. (2012) who previously reported, an optimum temperature for B. subtilis cell free supernatant was 35 to 40°C. Besides, an earlier study indicated that bacterial adsorption of aflatoxins was both temperature and concentration dependent (Haskard et al., 1998). Since yeast and other eukaryotic cells’ optimum temperature for growth is 37°C, lower activity would be expected at higher temperatures. However, the yeast activity was highest at 42°C because non-viable cells can also adsorb the toxins. The high activity at 42°C, pH 6.5 and at pH 4.5 implies that these microbial strains can impart their effect in vivo, since these conditions simulated those of chicken intestinal tract. Indeed, the crop and the proventriculus have a pH of about 4.5 whereas the small and large intestines have a pH of about 6.5 in chickens (Mabelebele et al., 2014).
Identification of potential mycotoxin-detoxifying agents is based on the ability to adsorb more than 80% aflatoxins available in solution; and determination of the mechanisms and conditions favorable for adsorption (Ahlberg et al., 2015; Pereyra et al., 2013). These are considered the minimum for passing the in vivo tests. However, the selected yeast candidate strain should be analyzed further to establish the mechanism of action and the appropriate concentrations for in vivo application in feed.
This study demonstrated the in vitro aflatoxin reducing activity of two selected microbial strains. From the results, it can be concluded that bentonite is more effective in adsorbing aflatoxins from poultry feed compared to their biological counterparts. Yeast strain Y833 was more effective than the bacterial strain B285 (Bacillus spp.) in decreasing the aflatoxin concentration in the poultry feeds. It can also be concluded that alternation of temperatures between 25°C through 37 to 42°C has no significant effect on the aflatoxin reduction capacity of the tested agents. Likewise, changing pH from 4.5 to 6.5 has insignificant influence on the aflatoxin reducing capacity of both biological and commercial chemical/mineral binders. In determining the mode of decontamination, reduction activity in relation to aflatoxin concentration, the duration of exposure and microbial cell population can elucidate the combined microbial activity to obtain conclusive explanations before recommendation for use by the feed processors. In addition, safety studies of the selected microbial candidates need to be performed before in vivo evaluation and large-scale production is embarked upon.
The authors have not declared any conflict of interests.
The authors are thankful to the National Agricultural Research Systems-Competitive Grant Scheme 2013/2014 for funding this work. The laboratory assistance offered by Ritah Nabunje, and Tom Bbosa is greatly appreciated.
REFERENCES
Ahlberg SH, Joutsjoki V, Korhonen HJ (2015). Potential of lactic acid bacteria in aflatoxin risk mitigation. International Journal of Food Microbiology 207:87-102.
Crossref |
|
Assaf JC, Nahle S, Chokr A, Louka N, Atoui A, El Khoury A (2019). Assorted methods for decontamination of aflatoxin M1 in milk using microbial adsorbents. Toxins 11(6):304.
Crossref |
|
Benkerroum N (2020). Chronic and Acute Toxicities of Aflatoxins: Mechanisms of Action. International Journal of Environmental Research and Public Health 17(2):423.
Crossref |
|
Boudergue C, Burel C, Dragacci S, Favrot MC, Fremy JM, Massimi C, Prigent P, Debongnie P, Pussemier L, Boudra H (2009). Review of mycotoxinâ€detoxifying agents used as feed additives: mode of action, efficacy and feed/food safety. EFSA Supporting Publications 6(9):22E.
Crossref |
|
Chlebicz A, Śliżewska K (2020). In vitro detoxification of aflatoxin B1, deoxynivalenol, fumonisins, T-2 Toxin and zearalenone by probiotic bacteria from genus lactobacillus and saccharomyces cerevisiae yeast. Probiotics and Antimicrobial Proteins 12(1):289-301.
