ABSTRACT
The indigenous Tswana pig is currently listed as an endangered animal genetic resource and if not conserved, might go extinct. The objective of this study was to assess the genetic diversity (genetic characterization) of the indigenous Tswana pig population. Blood samples were collected from 30 randomly selected Tswana pigs in Kgatleng and South-East districts of Botswana for the assessment of genetic diversity using a panel of 12 FAO-recommended microsatellite markers. All the microsatellite markers screened in indigenous Tswana pigs were polymorphic and the number of observed alleles per marker varied between 3 (SW2406) and 9 (SW225) with mean number of alleles per marker of 6.33. The observed heterozygosity ranged from 0.16 (SW2405) to 0.875 (SW2465) with average observed heterozygosity across all 12 loci of 0.647. The expected heterozygosity was lower than the observed heterozygosity and ranged between 0.143 (SW2405) and 0.776 (SO385) with mean expected heterozygosity across all loci of 0.603. The allelic diversity and levels of heterozygosity indicate high levels of genetic diversity in Tswana pig population. The within-locus inbreeding coefficient (Fis) ranged between -0.321(S0120) and 0.234 (SW35) with inbreeding coefficient of the entire population of -0.012 indicating that the Tswana pig population is relatively outbred.
Key words: Genetic diversity, microsatellite markers, heterozygosity
Indigenous pigs are kept by the rural populace under the low-output free range production system. Indigenous Tswana pigs are mostly owned by women, usually survive in harsh, low input environments and strive under high disease, parasite prevalence and nutrients shortages (Chabo et al., 2000). During the 1980’s, indigenous Tswana pigs were found in South East, Kgatleng and Kweneng districts of Botswana while nowadays they are fairly well distributed in the south east district of the country in and around Ramotswa village (Nsoso et al., 2006). The farmers who keep indigenous pigs in Botswana have a tendency to keep low numbers to match herd size with available feed resources. Notable attributes of indigenous Tswana pigs include disease resistance, high fertility, parasite and heat tolerance, low protein requirements, ability to utilize course fibrous rations and strong feet which make them suitable for free range low-intensity management systems affordable to the rural poor (Gandin and Oldenbroek, 1999; Lekule and Kyvsgaard, 2003).
The indigenous Tswana pigs are usually black or black with white stripes (Figure 1) and have a body of medium stature (Nsoso et al., 2006). Indigenous Tswana pigs are however shunned away from commercial production systems due to their inferior growth and reproductive performance and carcass traits relative to exotic breeds (Moreki and Montsho, 2012). Resource-poor farmers in rural areas also view genetic improvement of indigenous Tswana pigs as synonymous to crossbreeding, grading up and possible breed replacement with exotic breeds (Nsoso et al., 2004).
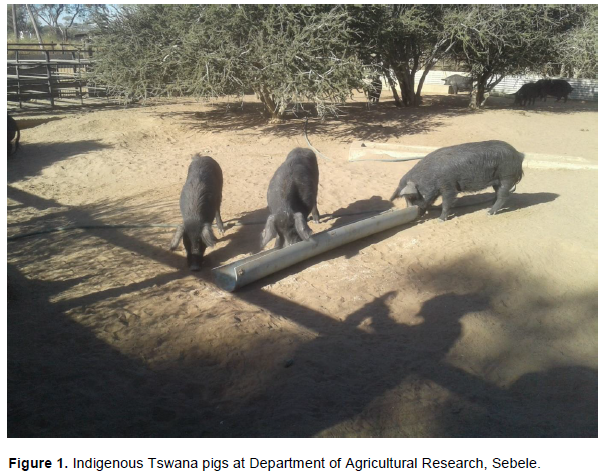
Extensive system coupled with undeveloped markets for indigenous Tswana pigs and lack of a clear policy on the conservation of indigenous animal genetic resources in the country is leading to the disappearance of indigenous Tswana pigs. This poses a risk of worsening poverty levels for most of the rural women populace who own most of the indigenous Tswana pigs since the fast-growing exotic pigs require high levels of inputs and management unaffordable to the resource-poor and highly marginalized farmers. The population of indigenous Tswana pigs has declined drastically in the last three decades and the indigenous Tswana pig is currently listed as an endangered animal genetic resource (Podisi, 2001). Rege and Lipner (1992) argued that some indigenous animal genetic resources of Africa are endangered and may even be lost before they are described and documented, and the indigenous Tswana pig is one classic example. Research to evaluate the indigenous Tswana pig has been sporadic and inadequate; consequently, the indigenous Tswana pig has not been sufficiently characterized. Information on phenotypic characteristics and production performance of Tswana pigs is still very scarce and there has been no attempt till date aimed at their genetic characterization. Genetic characterization of Tswana pigs by microsatellite markers is important to assess the degree of genetic diversity in the remaining population, the extent of inbreeding and will inform future conservation and management practices. The objective of this study was therefore to assess the genetic diversity of the indigenous Tswana pig population using microsatellite markers.
