Arabian Gulf is characterized by being nutrient-rich, which contributes to the biodiversity of marine macro- and microalgae. Marine algae are a potentially prolific source of many valuable products that may represent useful leads in developing the quality of human life. The studies on the bioactivity of marine algae in the Arabian Gulf region are very recent; thus, there are not many studies on this field. This review paper is focus on the importance of algae in various fields, and the benefit of such organisms to solve many environmental and health problems to develop the economy, and healthcare of surrounding Gulf countries by taking advantage of many common algal species present in the Arabian Gulf waters. Studies focuses on potentially using local algal species to control common environmental problems in the region such as harmful algal blooms, oil spills, and in wastewater treatment. Furthermore, they were a potential source of food and feed, and can be used in medical field for the treatment of many common diseases. This review describe researches involving the most common algal species found in Arabian Gulf contributing for the bioactive compound’s isolation, for its productivity in large scale, and for its potential biotechnological applications.
The information was selected from the following databases: PubMed, Web of Science, PubChem, SciFinder and LILACS. All original articles were included indexed in the period 1985-2019. The following uniterms were used: Arabian gulf, Biotechnology; Marine algae; seaweeds; algae processing; pharmacologically active compounds; algae as food; aquaculture feed; algae oil content.
BIOLOGY OF ALGAE
Algae are photosynthetic organisms that uses sunlight to produce energy through photosynthesis process. They are made up of eukaryotic cells that contains nuclei and organelles. All algae have plasmids that contains chlorophyll for photosynthesis process. However, they differ with chlorophyll combinations and carried pigments (Howe et al., 2008). Also, they vary in size ranging from unicellular microscopic algae (microalgae) such as cyanobacteria to more visible and complex multicellular algae (macroalgae or seaweeds). Marine algae comprises a variety of species, types, and groups that are unique in their characteristics. With over 150,000 species already identified and with many more yet to be identified, algae are classified into multiple major groupings as follows: Cyanobacteria (Cyanophyceae), green algae (Chlorophyceae), red algae (Rhodophyceae), brown algae (Phaeophyceae), yellow-green algae (Xanthophyceae), golden algae (Chrysophyceae), dinoflagellates (Dinophyceae), diatoms (Bacillariophyceae), and ‘pico-plankton’ (Prasinophyceae and Eustigmatophyceae) (Algaebase, 2020). These plants proliferate in aquatic environment, mainly in the sea, with some species having the ability to grow in freshwater environment (Fitzsimons et al., 1996).
Marine macroalgae
Marine macroalgae also known as seaweeds are macro-autotrophic, multicellular marine plants that are found
from intertidal to shallow subtidal zones. The macroscopic algae are classified into three major groups: Chlorophyta (green algae), Rhodophyta (red alga), and Phaeophyceae (brown algae) (Koch et al., 2013). They play a major role in marine coastal ecosystems food web by their ability of recycling nutrients. In addition, they support the growth of different associated species by providing them with a physical structure (Koch et al., 2013; Leopardas et al., 2014). Marine macroalgae have been used as food sources and crude pharmaceutical products in most East Asian countries especially Japan and China. It has been used in the treatment of many diseases such as iodine deficiency (Basedow’s disease, goiter, and hyperthyroidism). Some seaweeds were provided as a source of vitamins to support other treatments for patients with various intestinal disorders (such as vermifuges, and as hypocholesterolemic and hypoglycemic agents) (El Gamal, 2012). In addition, macroalgae extracts were included in many industrial and biotechnological applications like, cosmetics, organic fertilizers, medicine against various microbial infections, biofuel and more (Salehi et al., 2019).
Marine macroalgae use
Marine algae are considered as the most important marine resources in the world. They represent a source of food, different types of feed, and medicine in the east as well as in the west countries (Kovac et al., 2013). Marine macroalgae in the Arabian Gulf were distributed along the seashore of the surrounded countries. The biomass and diversity of marina algae in the Arabian Gulf region have been studied and documented. In the Arabian Gulf, 299 species of algae were cited (Algaebase, 2020) and about 44% of them have some potential biotechnological application (Table 1). Seaweeds in this region were tested to be used in different applications:
Pharmaceutical applications: Marine algae play a major role in the pharmaceutical industry due to their bioactivity. They may produce low and high molecular weight bioactive metabolites that can be used as a prototype of new medicines. The synthesized bioactive compounds by algae includes pigments (example, carotenoids, xanthophylls, chlorophyll and terpenoids), vitamins, saturated and polyunsaturated fatty acids, amino acids and antioxidants (example, polyphenols, alkaloids and halogenated compounds) and polysaccharides (example, agar, carrageenan, proteoglycans, alginate, laminaran, rhamnan sulfate, galactosyl glycerol and fucoidan) (Dhargalkar and Pereira, 2005; Smit, 2004). Many of these compounds were discovered to have anti-bacterial, anti-fungal, algicidal, anti-viral, anti-protozoan and various pharmaceutically-relevant activities (Lakshmi et al., 2006; Bowman, 2007) that can treat cancer, diabetes, arthritis, acquired immune deficiency syndrome (AIDS), and other diseases (de Almeida et al., 2011). The composition and content of these bioactive compounds mainly depend on the algae species and the environmental factors such as season and temperature variation (Sardari and Nordberg Karlsson, 2018)
1. Antimicrobial activity: Seaweeds are known for their antimicrobial activity against different pathogens such as bacteria, fungi, protozoa and viruses. Many studies were done on the most dominant algal species in the Arabian Gulf region to test their microbicidal activity. Sargassum latifolium is an example of marine flora that can produce an antibacterial agent that acts against shrimp pathogenic bacteria (Dashtiannasab et al., 2012). While different brown, green and red algae such as Caulerpa sertularioides, Gracilaria corticata, Gracillaria salicornia and Sargassum oligocystum species have an anti-Leishmanial activity (anti-protozoan) against Leishmania protozoa (Fouladvand et al., 2011). Sargassum oligocystum (collected from the Arabian Gulf) was also tested in another study showing its property to produce antibacterial activity against Staphylococcus aureus (ATCC 25923), Staphylococcus epidermidis (ATCC 14990), Pseudomonas aeruginosa (ATCC 27853), and E. coli (ATCC 25922) when extracted in hot water rather than alcoholic extract (Tajbakhsh et al., 2011a). Also, it was discovered that Sargassum oligocystum have remarkable antitumor activity against K562 and Daudi cell lines at concentrations 500 and 400 µg/ml of the algal extract (Zandi et al., 2010). Different studies were done on brown algae genus Cystoseira showed that different species showed different bioactivities against microbial community. A research done by Tajbakhsh and colleagues showed that Cystoseira trinodis have activity against S. aureus, S. epidermidis, E. coli, and P. aeruginosa bacterial species (Tajbakhsh et al., 2011b). While Cystoseira myrica has virucide activity against herpes simplex virus type 1 (Zandi et al., 2007).