Crossref |
|
Coppock RW, Christian RG, Jacobsen BJ (2018). Veterinary toxicology, basic and clinical principles. Aflatoxins. RC Gupta, ed. 3rd ed. Elsevier, Amsterdam, The Netherlands pp. 983-993.
Crossref |
|
Dogi C, Armando R, Ludueña R, De Moreno de LeBlanc A, Rosa C, Dalcero A, Cavaglieri L (2011). Saccharomyces cerevisiae strains retain their viability and aflatoxin B1 binding ability under gastrointestinal conditions and improve ruminal fermentation. Food Additives and Contaminants: Part A 28(12):1705-1711.
Crossref |
|
EFSA Panel on Additives and Products or Substances used in Animal Feed (FEEDAP), Rychen, G, Aquilina G, Azimonti G, Bampidis V, Bastos MDL, Bories G, Chesson A, Cocconcelli PS, Flachowsky G. and Group J (2017). Safety and efficacy of bentonite as a feed additive for all animal species. EFSA Journal 15(12):e05096.
Crossref |
|
FAO, IFAD, UNICEF, WFP and WHO (2019). The State of Food Security and Nutrition in the World 2019. Safeguarding against economic slowdowns and downturns. Rome, FAO.
Crossref |
|
Farzaneh M, Shi ZQ, Ghassempour A, Sedaghat N, Ahmadzadeh M, Mirabolfathy M, Javan-Nikkhah M (2012). Aflatoxin B1 degradation by Bacillus subtilis UTBSP1 isolated from pistachio nuts of Iran. Food Control 23(1):100-106.
Crossref |
|
Gan F, Hang X, Huang Q, Deng Y (2019). Assessing and modifying China bentonites for aflatoxin adsorption. Applied Clay Science 168:348-354.
Crossref |
|
Gonçalves BL, Rosim RE, Oliveira CA Fd, Corassin CH (2014). Efficiency of different sources of Saccharomyces cerevisiae to bind aflatoxin B1 in phosphate buffer saline. Food Process Technology 5:342.
Crossref |
|
Grace D, Lindahl JF, Atherstone C, Kang'ethe EK, Nelson F, Wesonga T, Manyong VM (2015). Building an Aflatoxin Safe East African Community. Aflatoxin standards for Feed Knowledge Platform. Situational Analysis for East Africa Region. Technical Policy Paper 7. |
|
Gul H, Khan S, Shah Z, Ahmad S, Israr M, Hussain M (2017). Effects of local sodium bentonite as aflatoxin binder and its effects on production performance of laying hens. Kafkas Üniversitesi Veteriner Fakültesi Dergisi 23(1):31-37. |
|
Haskard C, El Nezami H, Peltonen K, Salminen S, Ahokas J (1998). Sequestration of aflatoxin B1 by probiotic strains: binding capacity and localisation. Revue de Medecine Veterinaire (France) 149:571. |
|
Ismail A, Gonçalves BL, de Neeff DV, Ponzilacqua B, Coppa CF, Hintzsche H, Sajid M, Cruz AG, Corassin CH, Oliveira CA (2018). Aflatoxin in foodstuffs: Occurrence and recent advances in decontamination. Food Research International 113:74-85.
Crossref |
|
Jouany J, Yiannikouris A, Bertin G (2005). The chemical bonds between mycotoxins and cell wall components of Saccharomyces cerevisiae have been identified. Archiva Zootechnica 8:26-50. |
|
Kana J, Gnonlonfin B, Harvey J, Wainaina J, Wanjuki I, Skilton R, Teguia A (2013). Assessment of aflatoxin contamination of maize, peanut meal and poultry feed mixtures from different agroecological zones in Cameroon. Toxins 5(5):884-894.
Crossref |
|
Karaman M, Basmacioglu H, Ortatatli M, Oguz H (2005). Evaluation of the detoxifying effect of yeast glucomannan on aflatoxicosis in broilers as assessed by gross examination and histopathology. British Poultry Science 46(3):394-400.