Population sampling
Blood samples were collected from 30 unrelated Tswana pigs in the Southern half of the country in Kgatleng and South-East districts following the guidelines of Measurement of Domestic Animal Diversity FAO (2011) programme. Blood samples were collected from the ear vein of the animals in vacutainer tubes containing EDTA as the anticoagulant. Blood samples were then transported to the laboratory at 0-4°C (under ice cubes) and stored overnight at -20°C prior to DNA extraction. Information on sampling locations and number of samples per sampling location is given in Table 1.
DNA extraction
Genomic DNA was isolated from whole blood using Zymo Quick-gDNA miniPrep kit following the manufacturer’s protocol. Briefly, 400 µl of Genomic Lysis Buffer was added to 100 µl of whole blood and completely mixed by vortexing for 4-6 s. The mixture was allowed to stand for 5-10 min at room temperature, transferred to a Zymo-SpinTM Column in Collection Tube and centrifuged at 10,000 ×g for a minute. The collection tube with the flow through was discarded and the Zymo-SpinTM Column transferred into a new collection tube. 200 µl of DNA Pre-Wash Buffer was added to the spin column and centrifuged at 10,000 ×g for a minute. 500 µl of g-DNA Wash Buffer was added to the spin column and centrifuged at 10,000 ×g for a minute. The spin column was then transferred to clean micro centrifuge tube and 60 µl of DNA Elution Buffer was added and incubated 2-5 min at room temperature. The spin column was then centrifuged at top speed for 30 s to elude the gDNA. The concentration of eluded gDNA was measured using a spectrophotometer (Nanodrop, 2000) and the purity of the gDNA was verified by the 260/280 absorbance ratio (Thermo Fisher Scientific Inc., Waltham, MA, USA).
Microsatellite markers amplification and analysis
A panel of 12 microsatellites recommended by Food Agriculture Organisation (FAO)/ISAG-FAO Advisory Group on Animal Genetic Diversity FAO (1995) were used for genetic characterization of Tswana pigs. The markers used in the study (with chromosome position) were: SW2456 (X/Y), S0165 (3), SW225 (13), SW2008 (11), SW35 (4), SW2406 (6), S0385 (11), S0120 (18), S0073 (4), SW2443 (2), SW949 (X/Y), and SW2410 (17). Selective amplification of different microsatellites was achieved by polymerase chain reaction using the thermocycler GeneAmp PCR system 9700 (Applied Bio systems, Forster City CA, USA) and PCR reagents synthesized by Fermentas Life Sciences Opelstrasse, Germany. Each 25 µl PCR reaction comprised approximately 100 ng gDNA, primers (60 ng each), dNTPs (40 mm each), 10X ammonia-based PCR buffer (2.5 µL), 1.5 mm MgCl2, 1 unit of Taq DNA polymerase and PCR grade deionized water. The PCR reaction was accomplished by initial denaturation for 5 min at 94°C, followed by 33 cycles of denaturation at 94°C for 30 s, primer annealing for 45 s at the desired temperature and DNA replication at 72°C for 1 min. The final extension step was run at 72°C for 10 min. The resulting PCR products were denatured at 98ºC for 3 min and rapidly cooled by placing on ice. The PCR products were separated by capillary electrophoresis on ABI Prism 310 Genetic Analyzer (Applied Biosystems, Foster city, CA, USA) according to the manufacturers recommendations and allele sizing was achieved by using the internal size standard of Genescan-500 LIZ (Applied Biosystems, Foster city, CA, USA). Data on allele sizes was done using Genescan Analysis software v.3.1.2 and the identification of different alleles for each marker was performed by Genotyper 2.5 software.