2. Antioxidants: Marine plants and algae play a major role in human diet due to its richness in vitamins and minerals and the existence of phenolic compounds and flavonoids in all parts of the plant that acts as anti-oxidants in the human body (Khan and Satam, 2003; Mathew and Abraham, 2006). Plant-derived secondary metabolites, such as antioxidant compounds, help in cleaning the accumulated free radicals in the human body that may result in DNA and cell damage (Bergamini et al., 2004). Few studies were available on the antioxidant property of macroalgal species extracts from Arabian Gulf. It was discovered that different species of green, brown and red algae studied such as Laurencia snyderia, Acanthophora nayadiformis, Gracilaria corticata, Sargassum tenerrimum, and green algae Chaetomorpha.
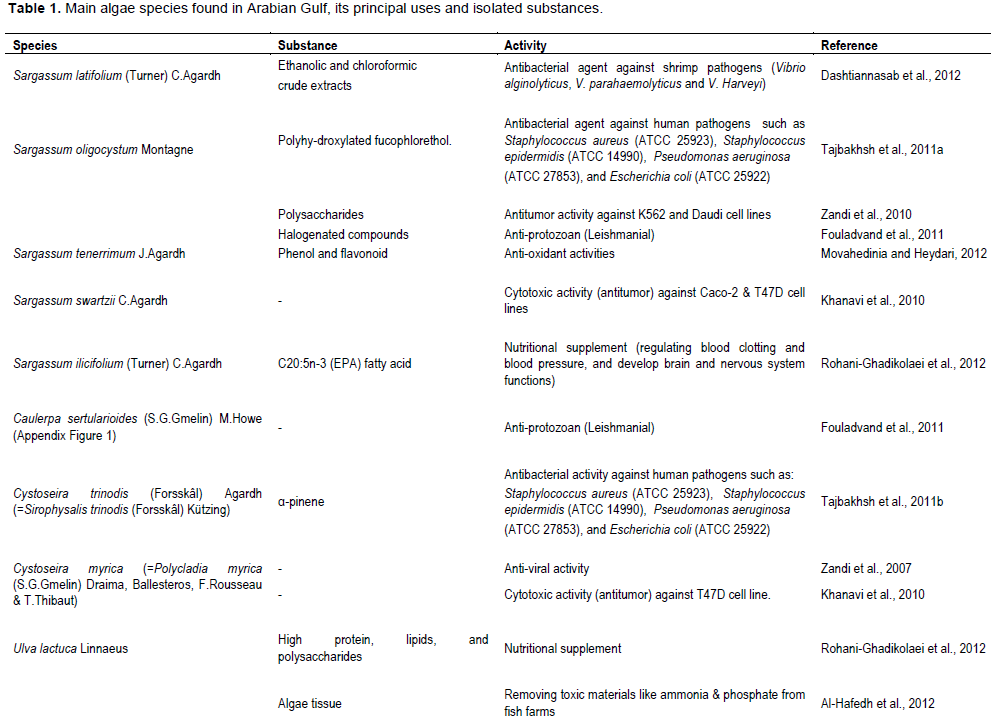
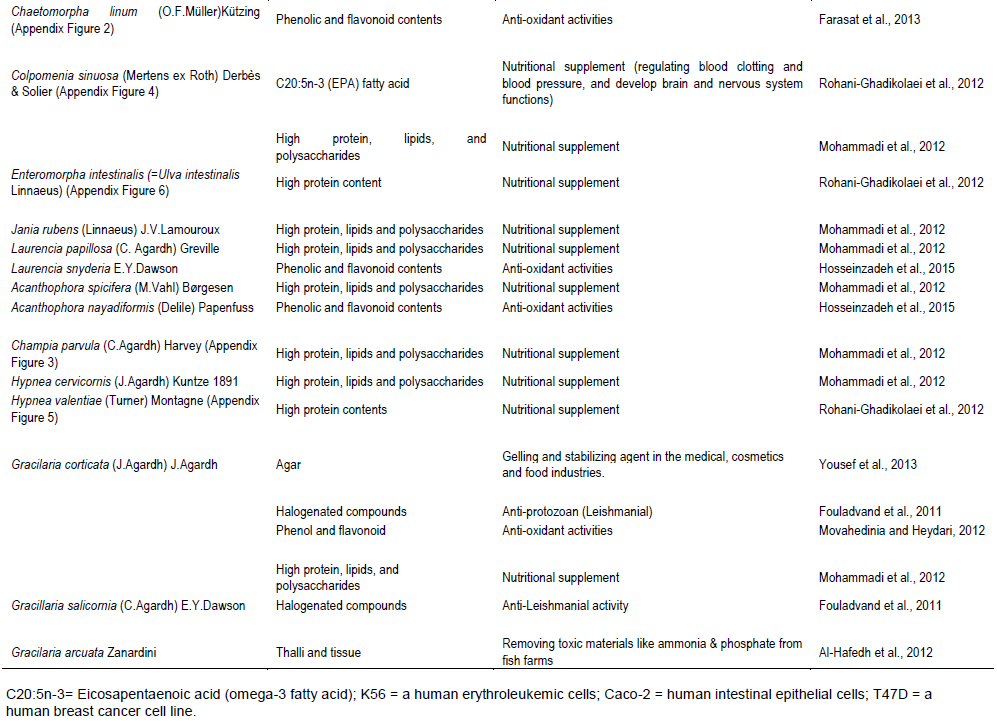
Genus showed antioxidant activity (Farasat et al., 2013; Hosseinzadeh et al., 2015; Movahedinia and Heydari, 2012). Several studies on Arabian Gulf seaweeds revealed the fact that Sargassum sp. has the highest antioxidant activity (2,2-Diphenyl-1-picrylhydrazyl (DPPH) free radical scavenging and Fe3+ reducing power) when compared with other tested seaweeds (Moubayed et al., 2017; Sadati et al., 2011). From Horincar's study, the antioxidant activity of marine algae depend mainly on algal species and the solvent used in extraction (Horincar et al., 2011). That, there is a positive correlation between the increase in algae phenolic content and antioxidant properties, because phenolic compounds act as electron donors and help in balancing the reactions created by free radicals in the body (Moubayed et al., 2017; Sadati et al., 2011). A recent study on seaweeds collected from the Kuwait coast revealed that the phenolic extracts of 26 tested seaweeds showed high antiradical and reducing power. In which the ethanol was efficient in extracting polyphenols, steroids, glycosides, tannins, and saponins, while water was efficient in extracting polysaccharides and proteins. The results showed that the seaweeds from this region with extreme climatic conditions produce more flavonoids than phlorotannins when compared with the same species at temperate climate. All Sargassum sp. and some other species like Canistrocarpus cervicornis, Feldmannia irregularis, Polysiphonia platycarpa, Chondria sp., and Cladophora sp., showed high antioxidant activity and they were rich in polyphenolic compounds. Moreover, almost all the tested seaweeds contained hydroquinone, which is known as a skin whitening agent. Thus, the properties of seaweeds from