Crossref |
|
Khaneghah AM, Fakhri Y, Sant'Ana AS (2018), Impact of unit operations during processing of cereal-based products on the levels of deoxynivalenol, total aflatoxin, ochratoxin A, and zearalenone: a systematic review and meta-analysis. Food Chemistry 268:611-624.
Crossref |
|
Kim S, Lee H, Lee S, Lee J, Ha J, Choi Y, Yoon Y, Choi KH (2017). Invited review: Microbe-mediated aflatoxin decontamination of dairy products and feeds. Journal of Dairy Science 100(2):871-880.
Crossref |
|
Li J, Huang J, Jin Y, Wu C, Shen D, Zhang S, Zhou R (2018). Mechanism and kinetics of degrading aflatoxin B1 by salt tolerant Candida versatilis CGMCC 3790. Journal of Hazardous Materials 359:382-387.
Crossref |
|
Lubna MA, Debnath M, Hossaini F (2018). Detection of Aflatoxin in Poultry Feed and Feed Materials through Immuno Based Assay from Different Poultry Farms and Feed Factories in Bangladesh. Bangladesh Journal of Microbiology 35(1):75-78.
Crossref |
|
Lukwago FB, Mukisa IM, Atukwase A, Kaaya AN, Tumwebaze S (2019). Mycotoxins contamination in foods consumed in Uganda: a 12- year review (2006-2018). Scientific African 3:e00054.
Crossref |
|
Mabelebele M, Alabi O, Ngambi J, Norris D, Ginindza M (2014). Comparison of gastrointestinal tracts and pH values of digestive organs of Ross 308 broiler and indigenous Venda chickens fed the same diet. Asian Journal of Animal and Veterinary Advances 9:71-76.
Crossref |
|
Magnoli AP, Tallone L, Rosa CA, Dalcero AM, Chiacchiera SM, Sanchez RMT (2008). Commercial bentonites as detoxifier of broiler feed contaminated with aflatoxin. Applied Clay Science 40(1-4):63-71.
Crossref |
|
Manafi M, Narayanaswamy H, Pirany N (2009). In vitro binding ability of mycotoxin binder in commercial broiler feed. African Journal of Agricultural Research 4(2):141-143. |
|
Mgbeahuruike AC, Ejioffor TE, Christian OC, Shoyinka VC, Karlsson M, Nordkvist E (2018). Detoxification of aflatoxin-contaminated poultry feeds by 3 adsorbents, bentonite, activated charcoal, and fuller's earth. The Journal of Applied Poultry Research 27(4):461-471.
Crossref |
|
Mukandungutse B, Tuitoek J, King'ori A, Obonyo M (2019). The effect of fermentation with and without Saccharomyces cerevisiae on the levels of aflatoxin in maize. Livestock Research for Rural Development 31(11). |
|
Mwakinyali SE, Ding X, Ming Z, Tong W, Zhang Q, Li P (2019). Recent development of aflatoxin contamination biocontrol in agricultural products. Biological Control 128:31-39.
Crossref |
|
Nakavuma JL, Kirabo A, Bogere P, Nabulime MM, Kaaya AN, Gnonlonfin B (2020). Awareness of mycotoxins and occurrence of aflatoxins in poultry feeds and feed ingredients in selected regions of Uganda. International Journal of Food Contamination 7(1):1-10.
Crossref |
|
Omara T, Nassazi W, Omute T, Awath A, Laker F, Kalukusu R, Musau B, Nakabuye BV, Kagoya S, Otim G (2020). Aflatoxins in Uganda: An Encyclopedic Review of the Etiology, Epidemiology, Detection, Quantification, Exposure Assessment, Reduction, and Control. International Journal of Microbiology.
Crossref |
|
Osuret J, Musinguzi G, Mukama T, Halage AA, Natigo AK, Ssempebwa JC, Wang JS (2016). Aflatoxin contamination of selected staple foods sold for human consumption in Kampala markets, Uganda. Journal of Biological Sciences 16(1):44-48.
