ABSTRACT
Microalgae are a promising alternative source to produce biofuels and bio-products, as they consume carbon dioxide to grow, multiply quickly, and can be cultivated in domestic and industrial wastewater. The efficiency of microalgae-based biodiesel industry depends on the selection of appropriate strains with best lipid content yields and adequate fatty acids composition. The aim of this study was to select a potential microalga strain for lipid production by bioprospecting and screening lipid productivity and fatty acid compositions of some local microalgae isolated from different water bodies located in the region of Agadir, Morocco and cultivated in homemade photobioreactors. For this purpose, four freshwater microalgae Parachlorella kessleri, Cyclotella species, Chlorella species and Closterium species were isolated and cultivated. Lipid extraction was carried out using Soxhlet and fatty acids profiling was performed using Gas Chromatography. The highest lipid content was obtained with a diatom microalga Cyclotella spp. (33 wt%) after 15 days of residence time. The investigated strains produced high amount of oleic acid (41-54%) followed by linoleic (18-33%), palmitic (13-23%) and stearic acid (4-12%), respectively. Variations in lipid production and fatty acids composition as responses to changes in residence times form another important factor to feedstock selection for biofuel production.
Key words: Biofuel, biomass, culture time, fatty acid, freshwater microalgae, lipid.
Biodiesel, as derived from vegetable oils or animal fats, is an alternative diesel fuel that is becoming accepted in a steadily growing number of countries around the world (Ganesan et al., 2020).
In fact, there is a huge interest in using microalgae toproduce oil for biodiesel because of their potential for high productivity in shorter time and because they hold the potential to provide a scalable renewable feedstock, without harming the food supply (Chisti, 2007). However, the production costs are still not competitive compared
to fossil fuels (Mizuno et al., 2013). Therefore, an increasing number of researches are in progress to enhance microalgae lipid production systems but there is barely any research dealing with the quality of this feedstock (Stansell et al., 2012). Since the late 1980s, biodiesel production has been increasing and standardization was a greater need than ever before. Two biodiesel standards that have served in the development of other standards around the world are the standards ASTM D6751 in the United States and EN 14214 in Europe. The development of those standards has accompanied the increasing interest in production and use of biodiesel (Knothe, 2009).
Some of the properties regulated by these two standards are difficult to be directly measured by most investigators due to the lack of necessary specialized equipment and, particularly in algal biodiesel pilot scale projects; the quantity of produced lipid is insufficient (Stansell et al., 2012). Therefore, many attempts have been undertaken to establish models that could predict some of the critical biodiesel properties from microalgae composition (Allen et al., 1999; Dunn, 2008; Imahara et al., 2006; Lapuerta et al., 2009; Lopes et al., 2008; Tong et al., 2010). These models provide preliminary assessments of the potential biomass of microalgae based on fatty acid (FA) composition (Stansell et al., 2012). These acids determine, in fact, the properties which influence the efficiency of the biodiesel feedstock such as cetane number, viscosity, cold flow, oxidation stability and lubricity (Knothe, 2009). In turn, the structural characteristics that influence the energetic properties of a FA are the length and the branching of the chain and the unsaturation degree (Knothe, 2008).
Most of reported studies support the concept that lipids rich in monounsaturated FAs (MUFAs) are suitable for biodiesel fuel, although the composition of the saturated FAs is also shown to be of great importance as well (Arguelles and Martinez-Goss, 2020). Therefore, the FAs composition should be modified to increase MUFA contents as well as enhancing lipid production.
Apparently there is a relationship between MUFA contents and algal class which potentially provides some basis for investigators to make initial selections of target classes of microalgae for bioprospecting (Stansell et al., 2012). Various studies on chemical and physical properties of biodiesel have suggested that biodiesel with a high level of MUFA may have excellent characteristics in regard to ignition quality, fuel stability, flow properties at low temperature, and iodine number (according to European biodiesel standard EN 14214) (Stansell et al., 2012; Ramos et al., 2009).
In Morocco, microalgae are less explored and further research is needed to assess the potential of these native microorganisms as a biofuel feedstock.
Recently, a study screened 57 marine microalgae isolated from the Moroccan coast to select favorable strains for biodiesel production (El Arroussi et al., 2017).
The authors concluded that, based on biomass productivity, lipid contents and FAs composition, Nannochloropsis species, Dunaliella tertiolecta, Isochrysis species and Tetraselmis species are promising species for biodiesel application (El Arroussi et al., 2017).
In the context of evaluation and bioprospecting of microalgae from local habitat, this is the first study to screen some of the freshwater microalgae from Agadir region.
In this sense, the objective of the work was to evaluate the cultivation of four microalgae strains at pilot scale. The study was aimed to examine the suitability of our homemade 25 L rectangular glass photobioreactors (PBRs) for production of lipids from the selected microalgae. Three strains from 10 microalgae isolates were selected based on some criteria like morphological differences, robustness, growth, ease of culture and harvesting. Biomass, lipid productivity and FAs composition of three selected microalgae strains (Cyclotella, Chlorella and Closterium species) were compared by using different residence times inside the PBRs.