this region qualify them to be used in various industries (Farvin et al., 2019a). Then, to increase the yield of bioactive compounds extraction from the same tested seaweeds, Farvin et al. (2019b) used five carbohydrases and three proteases enzymes to screen seven selected brown seaweeds for antioxidant and antimicrobial activity. The results showed that bioactivity defers among seaweeds and enzymes used for extraction. As an example, Sargassum boveanum-Viscozymes and Sargassum boveanum-Alcalase showed high antioxidant activity, while most of the seaweeds extracted by Flavourzyme showed antimicrobial activity. Thus, the study concluded that each seaweed species needs a specific enzyme to increase the yield of bioactive compounds.
3. Cytotoxic compounds: Tropical and subtropical algae were an important source of many natural products that can help in developing many drugs against cancer, microbial infections and inflammation (Cardozo et al., 2007; Stein et al., 2011). They also act as a source of protein, agar, carrageenan, alginates, vitamins and minerals that can be used in medical fields for the treatment of some diseases and related symptoms such as children fever, digestive disorders, joint pains, and cancer and used as sedatives and antibiotics (Iwashima et al., 2005; Mosaddegh et al., 2014). It was discovered that certain compounds in marine algae such as fucoidans, laminarians, and terpenoids have cytotoxic property against cancer cells by inhibiting their proliferation (Smit, 2004). The cytotoxic effects of algal extracts on different cell lines are currently being studied (Isnansetyo et al., 2017; Tannoury et al., 2017). Within these studies, cytotoxic activity against cancer and tumor cell lines, as well as immunomodulatory activity, has become one of the most important specificities of algae (Anastyuk et al., 2017; Khalifa et al., 2016). The bioactive compounds in algal extracts and isolates were used in the medical field in treating toxic cells in human body such as cancer cells by following three different scenarios. They can cause necrosis (cell lysis), apoptosis (a genetically controlled form of cell death), and decrease in cell viability by stopping the division of actively growing cells (Fadeel, 2004). The recent studies in this field are mainly focusing on elucidating the specific compounds that demonstrate such bioactivities. Also studying the synergistic effect of different bioactive compounds within algal extracts have attracted the interest of the scientific community, since the interactions between these substances increase the bioactive capacity (Choi et al., 2017).
