ABSTRACT
Cassava (Manihot esculenta Crantz) is a tropical root crop that serves as a food staple and a vital income source to resource deprived farmers in the tropics. Despite its importance, cassava production and consumption is faced by a myriad of biotic/abiotic constraints. Genetic transformation which can be applied to mitigate these challenges however has as a prerequisite the availability of robust regeneration systems. This study evaluated the effect of explant type and hormone regime on somatic embryogenesis and regeneration of Kenyan cassava cultivars. The embryogenic competence of immature leaf lobe and stem explants of three cassava cultivars was determined by culturing them in MS medium supplemented with picloram (4, 6, 8 and 10 mg/l). The optimum media composition for embryo maturation, germination and plant recovery was assessed by culturing embryos in media supplemented with varying concentrations of 6-Benzylaminopurine (BAP), α–Naphthalene acetic acid (NAA) and Gibberellic acid (GA3). Somatic embryo formation frequencies in leaf explants were significantly higher (P≤0.05) than in stem explants. Embryo formation rates were found to increase with increasing concentrations of picloram. Cultivar 08/080 gave the least response to embryogenesis while embryogenesis rates for cultivar 08/274 were similar to the control cultivar TMS 60444. Maturation and plant recovery rates differed significantly with regard to the ratios of BAP, NAA and GA3 in the maturation media. The optimal medium for embryo germination and plant recovery was supplemented with 1 mg/l BAP, 0.02 mg/l NAA and 1.5 mg/l GA3. This optimized regeneration protocol can be coupled with mutation breeding or genetic transformation to improve the cassava germplasm.
Key words: Cassava, explant, regeneration, somatic embryogenesis, plant growth regulator.
Cassava (Manihot esculenta Crantz, family Euphorbiaceae) is an important food security crop and a feasible feedstock for numerous industrial processes. In addition to use as food, cassava tubers are important for use as animal feed, for manufacturing industrial products such as an adhesive for laundry purposes, for manufacturing paper, biodegradable materials, beverages and pharmaceutical products (Anyanwu et al., 2015). Due to its increasing consumption in the starch industry and its potential use for biofuel production, the global demand for cassava is growing rapidly (Jansson et al., 2009, FAOSTAT, 2017). The main value of this tuber crop resides in its efficient carbohydrate production, ability to remain in the soil for long without decaying, and the capability of growing in marginal conditions (Alves, 2002). Though maximum yields are gotten under humid tropical conditions on loamy sandy soil, cassava can tolerate marginal/eroded soils and it easily adapts to poor acidic soils that are predominant in the tropics. Additionally, cassava has developed natural barriers against herbivores and arthropods (FAO Joint, 2018).
Cassava is clonally propagated using stem cuttings. Clonal propagation facilitates free exchange of planting material among farmers but is faced by a myriad of challenges such as susceptibility to pests and diseases, accumulation of cyanogens, post-harvest physiological deterioration and a low commercial quality and nutritional value of the starch (Piero et al., 2015). Macro-propagation is constrained by the accumulation of viral and bacterial diseases, unavailability or limited access to planting materials and low multiplication rates which reduce crop productivity. Improvement of the cassava germplasm by traditional breeding methods has been hampered by the non-availability of necessary genes in the germplasm, high heterozygosity, allopolyploidy, low fertility as well as unsynchronized flowering of cassava making it difficult and time-consuming. Genetic transformation techniques that can be applied to complement conventional breeding are hindered by the requirement of a reproducible transformation and regeneration system. In cassava, somatic embryogenesis is the most frequently used regeneration system for the recovery of transgenic events (Chavarriaga-Aguirre et al., 2016). Though efficient, responses to somatic embryogenesis greatly vary among genotypes, and not all cassava varieties are amenable to this morphogenesis process (Marius et al., 2018). Somatic embryogenesis is also affected by explant type and the composition of the culture medium among other factors (Shen et al., 2015). This therefore necessitates optimization of the different regeneration parameters to suit each cultivar of interest.