Crossref |
|
Pankaj S, Shi H, Keener KM (2018). A review of novel physical and chemical decontamination technologies for aflatoxin in food. Trends in Food Science and Technology 71:73-83.
Crossref |
|
Pereyra CM, Cavaglieri, LR, Chiacchiera SM, Dalcero A (2013). The corn influence on the adsorption levels of aflatoxin B 1 and zearalenone by yeast cell wall. Journal of Applied Microbiology 114(3):655-662.
Crossref |
|
Petchkongkaew A, Taillandier P, Gasaluck P, Lebrihi A (2008). Isolation of Bacillus spp. from Thai fermented soybean (Thuaâ€nao): screening for aflatoxin B1 and ochratoxin A detoxification. Journal of Applied Microbiology 104(5):1495-1502.
Crossref |
|
Rajarajan P, Rajasekaran K, Asha Devi N (2013). Isolation and quantification of aflatoxin from Aspergillus flavus infected stored peanuts. Indian Journal of Pharmaceutical and Biological Research 1:76-80.
Crossref |
|
Ramos A, Hernandez E (1996). In vitro aflatoxin adsorption by means of a montmorillonite silicate. A study of adsorption isotherms. Animal Feed Science and Technology 62(2-4):263-269.
Crossref |
|
Romoser AA, Marroquin-Cardona A, Phillips TD (2013). Managing risks associated with feeding aflatoxin contaminated feed. Paper presented at the Proceedings of the 22nd Tri-State Dairy Nutrition Conference, Fort Wayne, Indiana, USA, 23-24 April 2013. |
|
Shetty PH, Jespersen L (2006). Saccharomyces cerevisiae and lactic acid bacteria as potential mycotoxin decontaminating agents. Trends in Food Science and Technology 17(2):48-55.
Crossref |
|
Shotwell OL, Hesseltine C, Stubblefield R, Sorenson W (1966). Production of aflatoxin on rice. Applied and Environmental Microbiology 14(3):425-428.
Crossref |
|
Siahmoshteh F, Siciliano I, Banani H, Hamidi-Esfahani Z, Razzaghi-Abyaneh M, Gullino ML, Spadaro D (2017). Efficacy of Bacillus subtilis and Bacillus amyloliquefaciens in the control of Aspergillus parasiticus growth and aflatoxins production on pistachio. International Journal of Food Microbiology 254:47-53.
Crossref |
|
Vekiru E, Fruhauf S, Rodrigues I, Ottner F, Krska R, Schatzmayr G, Ledoux DR, Rottinghaus GE, Bermudez AJ (2015). In vitro binding assessment and in vivo efficacy of several adsorbents against aflatoxin B1. World Mycotoxin Journal 8(4):477-488.
Crossref |
|
Wang Y, Zhang H, Yan H, Yin C, Liu Y, Xu Q, Liu X, Zhang Z (2018). Effective biodegradation of aflatoxin B1 using the Bacillus licheniformis (BL010) strain. Toxins 10(12):497-513.
Crossref |
|
Wang M, Hearon SE, Phillips TD (2020). A high capacity bentonite clay for the sorption of aflatoxins. Food Additives and Contaminants: Part A 37(2):332-341.
Crossref |
|
Womack ED, Brown AE, Sparks DL (2014). A recent review of nonâ€biological remediation of aflatoxinâ€contaminated crops. Journal of the Science of Food and Agriculture 94(9):1706-1714.
Crossref |
|
Wongtangtintan S, Neeratanaphan L, Ruchuwararak P, Suksangawong S, Tengjaroenkul U, Sukon P, Tengjaroenkul B (2016). Comparative study of aflatoxin B1 adsorption by Thai bentonite and commercial toxin binders at different temperatures in vitro. Livestock Research for Rural Development 28(4). |