The cytotoxic activity studies of seaweeds from the Arabian gulf region against human cell lines have started since 2010. A study on three different brown algal species Sargassum swartzii, Cystoseira myrica and Colpomenia sinuosa collected from the Arabian Gulf water showed that different fractions of these algae exhibited cytotoxicity against different cancer cell lines (HT-29 (human colon carcinoma), Caco-2 (human epithelial colorectal adenocarcinoma cells), T47D (breast cancer cell) and NIH 3T3 (mouse embryonic fibroblast cell)). The cytotoxicity of these algal extracts activates an estrogen receptor-independent mechanism in breast cancer cells, which promote cell proliferation and tumor progression (Khanavi et al., 2010). Another study on the cytotoxicity of Arabian gulf seaweeds species against cancer cells showed that the alcoholic extracts of Gracillaria corticata, Ulva fasciata, and Sargassum ilicifolium were antiproliferative against all the tested cancer cell lines (MCF-7 (human breast carcinoma cell line), MDA-MB-231 (human epithelial breast cancer cell), HeLa (cervical carcinoma), HepG-2 (human hepatocellular carcinoma cell line), and HT-29 (human colon carcinoma). While the methanolic extract of Gracillaria corticata showed the highest inhibition activity against MCF-7 cell line, which could induce apoptosis in human breast cancer in time and dose depended manner (Namvar et al., 2014). Ghannadi et al., (2016) did a study to compare the cytotoxicity of two Gracillaria species (red seaweed) collected from the Arabian Gulf water against different human cell lines. The obtained results showed that both Gracillaria species (G. corticata and G. salicornia) have cytotoxic activity against all the tested cell lines (HT‑29, HeLa, and MCF‑7), but G. salicornia activity was a little bit higher (HT‑29= 68.2 µg/ml, HeLa= 125.5 µg/ml and MCF‑7= 185.8 µg/ml). The production of algal extracts for these two species is easy and cost-effective; thus, the study suggested to use them as a therapeutic tool in the form of food or drug against human cancer (Ghannadi et al., 2016). The cytotoxicity of G. salicornia was also tested by Efrani showing a higher toxicity effect (IC50>400 μg/ml) against MCF‑7 cel line (Erfani et al., 2015) when compared with Ghannadi study (MCF‑7= 185.8 µg/ml) (Ghannadi et al., 2016). The differences in the activity results between both studies may be a consequence of different solvents used for extraction, season and time of collection, and also different methods used.
Food applications: Marine algae are known for their nutritional value because they are rich in protein, lipids, carbohydrates, and other essential elements. They are used as food and feed sources for fish farming due to their addedâ€value dietary ingredients. Besides their nutritional value, seaweeds contain a number of pigments, defensive and storage compounds, and secondary metabolites that could have beneficial effects on farmed fish (Wan et al., 2018). Many studies were done on different macroalgae species collected from the Arabian Gulf to study their nutritional composition. Six seaweeds collected from the Arabian Gulf evaluated for their nutritional value and if these macroalgae can be used as a potential source in aquaculture nutrition. The study showed that all the tested species have high nutritional value, rich in protein, lipids, carbohydrates, and essential elements. The collected seaweeds were high in carbohydrate (31.8 to 59.1% dry weight), ash (12.4 to 29.9%) and minerals (K, Mg, Fe, Mn, Cu, Zn and Co) but low in lipid content (1.5 to 3.6%). The tested red (Gracilaria corticata and Hypnea valentiae) and green seaweed (Ulva lactuca and Enteromorpha intestinalis) have high protein content (p < 0.05) compared to brown seaweeds. Moreover, the green algae U. lactuca was rich in carbohydrates (59.1%) and lipids (3.6%). While the brown algae (Colpomenia sinuosa and Sargassum ilicifolium) were rich in C20:5n-3 (EPA) fatty acid (5.4 ± 0.86 and 1.9 ± 0.22, respectively) compared with the other tested seaweeds, which is health-beneficial eicosapentaenoic acid that used as nutritional supplement for human diet, helping in regulating blood clotting and blood pressure, and develop brain and nervous system functions (Rohani-Ghadikolaei et al., 2012). The tested seaweeds were rich in essential elements that were absorbed from the seawater and accumulated in seaweed thalli (Azmat et al., 2006). The differences in their mineral composition and concentration are due to species and location specificity. Therefore, they concluded that these seaweed species could be a potential source of food or feed additive. Agar is also an important polysaccharide that broadly used as a gelling and stabilizing agent in different applications like food, cosmetics and medical industries (Villanueva et al., 2010). Some macroalgal genera that are common in these regions, such as Gelidium and Gracilaria are known as agar-containing seaweeds. These two genera give high-quality agar that commercially produced by France, Indonesia, the Republic of Korea, Mexico, Morocco, Portugal and Spain (McHugh, 2003). Gracilaria sp. is common in the northern part of the Arabian Gulf and considered as the major source of commercially valuable agar around the world (Marinho-soriano and Bourret, 2003). A study done by Yousef et al., (2013) to test the best conditions for agar extraction from Gracilaria corticata revealed that agar properties are highly affected by experimental variables except for socking time that had less effect. Thus, a soaking time of 1 h at 40°C with a ratio of 1:200 of algae-to-water and then extraction for 2.5 h at 80°C is recommended to achieve a better quality agar. However, to increase agar yield, a soaking time of 0 h at 40°C with a 1:100 algae-to-water ratio followed by extraction for 2 h at 60°C must be done.