The morphogenic response of plants in vitro is highly depended on the balance between auxin and cytokinin hormones in the media (Schaller et al., 2015). Somatic embryogenesis and in vitro regeneration can therefore be improved by identifying an optimum hormonal balance for regeneration media (Wongtiem et al., 2011). This study determined the effect of genotype, explant type and hormone regime on somatic embryogenesis and shoot regeneration of selected Kenyan cassava cultivars. We found out that the tested cassava cultivars were amenable to regeneration via somatic embryogenesis though the rates varied among the cultivars.
Plant material and culture conditions
Three selected cassava cultivars, namely TMS 60444, 08/080 and 08/274 were obtained from the Kenya Agricultural and Livestock Research Organization (KALRO)- Mtwapa, Kenya. TMS 60444 is a model genotype in cassava regeneration and transformation and was used as a control in the study because of the excellent regeneration capacity of its embryogenic tissues (Zainuddin et al., 2018). In vitro cultures were established by culturing surface sterilized cuttings (5 cm long) on cassava micro-propagation medium (CMM) containing MS salts with vitamins (Murashige and Skoog, 1962), 3% sucrose, 0.3% gelrite; pH 5.8 and were sub-cultured every four weeks. All cultures except for callus induction were maintained in the growth chamber at 25±2°C, 16 h /8 h light/dark cycle. Cassava mother stock and regenerated plants were grown in a glasshouse at 27±1°C.
Effect of explant type on callus induction and somatic embryogenesis
Immature leaf lobes (ILL) and stem internodes (both 5 to 10 mm long) from 8 weeks old in vitro plantlets were excised and cultured in callus initiation media for 8 weeks in the dark at 25±2°C. Callus were sub-cultured onto fresh media after every 2 weeks. The callus induction media was composed of MS salts, Gamborg B5 vitamins (Gamborg et al., 1968), 100 mg/l myo-inositol, 0.2% sucrose, 0.5 mg/l CuSO4, 50 mg/l casein hydrolysate) supplemented with picloram at concentrations 4, 6, 8 and 10 mg/l. The type of callus, number of explants producing callus and direct somatic embryos were recorded after 8 weeks of culture.
Maturation of somatic embryos
Callus (8 weeks old) were transferred to MS medium supplemented with various hormone combinations of α-naphthalene acetic acid NAA, 6-benzylaminopurine (BAP) and gibberellic acid (GA3), The media were abbreviated M, M1, M2, M3 and M4 (Table 1). The number of cotyledonary embryos formed from each callus was recorded following 4 weeks of culture. The percentage maturation was the number of callus that produced cotyledonary embryos as a percentage of the total number of callus transferred to maturation media. The mean number of cotyledonary embryos was assessed by determining the average number of cotyledonary embryos produced from each callus.
Embryo germination and plant recovery
Callus harboring cotyledonary embryos and shoots were aseptically transferred to hormone free MS media supplemented with 0.8% activated charcoal for 14 days. Embryos with defined root and shoot apices were transferred and maintained on CMM for 4 weeks. The number of germinated plantlets/callus cluster was recorded.
Acclimatization
Plantlets with defined roots and shoots were transferred to small pots filled with sterile peat moss for four weeks in the glass house.
After the lapse of four weeks, the surviving plants were transferred to larger pots filled with a mixture of peat moss and soil (50/50 % v/v). After four weeks, surviving plants were transferred to soil. The ability of regenerated cassava plants to acclimatize and efficiently establish in the glasshouse was assessed by measuring the increment in height during each stage of acclimatization and the number of plants that survived for up to 3 months after transplanting to soil.
Study design and statistical analysis
For callus induction, ten explants were cultured on a 90 mm petri dish and a total of 60 explants were tested for each treatment; the experiment was repeated thrice. The frequencies for callogenesis and embryogenesis were calculated as the number of callus or embryos formed as a percentage of the total number of explants cultured. Frequencies of callus induction, somatic embryogenesis and shoot formation were subjected to analysis of variance (ANOVA) using SPSS statistics program (SPSS software for Windows release 10.0; SPSS Inc., Chicago, IL) and differences among treatment means were separated by Duncan’s multiple-range test (DMRT) at a 95% confidence level (P≤0.05). All quantitative data values were expressed as Mean ± SEM (n=3).