Statistical analysis
The within breed genetic diversity parameters for Tswana pigs which included observed heterozygosity (Ho), expected heterozygosity (He), polymorphism information content (PIC) and mean number of alleles (MNA) were calculated using Microsatellite Toolkit software (Kim et al., 2005). The inbreeding coefficient (Fis) for each locus was computed using the program FSTAT (Goudet, 2001). The probability test approach (Gou and Thompson, 1992) implemented in the GENEPOP software (Gou and Thompson, 1992) was used to test each locus for Hardy-Weinberg equilibrium.
All the microsatellite markers screened in indigenous Tswana pigs were polymorphic (Table 2). A total of 76 alleles were detected in 12 microsatellite markers screened and the allele size range varied from 83-107 bp at locus S0073 to 220-234 bp at marker locus SW2406. The number of observed alleles per marker varied between 3 (SW2406) and 9 (SW225) with mean number of alleles per marker of 6.33 (Table 2). The range of observed number of alleles per marker and mean number of alleles per marker observed in this study are comparable to 3.38-8.71 and 6.25, respectively, found in local Criollo pig breeds from the Americas (Revidatti et al., 2014) but lower than the range of 5-12 alleles per marker and mean number of alleles per marker of 7.04 reported in indigenous Andaman Desi pig of India (De et al., 2013) and mean number of alleles per marker of 8.45 found in indigenous pigs of Mozambique (Swart et al., 2010). The range of observed number of alleles per marker found in this study is however, higher than the range of 3.98- 5.54 reported by Swart et al. (2010) in commercial pig breeds of South Africa (Landrace, large white and Duroc).
The mean number of alleles per marker of 6.33 found in this study is comparable to 6.18 found in indigenous South African Kolbroek breed (Swart et al., 2010) but higher than 5.72 found in Uruguayan Pampa Rocha pigs (Montenegro et al., 2015), 3.93 and 5.97 in Namibia and Kune-kune breeds (Swart et al., 2010). Effective number of alleles in Tswana pigs ranged between 1.11 (SW2406) and 5.01 (S0165) with mean effective number of alleles per marker of 3.31±1.18. Revidatti et al. (2014) reported a lower mean effective number of alleles per marker of 3.33±1.56 in Criolli pig breeds of the Americas which is comparable with the present study. The mean effective number of alleles per marker in Tswana pigs is however lower than the mean effective number of alleles per marker of 5.09±0.20 found in Andaman Desi pigs of India. According to Pandey et al. (2006), FAO specified a minimum of four alleles per marker for effective screening of genetic differences between breeds and all the markers used in this study with the exception of SW2406 exhibited sufficient polymorphism for evaluation of genetic variation within breed and genetic differences between breeds.
Apart from the number of alleles per locus and mean number of alleles for all loci, other measures of genetic diversity include observed heterozygosity, expected heterozygosity and polymorphic information content (PIC) and those are depicted in Table 3.