Another study by Mohammadi et al., (2012) on eight seaweed species (Caulerpa sertulariodes, Colpomenia sinuosa, Acanthophora spicifera, Champia parvula, Hypnea cervicornis, Gracilaria corticata, Jania rubens and Laurencia papillosa) belonging to different orders (Rhodophyta, Chlorophyta and Phaeophyta) showed that the eight analyzed seaweeds have valuable nutritional compositions. This was due to their high levels of proteins, carbohydrate and fat owning in their cells (Mohammadi et al., 2012). Thus, they can provide dietary alternatives to the human diet to improve the nutritive value.
Seaweeds act as biofilters that increase the sustainability of intensive fish farming by filtering and recycling fish waste. Some studies on common seaweeds in the Arabian Gulf water concluded that Ulva lactuca and Gracilaria arcuata species were both suitable for integrated aquaculture and bioremediation by removing toxic materials like ammonia and phosphate from fish farms (Al-Hafedh et al., 2012).
Controlling harmful algal blooms (HABs): Harmful algal blooms (HABs) occur more frequently in the Arabian Gulf waters. The bloom of these microalgal species causes harm to marine organisms due to their ability to produce toxins. HAB species toxins kill marine organisms either directly or by accumulating in the food web causing mortality for fish, seabirds, marine mammals and humans when consuming affected seafood products (Al Shehhi et al., 2014). The Arabian Gulf area has experienced serious economic losses (Kuwait, Saudi Arabia, Iran, UAE, Oman, Bahrain and Qatar) during 2008-2009 when dinoflagellate algae known as Cochlodinium polykrikoides bloomed and killed thousands of tons of fish. This affected the economy of the Arabian Gulf countries by impacting tourism, blocking desalination plants, obstruct traditional fisheries and damaged coral reefs (Mindy et al., 2010). To control such problem, a study was done on polyunsaturated fatty acids (PUFAs) in green algae (Ulva fasciata; collected from Japan) showed an algicidal activity against harmful algal blooms caused by different micro-algal species such as Chattonella antiqua, C. marina, Fibrocapsa japonica, Heterosigma akashiwo, Karenia mikimotoi, Heterocapsa circularisquama, Prorocentrum minimum, P. sigmoides, Scrippsiella trochoidea, Alexandrium catenella and Cochlodinium polykrikoides. The activity of the isolated PUFAs (C16:4 n-3, C18:3 n-3, and C18:4 n-3) is limited to the specified microalgae without causing any harm to the surrounding marine living organisms. The produced allelochemical (PUFA’s) were highly recommended for HAB’S controlling mechanism because of their high inhibition effect at very low concentration, ecologically accepted and, their high biodegradability (Alamsjah et al., 2008). Thus, they can be used in the Arabian Gulf region to end frequently occurring harmful algal blooms (HAB) without affecting the surrounding ecosystem.
Marine microalgae
The Arabian Gulf is known for its unique hypersaline, semi-arid and nutrient-rich environment (Quigg et al., 2013). The abundance and the diversity of marine microalgae (phytoplankton) in the regional waters were well studied. The identified phytoplankton taxa in the Arabian Gulf waters and Oman Sea reached up to 376 in 2016 as described by Polikarpov et al., (2016), dominated by Dinophyta phylum that represents the most diverse group. Every year new microalgal species were introduced to the Arabian Gulf water via transporting ships ballast water discharge, that when proliferate can cause a serious economic and environmental problem (Al-Yamani et al., 2015). Generally, phytoplankton normally exists in the Arabian Gulf waters, but at certain favorable environmental conditions, they bloom affecting the surrounding marine organisms. Some species may produce toxins that can cause immortality for marine organisms, while others may discolor the surface water, thus blocking the sunlight for deeper organisms (Richlen et al., 2010). In 1999, a massive fish kill occurred in Kuwaiti waters due to the bloom of Gymnodinium species that produce a toxin called gymnodimine (Heil et al., 2001). Again in 2008-2009, the bloom of Cochlodinium polykrikoides resulted in killing thousands of tons of fish due to the secretion of biologically active metabolites such as cytotoxic agents and mucus substances (Richlen et al., 2010). On the other hand, the proliferation of microalgae can be beneficial by producing high levels of oxygen, participating in the global carbon cycle by fixing carbon and, act as a feed source for other higher organisms like fish larvae (Shams et al., 2012). Most of the phytoplankton species are known for their richness in bioactive compounds like pigments and lipids (Mimouni et al., 2015), that have anti-oxidant, anti-inflammatory, anti-mutagenic, anti-cancer, anti-obesity, anti-allergic activities, and cardio-neuro-, hepato- and photoprotective effects when combined together or either alone (Heydarizadeh et al., 2013).