Effect of explant source on callogenesis and somatic embryogenesis
Immature leaf lobes (ILL) took an average of 10 days to initiate callus while stem explant took up to 15 days. Callus formation in immature leaf lobes (Figure 1A) started off by the formation of a swollen mass of tissue at the cut edges of the leaf (Figure 1B) then spread into the entire leaf. Callus from meristematic stem segments were mainly loose, friable, non-embryogenic and white in color (Figure 1C) while callus from immature leaf lobes were mainly translucent, gelatinous and highly embryogenic (Figure 1D). Although no significant differences were noted in the ability of stem and leaf explants to form callus (Table 2), significant differences were noted in their ability to form organized embryogenic structures (Figure 2). Callus from leaf explants gave significantly higher embryogenesis frequencies than the stem explants in all the tested cultivars. An increase in the concentration of picloram led to an increase in the frequency of callus formation and embryogenesis in all the cultivars tested. Embryogenesis frequencies for ILL in cultivars 08/274, TMS 60444 and 08/080 ranged from 62 - 84, 70 - 83 and 46 - 63%, respectively. Embryogenesis frequencies for stem explants ranged from 38 - 44, 30 - 39 and 28 - 29%, respectively. Among the three cultivars tested, 08/274 gave the best response for somatic embryo formation using both leaf lobes and stem internodes (Figure 2).
Effect of varying plant growth regulator combinations on embryo maturation
Results on somatic embryo maturation and number of cotyledonary embryos per callus showed that both parameters varied across each media formulation and cultivar (Table 3). Embryogenic callus obtained from stem explants turned green in maturation media but neither formed distinct cotyledons nor plantlets (Figure 1E). Immature leaf lobe derived embryos matured to form either two distinct cotyledons, one cotyledon or fused cotyledons, but germination was attained in all the three cotyledon morphologies. Cotyledonary embryos either formed cotyledonary leaves (Figure 1F) or a collar like cotyledon, which ultimately formed a horn like structure (Figure 1G). Calli cultured on M and M1, both of which had high BAP concentration (3 mg/l) were found to generally produce the fused type of cotyledonary embryos (Figure 1F). Most of the cotyledonary embryos from these media formulations did not, however, convert to complete plantlets hence significantly lower (P≤0.05) maturation frequencies were obtained from the two combinations (62 and 63% respectively, Table 2). The two hormone combinations produced an average of 5 and 6 shoots per callus respectively (Table 3). Media M3 and M4 both of which had relatively low BAP concentrations (1 mg/l) and high GA3 (1.5 mg/l) concentrations gave embryo conversion rates of 69% (Table 3). For these two media formulations, averages of 14 and 16 shoots were recovered respectively (Table 4). It was also observed that cotyledonary embryos from media formulations M3 and M4 either had only one cotyledon or two but none was fused (Figure 1H).