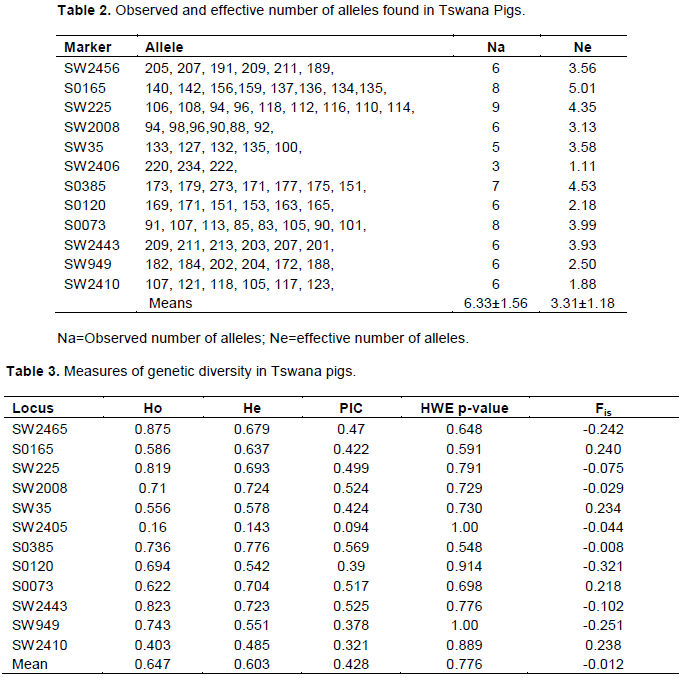
The observed heterozygosity for individual markers ranged from 0.16 (SW2405) to 0.875 (SW2465) with average observed heterozygosity across all 12 loci of 0.647. The expected heterozygosity was lower than the observed heterozygosity and ranged from 0.143 (SW2405) to 0.776 (S0385) with mean expected heterozygosity across all loci of 0.603. For markers to be useful in measuring genetic variation they should have average heterozygosity between 0.3 and 0.8 (Takezaki and Nei, 1996) and therefore all the markers used in this study with the exception of SW2405 were appropriate for measuring genetic variation in Tswana pigs. According to Nei and Kumar (2000), observed heterozygosity and expected heterozygosity are highly correlated but expected heterozygosity also known as Hardy-Weinberg heterozygosity is considered a better estimator of the genetic variability present in a population.
More heterozygous loci than expected in Tswana pigs is consistent with Setyawan et al. (2015) who observed a similar pattern in most Indonesian Native cattle breeds. Unlike in Tswana pigs, most pig genetic characterization studies report heterozygote deficiencies than heterozygote excesses (De et al., 2013) due to inbreeding resulting from limited population sizes and selective breeding in pig improvement programs. The average expected heterozygosity of 0.603 and observed heterozygosity of 0.647 indicate high level of genetic variability or genetic diversity in Tswana pigs since it is interpreted as such when the heterozygosity values exceed 0.5 (Melendez et al., 2014). High degrees of genetic diversity have also been reported in indigenous pigs of Cerete-Colombia, Andaman Desi pig, Criollo pig breeds from the Americas, Uruguayan Pampa Rocha pigs and Chinese village pigs with expected heterozygosity values of 0.527, 0.77, 0.622, 0.603 and 0.826, respectively (Melendez et al., 2014; De et al., 2013; Revidatti et al., 2014; Montenegro et al., 2015; Fang et al., 2009). The expected heterozygosity value of 0.603 found in Tswana pigs is comparable to those found in other Southern African pig breeds such as Mozambique indigenous pig, South African Kolbroek and South African Kune-Kune with Hardy-Weinberg heterozygosity values of 0.692, 0.634 and 0.675, respectively (Swart et al., 2010). Compared to commercial pig breeds, the average expected heterozygosity of the indigenous Tswana (0.603) is similar to 0.60 of the large white (Oh et al., 2014) but slightly higher than 0.580 and 0.531 of the South African Landrace and Duroc breeds, respectively (Swart et al., 2010). The high level of genetic variation or diversity in Tswana pigs might be attributed to lack of selective breeding or improvement programs targeted towards the breed and possible existence of population substructure (Genetic uniqueness in terms of alleles of Tswana pigs coming from different villages).
The polymorphic information content (PIC) values of the 12 markers employed in the characterization of Tswana pigs ranged from 0.094 for SW2405 to 0.569 for S0385 with average PIC value of all the markers of 0.428 (Table 3). According to Montenegro et al. (2015), markers with PIC values greater than 0.5 are highly informative, those with PIC values between 0.25 and 0.5 are moderately informative and those with PIC values less than 0.25 are uninformative. Following the same classification criterion, four markers (SW2008, S0385, S0073 and SW2443) were highly informative, seven (SW2465, S0165, SW225, SW35, S0120, SW949 and SW2410) were moderately informative and one (SW2405) was uninformative in Tswana pigs. Moderately informative and highly informative markers are more variable and therefore more suitable for genetic diversity studies in indigenous Tswana pigs.