Lipid contents of microalgae
Microalgae is a promising energy source for biofuel production in the Persian/Arabian Gulf. Since 2010, the Arabian Gulf countries focused on finding an alternative biofuel source rather than petroleum due to the depleting supplies. Many microalgal species in the Gulf area can accumulate high levels of lipids that can be extracted and converted to biodiesel, green diesel, or green jet fuel (Najafi et al., 2011; Wilson et al., 2012). Some microalgal species like Nannochloropsis, Chlorella, and Dunaliella sp. (common in the Arabian Gulf waters) were studied in a small indoor scale since 2004, started from Kuwait for commercial use and biofuel production. The advantage of producing biofuel from microalgae is summarized by their higher growth rates and productivity per unit land area, with the ability to use the non-fertile land for their reproduction (Williams and Laurens, 2010). Algal biofuel production mainly depends on algal biomass that varies among species (Ben-Amotz et al., 1987) and growth conditions (Fernandezreiriz et al., 1989). This was supported by research done on three Dunaliella species isolated from Qatar seawater (Arabian Gulf) showed that Dunaliella bardawil was highly productive and showed higher carbon sequestration and photosynthetic efficiencies than D. salina and D. parva. D. bardawil and D. salina were able to thrive in Gulf seawater under high salinity and hot, sunny climate, showing high oil contents in their biomass. Thus, they can be used as a biodiesel feedstock for Qatar (Wilson et al., 2012). In addition, other microalgal species isolated from Arabian Gulf and tested by Moazami et al. (2011) for possible biofuel production, and they were Nanochloropsis (PTCC 6016), Nanochloropsis (PTCC 6003), Nitzschia (PTCC 6001) and Chlorella (PTCC 6022). It was shown that the tested species have high oil contents, high biomass, and high productivity compared with the other tested species (Table 2). They concluding that, the two-isolated Nanochloropsis sp. proved to be suitable for commercial biofuel production (Moazami et al., 2011). Nannochloris sp. (strain QUCCCM31) is another promising biodiesel producing strain in the Arabian Gulf region. This strain was isolated from Qatar seawater and can thrive under high temperature (up to 45°C) and high salinity (35-100 ppt). It has high lipid productivity under harsh conditions and can produce nervonic acid, which is a straight fatty acid having high pharmaceutical potentials (Saadaoui et al., 2016).
To enhance biodiesel production in some microalgal species, certain products can be added to the supported media to increase their lipid productivity. The addition of myoinositol (200 mg/l); which is a stereoisomer of inositol; enhances the lipid productivity of Dunaliella salina to up to 50% and biodiesel quality by increasing auxin and phosphatidylinositol accumulation in the cells (Talebi et al., 2015). While another study on Nanochloropsis sp. showed an increase in biomass productivity when nano-chitosan flocculant agent was added to the culture media; rather than chitosan; to up to 9%. This will result in high biomass recovery that will lead to an efficient cost-effective technique for harvesting microalgae for biofuel production (Farid et al., 2013).
Carotenoid pigments of microalgae
The carotenoid pigment in marine microalgae plays an important role in food, feed, pharmaceutical, and nutraceutical industries due to their strong coloring ability and antioxidative activity (Fraser and Bramley, 2004). Microalgae are known to be the best natural source of carotenoids, especially strains belonging to Chlorophyta such as Dunaliella salina, Nannochloropsis sp. and various Chlorella species (James and Al-Khars, 1990; Zhang et al., 2014). These strains are common in the Arabian Gulf waters especially Dunaliella spp. (El-Gammal et al., 2017). Dunaliella spp. are common in the Arabian Gulf due to its ability to tolerate high salinities (halotolerant), and their blooms will be noticed by the brownish-red color (Al-Hasan and Sallal, 1985). The most dominant species are D. salina (Abu-Rezq et al., 2010a; Saburova et al., 2009) and D. viridis (Alshuaibi et al., 2011). Dunaliella spp. are known for mass production of β-carotene that is used as a food preservative (El-Baky and El-Baroty, 2011). Also, β-carotene in Dunalliela sp. showed a decrease in several types of cancer and degenerative diseases incidents due to the presence of 9-cis and all-trans stereoisomers (comprising 40 and 50% of β-carotene, respectively) (Ben-Amotz, 1999). For that reason, intensive researches were done to test the optimum conditions to increase β-carotene accumulation and harvesting from certain producing species. β-carotene accumulation in Dunaliella salina was discovered to increase when the light intensity (Abu-Rezq et al., 2010b) and salinity increases (Talebi et al., 2013). These two important factors were tested by Kitto and Reginald (2011) to check the best climatic conditions for harvesting β-carotene from D. salina for industrial purposes in UAE (United Arab Emirates). The productivity of D. salina increased from 120,000 cells/ml to 1.2 million cells/ml in day 12 at 43 PSU in outdoor culture ponds during summer. And the β-carotene production was the highest during summer (at 100,000 lux) reaching up to 98.34 pg/cell (Beta-carotene concentration) at 243 PSU salinity due to high light intensity (Kitto and Reginald, 2011).
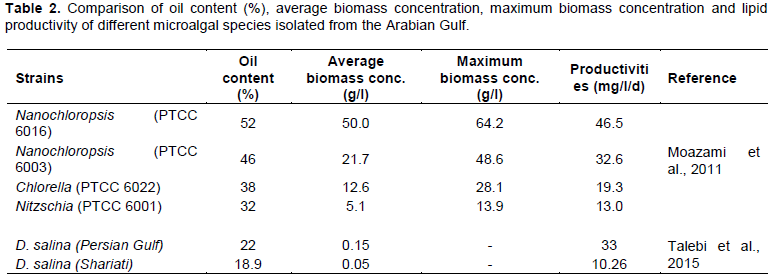
It was discovered that under certain environmental stresses, some marine microalgal species surprisingly increase their pigments production. Since D. salina and C. vulgaris, microalgal species were commonly found in Arabian Gulf waters due to their high ability to tolerate salinity; Talebi et al. (2013) found that there is a correlation between species tolerance to salinity fluctuation and intracellular structure changes. Thus, D. salina resists the extra sodium ions (Na+) in the surrounding environment to up to 2 M by ejecting them externally to maintain the potassium ions (K+) internally balanced and the accumulation of β-carotene was surprisingly increased in order to protect their photosynthetic apparatus. While freshwater Chlorella strain (Chlorella Vulgaris) showed successful adaptation and increased fitness to high salinity levels rather than the saline strain (C. salina) that decreased (Talebi et al., 2013).