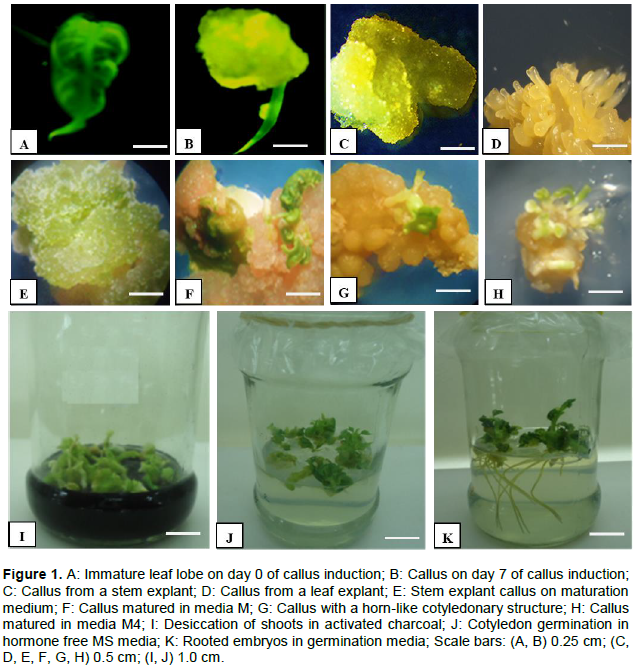
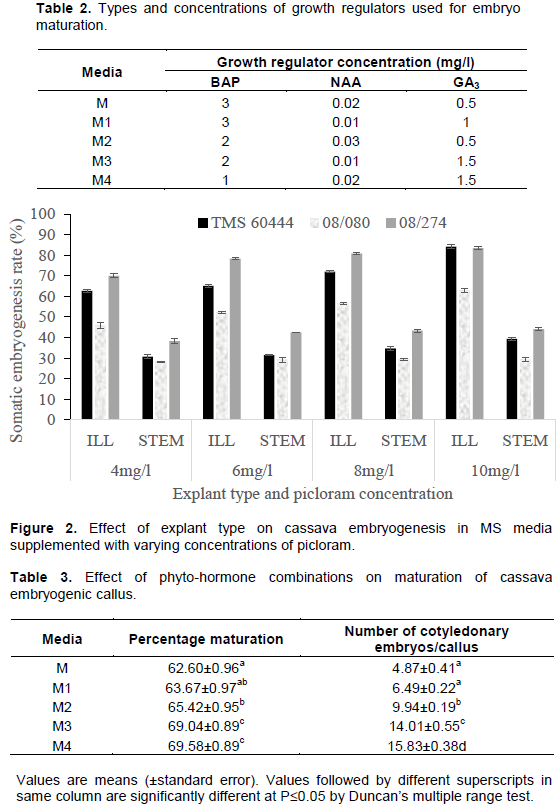
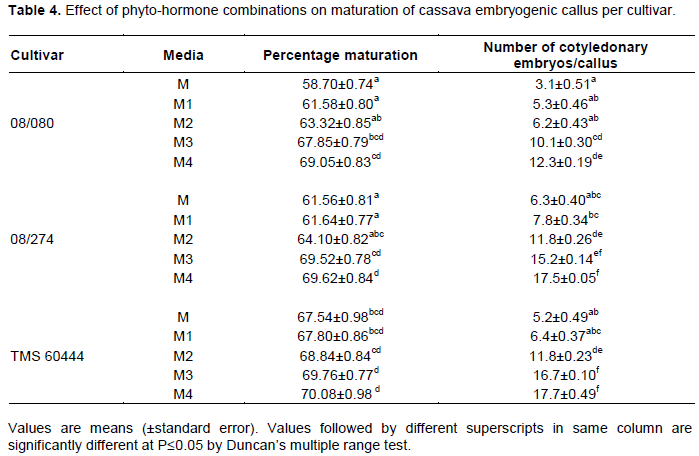
Embryo germination and plantlet acclimatization
Embryo germination started off by the gradual opening of cotyledonary leaves and horns from where a shoot emerged (Figure 1I). Shoots (Figure 1J) of all cultivars successfully established roots (Figure 1K) on cassava basic medium. Regenerated plants were phenotypically similar to the mother stock plants in the glass house and their growth rate was normal. Well-developed cassava plantlets measuring 8 to 10 cm (Figure 3A) were able to establish in peat moss. During the 1-month period, an increase in the height of cassava plants up to an average of 15 cm was observed (Figure 3B). Gradual removal of the polythene bag by first punching holes then removing it after a month was found optimal for efficient cassava acclimatization (Figure 3C). Growth of the cassava plants in a mixture of peat moss and soil for 1 month led to further plant elongation (Figure 3D, 30 cm in height). Plantlets from all the three cassava cultivars were able to efficiently establish in the glasshouse. Three months after transplanting cassava to soil, the plants were found to elongate further to a height of 60 to 90 cm (Figure 3E). In our study, all cassava cultivars attained a survival rate of 60%. Overall, the regeneration efficiency of cassava cultivar 08/080(48.1%) was significantly lower (P≤0.05) than that of cultivars 08/274(75.1%) and TMS 60444(76.3%)
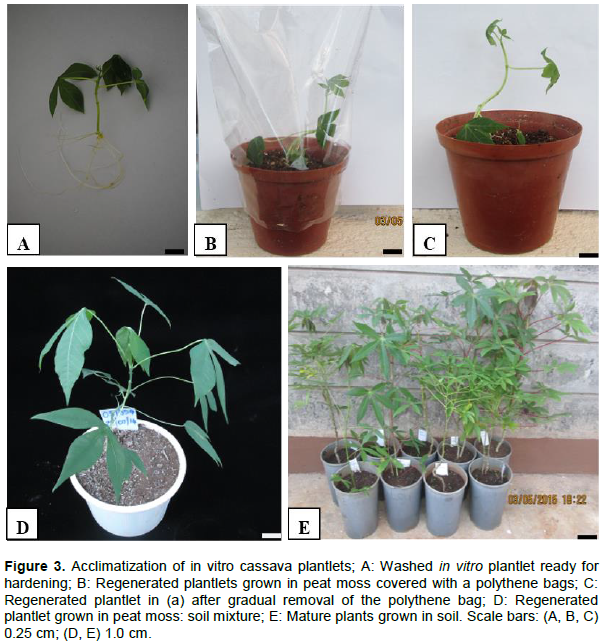
Auxins are significant plant growth regulators for stimulating the embryogenic competence of somatic tissues (Gaj, 2004; Lincy et al., 2009). Different explants of the same plant species, however, respond differently to the same hormone treatment. In the present study, immature leaf lobes cultured in picloram gave a better response for somatic embryogenesis as compared to stem explants in the three cassava cultivars tested. A similar response was reported in Quercus robur L. where leaf explants gave an embryogenesis frequency of 16% while stem internode segments gave an embryogenesis rate of 4% in media containing NAA (Cuenca et al., 1999). This trend is also consistent with the report by Xu et al., (2014) who noted that the response of Solanum nigrum leaf explants cultured in auxin media was better than that of stem explants cultured in