All the 12 microsatellite markers used in the current study were in Hardy-Weinberg Equilibrium clearly indicating the high genetic stability of indigenous Tswana pigs kept by farmers under extensive management system. The high genetic stability of indigenous Tswana pigs confirm that Tswana pigs are mostly random mating under free running management system practised by majority of farmers, is not undergoing any artificial selection (no improvement program for indigenous Tswana pig), the effects of random genetic drift common in small populations like that of Tswana pigs are negligible and Tswana pigs are not subjected to other evolutionary forces such as mutation and migration capable of altering gene, genotype frequencies and causing significant departures form Hardy-Weinberg equilibrium.
The within-locus inbreeding coefficient (Fis) ranged between -0.321(S0120) and 0.234 (SW35) with multilocus inbreeding coefficient of the entire population of -0.012. The negative inbreeding coefficient of Tswana pigs might be due to avoidance of mating among closely related animals (Hui-Fang et al., 2010) which resulted in significant excess of heterozygotes in the population. All the markers with the exception of SW35 and S0073 contributed to the negative inbreeding coefficient of the Tswana pigs. Markers SW35 and S0073 exhibited significant deficit of heterozygotes probably due to genetic drift or linkage disequilibrium of the marker with loci under either natural or artificial selection (Ibeagha and Erhardt, 2005).
Moderate levels of genetic diversity and no inbreeding exist within the Tswana pig population in Southern Botswana. This genetic diversity in the Tswana pigs showed that there is random mating and the animals are not undergoing any form of artificial selection. If deliberate efforts towards conservation are not put in place, this valuable genetic resource with its hardiness, disease resistance and heat tolerance genes might become extinct within the next decades, even before it has been fully characterized. The conservation of indigenous Tswana pigs should be given high priority because it contains valuable genes (disease resistance and heat tolerance genes) for future breed developments and genetic engineering applications to counter the effects of global warming or climate change on pig production and productivity.
The authors have not declared any conflict of interests.
The authors thank Botswana University of Agriculture and Natural Resources for funding the study and Agricultural Research Council-Animal Production Institute (ARC-API) for availing their laboratory (equipment and reagents) for microsatellite marker amplification and analysis and for further assisting with data analysis using their various molecular software’.
REFERENCES
Chabo RG, Malope P, Babusi B (2000). Pig Productivity; A case study for South Eastern Botswana. Livestock Research for Rural Development 12:3.
Crossref
|
|
De AK, Jeyakumar S, Kundu A, Kundu MS, Sunder J, Ramachandran M (2013). Genetic characterization of Andaman Desi pig, an indigenous pig germplasm of Andaman and Nicobar group of islands, India by microsatellite markers. Veterinary World 6(10):750-753.
Crossref
|
|
|
Fang M, Hu X, Jin W, Li N, Wu C (2009). Genetic uniqueness of Chinese village pig population inferred from microsatellite markers. Journal of Animal Science 87:3445-3450.
Crossref
|
|
|
FAO (1995). Measurement of Domestic Animal Diversity. Draft Project Formulation Report. Rome. Italy.
|
|
|
FAO (2011). Molecular genetic characterisation of animal genetic resources. FAO Animal Production and Guidelines No. 9. Rome
|
|
|
Gandin GC, Oldenbroek JK (1999). Gene bank and the management of farm animal genetic resources. DLO Institute for Animal Science and Health. 8200 AB Lelystad, Netherlands.
|
|
|
Goudet J (2001). F-STAT A computer programme to calculate F-statistics. University of Lausanne. Version 1.2.
|
|
|
Gou SW, Thompson EA (1992). Performing the exact test of Hardy-Weinberg Proportions of multiple alleles. International Biometry Society 4(2):361-372.
Crossref
|
|
|
Hui-Fang L, Wei-Tao S, Jing-Ting S, Kuan-Wei C, Wen-Qi Z, Wei H, Wen-Juan X (2010). Genetic diversity and population structure of 10 Chinese indigenous egg-type duck breeds assessed by microsatellite polymorphism. Journal of Genetics 89:65-72.