Chlorella species are known for their green colour due to the high amount of chlorophyll in their cells which make up 1 to 4% of their weight (Shim et al., 2008). Other pigments like alpha and beta carotenoids, neoxanthin, violaxanthin, zeaxanthin and lutein pigments are commonly found in their cells, except some keto-carotenoids like canthaxanthin and astaxanthin are only found in certain Chlorella sp. (Ip and Chen, 2005). Nannochloropsis sp. is recognized as a great source of lipids and pigments. It contains a variety of pigments with commercial interests like chlorophyll-a, violaxanthin, vaucheraxanthin, zeaxanthin, canthaxanthin, astaxanthin, antheraxanthin and beta-carotene (Jorge et al., 2003; Neto et al., 2018).
Pigments extraction (chlorophylls and carotenoids) from microalgal species could be achieved by four methods, such as ultrasoundâ€assisted extraction (UAE), ethanol as a coâ€solvent, supercritical fluid extraction (SFE), microwaveâ€assisted (MAE), and conventional Soxhlet extraction (Poojary et al., 2016).These techniques are strongly dependent on the type of extraction, the solvent used, temperature and duration of the process. Thus, the novel extraction techniques (MAE and UAE) were shown to provide a higher yield and, in consequence, lower costs compared to traditional solvent extraction techniques like Soxhlet extraction and SFE (Fabrowska et al., 2017). These pigment extraction techniques were tested worldwide on Chlorella and Nannochloropsis, in which these two species are common in the Arabian Gulf waters. However, in the Arabian Gulf region pigments extraction for these two strains yet to be done.
Marine microalgae use
Microalgae have the ability to utilize several compounds, including pesticides, hydrocarbons, endocrine disruptors, and cyanides as carbon and nitrogen sources. Microalgae are known for their cell wall that is made up of carbohydrate structures, which helped in the bio-absorption of toxic chemical agents in wastewater. For that reason, microalgae were used to reduce several compounds including nitrogen, phosphorus, heavy metals, and other pollutants in wastewaters (Hammad et al., 2016).
Wastewater treatment: Chlorella is known for the production of various organic macromolecules of interest (proteins, lipids, starch, carotenoids, minerals, vitamins, and pigments) that need a specific technique to be targeted and producing biomass (Safi et al., 2014). Among Chlorella species, C. vulgaris is the fastest-growing microalgae that can uptake certain nutrients like nitrogen and phosphorus from the surrounding waters, thus it was used in wastewater treatment plants (Kim et al., 2010). Many studies demonstrated that C. vulgaris has two cultivation modes: autotrophic and heterotrophic, and in the two modes can be used in wastewater treatment (Wang et al., 2009). A research done by Ansari et al., (2016) showed that Chlorella vulgaris showed a higher removal rate of total nitrogen (TN), alkalinity and chemical oxygen demand (COD) compared with other species. The removal rate for TN, alkalinity, and COD in the primary treated wastewater was 54, 24-28 and 78-82%, respectively. And the removal efficiency increased in the secondary wastewater, allowing the treated wastewater to be used for other industrial processes (Ansari et al., 2016). In addition, the productivity of C.vulgaris in municipal wastewater was noticed to increase under the elevated concentration of sodium hydrogen carbonate and higher light intensity until a certain value and then decreased. However, both cell biomass and lipid productivity increased under long exposure to daily illumination (Ebrahimian et al., 2014). Qatar municipal wastewater was successfully biotreated by Chlorella sp. and Scenedesmus sp. that showed high biomass yield and higher nitrogen and phosphorus uptake. Then, the microalgae biomass was used as a biofertilizer showing a noticeable increase in plant growth when compared with conventional inorganic fertilizer (Abdul Hakim et al., 2016). The manipulation of some microalgal species may help in enhancing wastewater treatment. This was supported by Ebrahimi et al. (2016) work that modified green algae Cladophora sericioides (harvested from the Arabian Gulf) with 3 mg/L L-cysteine resulting in increasing the copper adsorption from 65 to 95% with an initial concentration of 20 mg/L copper from the wastewater. Thus, bio-treatment by microalgae becomes an attractive process because of their photosynthetic capabilities, converting solar energy into useful biomasses and mitigating eutrophication by integrating nutrients like carbon, nitrogen and phosphorus.
Oil spills bioremediation: Seawater contamination with oil spills is a serious problem that attracted the attention of many environmental organizations due to their negative effect on the marine ecosystem (Roy et al., 2014). Such a problem if not treated very fast, it will cause the death of millions of organisms in seawater (Gu et al., 2003; Sverdrup et al., 2002). This problem will be mainly a concern to oil-producing countries such as those in the Arabian Gulf region (Dashti et al., 2018), in which oil wastes input in the seawater were continuously increasing because of chronic and careless habits in the use of oil and oil products (Pashaei et al., 2015). The biogeochemical process in the seawater increases the availability of hydrocarbons to benthic organisms as well as organisms in the water column through the sediment-water interface (Perelo, 2010). Hydrocarbons consist of carcinogenic compounds like polyaromatic hydrocarbons (PAHs) and polychlorinated biphenyls (PCBs), which become a serious problem when they enter the food-chain (IARC, 1983). Therefore, bioremediation was the best technique to restore the natural environment and treat the contaminated area including water, soil and subsurface materials (Rosenberg, 1993). Bioremediation by microalgae was under concern especially in the Arabian Gulf region, and several tests were done on certain common microalgal strains to enhance their hydrocarbon degradation activity. A study was done on two microalgal species (Scenedesmus obliquus and Chlorella vulgaris) showed their biodegradation rate under heterotrophic conditions of 0.5, 1, 1.5 and 2% crude oil. The results show that S. obliquus has the highest growth rate at 0.5% crude oil, while C. vulgaris was at 2% under the same heterotrophic conditions. And both algal species were able to degrade oil effectively at low concentrations of oil (El-Sheekh et al., 2013). Also, a significant increase in C. vulgaris biomass was noticed as the crude oil concentrations increased indicating their high ability of crude oil hydrocarbons remediation. And it was noticed that approximately 94% of the light and 88% of heavy compounds were degraded by C. vulgaris in 14 days (Kalhor et al., 2017).