the same medium. Differences in the response of different explants to somatic embryogenesis could be attributed to their differences in morphological structure and stages of maturity (Schädel et al., 2010). The flat shape of leaf explants as opposed to the round shape of stem explants allowed for a bigger leaf surface area to be in contact with the media hence a better embryogenesis response. Additionally, the differential response by the two explants could be attributed to fact that leaves are less lignified as compared to stem explants making them more responsive to regulatory hormones and easily programmed to undergo dedifferentiation (San-José et al., 2010; Schädel et al., 2010; Twumasi et al., 2009). This study noted that when stem segments were cut and poked, they produced latex that possibly interfered with the formation of somatic embryos.
Indirect somatic embryogenesis in cassava previously relied on the use of the auxin 2,4-dichlorophenoxy acetic acid (2,4-D) for initiation of embryogenic competence on different tissues (Danso et al., 2010). This has however been found to lead to low rates of primary embryogenesis and poor plant recovery rates owing to lack of root primordium especially when the culture period in callus induction media is prolonged (Raemakers et al., 1993). To enhance somatic embryogenesis system in cassava, it is therefore important to investigate on the potential of other auxins such as picloram or dicamba as well as jasmonates and brassinosteroids especially in cassava landraces whose embryogenic ability is unknown. We investigated the optimum concentration of picloram for somatic embryogenesis in cassava and noted that embryogenesis frequencies increased with increasing concentrations of picloram from 4 to 10 mg/l. The culture of immature leaf explants in 10 mg/l picloram was found optimal for maximum somatic embryogenesis.
High auxin concentrations evoke callogenesis and embryogenesis but impede embryo germination and shoot regeneration. Consequently, most studies use a high auxin/ cytokinin ratio to evoke callus formation after which the auxin level is reduced while increasing the cytokinin level to stimulate embryo germination and shoot formation. According to Uzelac et al., (2007), the poor capacity of somatic embryos to mature and germinate limits the application of somatic embryogenesis for crop improvement in a number of species.