Crossref
|
|
|
Ibeagha EM, Erhardt G (2005). An evaluation of genetic diversity indices of the Red Bororo and White Fulani cattle breeds with different molecular markers and their implications for current and future improvement options. Tropical Animal Health and Production 38(5):431-441.
Crossref
|
|
|
Kim TH, Kim KS, Khoi BH, Yoon DH, Jang GW, Lee KT, Chung HY, Lee HY, Park HS, Lee JW (2005). Genetic structure of pig breeds from Korea and China using microsatellite loci analysis. Journal of Animal Science 83:2255-2263.
Crossref
|
|
|
Lekule FP, Kyvsgaard NC (2003). Improving pig husbandry in tropical resource poor communities and its potential to reduce the risk of porcine cysticercosis. Acta Tropica 87L:111-117.
Crossref
|
|
|
Melendez Ivan G, Enrique Pardo P, Teodora Cavadia M (2014). Genetic characterization of the domestic pig (Susscrofa domestica) in Cerete-Colombia, using microsatellite markers. Revista MVZ Cordoba 19(2):4150-4157.
Crossref
|
|
|
Montenegro M, Llambi S, Castro G, Barlocco N, Vadell A, Landi V, Delgado JV, Martínez A (2015). Genetic characterization of Uruguayan Pampa Rocha pigs with microsatellite markers. Genetics and Molecular Biology 38(1):48-54.
Crossref
|
|
|
Moreki JC, Montsho T (2012). Challenges in commercial pig production in Botswana. Journal of Agricultural Technology 8(4):1161-1170.
|
|
|
Nei M, Kumar S (2000). Molecular Evolution and Phylogenetic. Oxford University Press, New York.
|
|
|
Nsoso SJ, Mosweu S, Malela L, Podisi B (2004). A survey on Population, distribution, management and utilization of indigenous Tswana Pigs in Southern Botswana. Animal Genetic Resources Information 34:85-96.
Crossref
|
|
|
Nsoso SJ, Mannathoko GG, Modise K (2006). Monitoring production, health and marketing of indigenous Tswana pigs in Ramotswa village of Botswana. Livestock Research for Rural Development 18(9):1-12.
|
|
|
Oh JD, Chacho RGC, Choi JY, Seo JH, Song K, Vega RSA, Santiago RC, Octura JER, Kim SW, Kim CW, Kim SH, Kim SH, Kong HS, Lee HK, Cho BW (2014). Genetic Analysis of Philippine Native Pigs (Susscrofa L.) Using Microsatellite Loci. Philippine Journal of Science 143(1):87-94.
|
|
|
Pandey AK, Sharma R, Singh Y, Prakash BB, Ahlawat SPS (2006). Genetic diversity studies of Kherigarh cattle based on microsatellite markers. Journal of Genetics 85(2):117-122.
Crossref
|
|
|
Podisi B (2001). Management of farm animal resources in Botswana. In Lebie SHB, Kamau L (Editors). Proceedings of planning and priority setting. Workshop on animal genetic resources in SADC region held in Gaborone, Botswana, 2001:19-22 February.
|
|
|
Rege JE, Lipner ME (1992). African animal genetic resources: their characterization, utilization and conservation. ILCA, Addis Ababa, Ethiopia.
|
|
|
Revidatti MA, Delgado Bermejo JV, Gama LT, Landi Periati V, Ginja C, Alvarez LA, Vega-Pla JL, Martínez AM (2014). Genetic characterization of local Criollo pig breeds from Americas using microsatellite markers. Journal of Animal Science 92:4823-4832.
Crossref
|
|
|
Setyawan AD, Lymbery AJ (2015). Genetic Diversity of Five Indonesian Native Cattle Breeds at Microsatellite Loci. Journal of Animal Science 9:57-64.
Crossref
|
|
|
Swart H, Kotze A, Olivier PAS, Grobler JP (2010). Microsatellite-based characterization of Southern African domestic pigs (Sus scrofa domestica). The South African Journal of Animal Science 40(2):121-132.
Crossref
|
|
|
Takezaki N, Nei M (1996). Genetic distances and reconstruction of polygenetic tree from microsatellite DNA. Genetics 144:389-399.
|
|