Microalgae can work in a symbiotic relationship with bacteria by supplying oxygen and in return up-take carbon dioxide (Oswald, 1988). Furthermore, they produce biosurfactants and extracellular enzymes to enhance bacterial biodegradation of pollutants (Muñoz et al., 2003). They also can accumulate hydrocarbons and make it available for hydrocarbon-degrading bacteria (Radwan, 2005). Microalgal species (cyanobacteria) of Synechococcus, Synechocystis, Pleurocapsa, and Dermocarpella were found in Arabian Gulf to be associated with other heterotrophic bacteria capable of degrading hydrocarbon mainly belonging to Caulobacter, Acinetobacter and Pseudomonas genera. These microalga species accumulate different hydrocarbons in their cells from the surrounding environment, thus resulting in increasing the inter-thylakoid spaces, and as a result, they will contribute to hydrocarbon pollutants removal from this water body (Al-Hasan et al., 2001). A study on hydrocarbon-degrading Marinobacter isolates supports the cyanobacteria-bacteria association, in which it was noticed that this hydrocarbon-degrading bacteria grow better and consume more oil (crude oil, n-octadecane and phenanthrene) in the presence of their cyanobacteria partner than in their absence. Thus, they play an important role in the bioremediation of oil spills in the Arabian Gulf waters (Al-Wahaib et al., 2016).
Aquaculture feed: Unicellular microalgae form the base in the food web in the marine environment and are traditionally utilized for aquacultural purposes. Recently, these unicellular organisms were introduced to the aquaculture industry in two forms, liquid paste or as a dry powder. Unicellular microalgae are carefully chosen according to their nutritional value, including high levels of protein, carotenoids, minerals, vitamins and fatty acids (Ben-Amotz et al., 2009).
The studies on microalgae in the Arabian Gulf as a feed source in aquaculture began in the late 70s (Higuchi, 1978), and commercial fish production began in Kuwait in 1992 (Bishop, 2002). James et al., (1989) tested the best local seawater microalgal species for their nutritional value with the best conditions to increase their growth and productivity to use as fish feed. First, they evaluated the nutritional quality of Chlorella and Nannochloropsis species isolated from Kuwait's seawater under different subjected temperature. It showed that Chlorella strain MFD-1 have a higher growth rate in all tested temperatures from 15 to 35°C, and a clear increase in growth rate as the temperature increases than Nannochloropsis strain MFD-2. The total amino acid composition for both strains was the highest at 30°C with similar patterns in the amino acid profile. And the total essential w3 highly unsaturated fatty acids (HUFA) content in Chlorella strain MFD-1 increased when the temperature decreases. On the other hand, the total w3 HUFA in Nannochloropsis strain MFD-2 increased when the temperature increases up to 25°C, showing that, the thermophilic strains (Chlorella) are more productive than mesophilic strains (Nannochloropsis) of algae even under controlled conditions (James et al., 1989). Then another study was conducted by James and Al-Khars (1990) to test the growth and the productivity of these 2 strains under intensive continuous culture system using tubular photobioreactors. The results showed that Nannochloropsis Strain MFD-2 is more suitable for aquacultural purposes than Chlorella Strain MFD-1 because the total w3 HUFA and the essential fatty acid eicosapentaenoic acid (EPA) content were significantly higher (P < 0.001) in the former since EPA is mandatory for the feeding of marine fish larvae. Recently, D. salina is used in shrimp and fish aquaculture in Kuwait. This species was chosen because it has the ability to thrive under Kuwait’s water harsh environment (high temperature, light intensity, and salinity) and due to its nutritional value (Abu-Rezq et al., 2010a, b). However, local halotolerant Nannochloropsis sp. was selected as a fish feed in Qatar due to its richness in various compounds that provide vital nutrition to the fish and ability to thrive under harsh environmental conditions such as high temperature, light intensity, evaporation rate and salinity (Das et al., 2015).
To enhance the production of certain shrimp species of economic value in the Arabian Gulf (Al-Harbi and Dimaano, 2010), a study on three microalgal species (D. tertiolecta, T. suecica and N. oculata) showed that D. tertiolecta was the best feed for the brine shrimp Artemia urmiana. It enhanced the length growth, survival rates and reproduction outcomes of this shrimp species rather than the other 2 tested microalgal species. The mean growth rate of the shrimp was 5.171 mm and the mean survival rate was 90% at day 8, and A. urmiana produced more cysts and nauplius when fed on D. tertiolecta with a mean of 12.87 cysts and 8.36 nauplius during the experiment period (20 days). Moreover, this was due to the high nutritional value of D. tertiolecta that comprises high lipid, carbohydrates, proteins, and β-carotene pigment (Mohebbi et al., 2015).