Embryo maturation was successfully achieved in all the tested maturation media formulations. Differences in embryo germination and plant recovery rates were however recorded between the various plant growth regulator combinations tested. Media M3 and M4 were found to be better suited for maximal plant recovery in cassava. Embryo maturation was characterized by the formation of cotyledonary embryos which differentiated into either cotyledonary leaves or a collar like cotyledon that ultimately formed a horn like structure. Alterations in auxin and cytokinin ratios are sufficient to control plant morphogenesis. In Pinus caribaea, it was found necessary to have a 1:2 NAA and BAP ratio to regenerate plants (Akaneme and Eneobong, 2008).
According to Moura, (2009), the low rate of somatic embryo conversion to plantlets has hindered the improvement of Acrocomia aculeate using bio-technological techniques. To improve embryo conversion rates, GA3 is usually added into such systems. In this study, an increase in plant recovery rates was observed with increase in the concentration of GA3 used. Xu et al., (1997) and Yang and Choi (2000) also gave reports of significant stimulatory effects of GA3 in cultures of Sesamum indicum and in P. ginseng, respectively.
Cassava regeneration via somatic embryogenesis can be coupled with genetic transformation and mutation breeding to mitigate the challenges associated with the application of conventional breeding for the improvement of the cassava genome. The regeneration pipeline must hence be optimized to suit each cultivar of interest. This study presents a somatic embryogenesis based cassava regeneration system capable of producing shoots within 5 to 6 months.
The authors extend their thanks to the Eastern Africa Agricultural Productivity Project for funding the study. The authors acknowledge the Plant Transformation Laboratory, Department of Biochemistry and Biotechnology, Kenyatta University, Nairobi, Kenya, for providing the laboratory facilities.
The authors have not declared any conflict of interests.
REFERENCES
Akaneme FI, Eneobong EE (2008). Tissue culture in Pinus caribaea Mor. var. Hondurensis barr. and golf. II: Effects of two auxins and two cytokinins on callus growth habits and subsequent organogenesis. African Journal of Biotechnology 7(6):757-765.
|
|
Alves AAC (2002). Cassava botany and physiology. In Cassava: Biology, Production and Utilization. New York, NY, USA: CABI Publishing, pp. 67-89.
Crossref
|
|
|
Anyanwu CN, Ibeto CN, Ezeoha SL, Ogbuagu NJ (2015). Sustainability of cassava (Manihot esculenta Crantz) as industrial feedstock, energy and food crop in Nigeria. Renewable Energy 81(1):745-752.
Crossref
|
|
|
Chavarriaga-Aguirre P, Brand A, Medina A, Prías M, Escobar R, Mar-tinez J, Díaz P, López C, Roca W, Tohme J (2016) The potential of using biotechnology to improve cassava: a review. In Vitro Cellular and Developmental Biology-Plant 52(5):461-478.
Crossref
|
|
|
Cuenca B, San-Jos M, Martínez M (1999). Somatic embryogenesis from stem and leaf explants of Quercus robur L. Plant Cell Reports 18(7):538-543.
Crossref
|
|
|
Danso K, Elegba W, Oduro V, Kpentey, P (2010). "Comparative study of 2, 4-D and Picloram on friable embryogenic calli and somatic embryos development in cassava (Manihot esculenta Crantz)." International Journal of Integrative Biology 10(2):94-100.
|
|
|
FAO Joint - 2018 - inis.iaea.org. Cassava Production Guidelines for Food Security and Adaptation to Climate Change in Asia and Africa.
View
|
|
|
FAOSTAT (2017). Food and agriculture data.
View
|
|
|
Gaj M (2004). Factors influencing somatic embryogenesis induction and plant regeneration with particular reference to Arabidopsis thaliana (L.) Heynh. Plant Growth Regulation 43:27-47.
Crossref
|
|
|
Gamborg OL, Miller RA, Ojima K (1968). Nutrient requirements of suspension cultures of soybean root cells. Experimental Cell Research 50(1):151-158.
Crossref
|
|
|
Jansson C, Westerbergh A, Zhang J, Hu X, Sun C (2009). Cassava, a potential biofuel crop in China. Applied Energy 86(1):95-99.
Crossref
|
|
|
Lincy AK, Remashree AB, Sasikumar B (2009). Indirect and direct somatic embryogenesis from aerial stem explants of ginger (Zingiber officinale Rosc.). Acta Botanica Croatica 68(1):93-103.
|
|
|
Marius K, Modeste K, Edmond K, Gwladys G, Laurent K, Mongomaké K (2018). Whole Plants Regeneration of Cassava Cultivars (Manihot esculenta Crantz) Originated from Côte d'Ivoire via Somatic Embryogenesis. Journal of Advances in Biology and Biotechnology 19(2):1-11.
Crossref
|
|
|
Moura EF (2009). Somatic embryogenesis in macaw palm (Acrocomiaaculeata) from zygotic embryos. ScientiaHorticulturae 119(2009):447-454.
Crossref
|
|
|
Raemakers C, Bessembinder J, Staritsky G, Jacobsen E, Visser R (1993). Induction, germination and shoot development of somatic embryos of cassava. Plant Cell Tissue and Organ Culture 33(2):151-156.
Crossref
|
|
|
San-José M, Corredoira E, Martínez M, Vidal N, Valladares S, Mallón R, Vieitez M (2010). Soot apex explants for induction of somatic embryogenesis in mature Quercus robur L. trees. Plant Cell Reports 29(6):661-671.
Crossref
|
|
|
Schädel C, Blöchl A, Richter A, Hoch G (2010). Quantification and monosaccharide composition of hemicelluloses from different plants. Journal of Plant Physiology and Biochemistry 48(1):1-8.
Crossref
|
|
|
Schaller GE, Bishopp A, Kieber JJ (2015). The yin-yang of hormones: cytokinin and auxin interactions in plant development. The Plant cell 27(1):44-63.
Crossref
|
|
|
Shen X, Michael E, Jianjun C (2015). Effects of genotype, explant source, and plant growth regulators on indirect shoot organogenesis in Dieffenbachia cultivars. Journal of In Vitro Cellular and Developmental Biology, Plant 44(4):282-288.
Crossref
|
|
|
Twumasi P, Schel H, van W, Woltering E, van O, Emons M (2009). Establishing in vitro Zinnia elegans cell suspension culture with high tracheary element differentiation. Cell Biology Integrated 33(4):524-533.
Crossref
|
|
|
Uzelac B, Ninković S, Smigocki A, Budimir S (2007). Origin and development of secondary somatic embryos in transformed embryogenic cultures of Medicago sativa. Biologia Plantarum 51(1):1-6.
Crossref
|
|
|
Wongtiem P, Courtois D, Florin B, Juchaux M, Peltier D, Broun P (2011). Effects of cytokinins on secondary somatic embryogenesis of selected clone Rayong 9 of Manihot esculenta Crantz for ethanol production. African Journal of Biotechnology 10(9):1600-1608.
|
|
|
Xu Q, Jia F, Hu D (1997). Somatic embryogenesis in Sesamum indicum L. cv. Nigrum. Journal of Plant Physiology 150(6):755-758.
Crossref
|
|
|
Xu K, Chang Y, Liu K, Wang F, Liu Z, Zhang T, Li T, Zhang Y, Zhang F, Zhang J, Wang Y, Niu W, Jia S, Xie H, Tan G, Li C (2014). Regeneration of solanum nigrum by somatic embryogenesis, involving frog egg-like body, a novel structure. PLoS ONE 9(6):e98672.
Crossref
|
|
|
Yang C, Choi E (2000). Production of transgenic plants via Agrobacterium rhizogenes-mediated transformation of Panax ginseng. Plant Cell Reports 19(5):491-496.
Crossref
|
|
|
Zainuddin M, Fathoni A, Sudarmonowati E, Beeching J, Gruissem W, Vanderschuren H (2018). Cassava postharvest physiological deterioration: From triggers to symptoms. Postharvest Biology and Technology 142:115-123.
Crossref
|
|