Parachlorella kessleri, is a microalga characterized by high biomass and lipid productivity (Mizuno et al., 2013; Takeshita et al., 2014). It has been investigated for its lipid productivity at laboratory scale (Mizuno et al., 2013; Takeshita et al., 2014; Fernandes et al., 2013) and at semi-industrial outdoor condition (Li et al., 2013), and is considered an ideal microalgae species for biofuel feedstock. For all these reasons, P. kessleri was selected as a control reference in this study to design our PBRs and to optimize the culture parameter including NaHCO3 concentration and light intensity.
Field collection
Water samples were collected about the same time from different water bodies that are located in the region of Agadir, Morocco. Sampling of large bodies of fresh water occurred at multiple sites along the waterfront. Collections were made for the top and bottom of the water at each location, with the goal of determining the dominant microalgae species in each area. All field samples were collected in 50 mL tubes and maintained at refrigerated condition while transferring to laboratory
Isolation and identification of microalgae
To isolate microalgae, serial dilutions of enriched flask were performed, and 50 μL samples from 1, 2, 4, and 6 dilution fractions were inoculated to a new 500 mL flask containing 250 mL of Bold Basal Medium (BBM) (Arif et al., 2020). A volume of 50 μL from different dilutions was transferred to wells of microtiter plates that had 200 μL BBM and were kept at 22°C under continuous illumination for 3 weeks of 850 Lux with a 16/8 h light/dark cycle using T8 led tube light (G13/9W 6000 K, Brilliant, Germany) (Cobos et al., 2017). The culture’s purity was confirmed by repeated culturing and microscopic examinations.
In fact, the isolation of microalgae by serial dilution method does not necessarily ensure purity of cultures; smaller adherent heterologous microorganisms may not be detected by microscope examination.
Therefore, we have routinely tested our culture for purity by microscopic examination and if any contamination is detected, further dilutions were made. Culture purity assessments were based on conventional observations of cellular morphologies.
The morphological identification was done using botanical approaches (John et al., 2002). These approaches are based on classic morphological taxonomy, which includes the use of characteristics observable by light microscope such as shape, size, arrangement and pattern of cells.
The photobioreactor
Four glass vertical rectangular PBRs (110.0 cm in height, 15.0 cm in length, a closed system) with 24 L of working volume each (Musa et al., 2019), were developed and used for microalgae cultivation. The reactors contain three openings near the top, middle and bottom. The opening at the top was used as an exhaust for the disengaged bubbles, the bottom one was used to collect biomass, while de middle one was used for sampling. The wall thickness was 5 mm. For culture mixing, ambient air was introduced continuously with a constant air flow rate of 0.5 L/min through sterilized membrane into the bottom by a sparger fixed at the bottom of the PBR which converts the sparged air into tiny bubbles.
Fluorescent lamps were placed vertically and parallel to the front side of the PBRs with the same height as the PBR. They supply continuous illumination of 850 Lux with a 16/8 h light/dark cycle. The light intensity was measured at three fixed spots on the inside of the empty reactor using a light meter, and the average of three readings of light intensity was obtained.
Before all experiments, the PBRs were sterilized overnight with antiseptic detergent and rinsed many time with sterilized distilled water. Figure 1 shows the homemade PBRs used in this study.
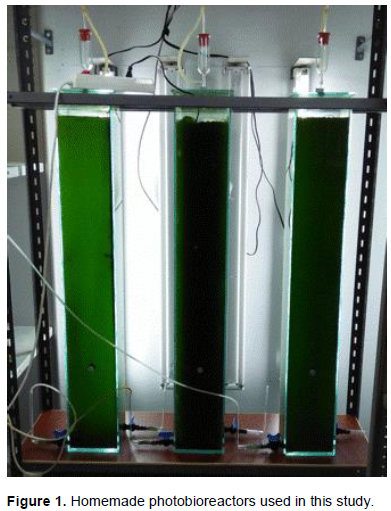
Microalgae and cultivation conditions
P. kessleri, was obtained from GEPEA UMR CNRS 6144, Saint-Nazaire, France. Cyclotella, Chlorella and Closterium spp. were collected from different locations in the region of Agadir, Morocco. Growth media for P. kessleri, Cyclotella spp. and Chlorella spp. was based on BBM (Pruvost et al., 2009). For Closterium spp., culture medium was based on Bold 3N Medium (B3NM) and formulated based on UTEX recommendation (UTEX LB 2398).
These strains were easy to cultivate in PBRs without hindrances such as cell settlement, biofilm, or floc formation.
The culture medium BBM was prepared on distilled water and was composed of (per liter) 0.05 g Na2EDTA·2H2O, 0.75 g NaNO3, 0.225 g MgSO4·7 H2O, 0.025 g CaCl2·2H2O, 0.014 g FeSO4·7H2O, 0.123 g KH2PO4, 0.15 g K2HPO4, and 1.26 g NaHCO3. Two micronutrients (1 ml each) were added to the culture medium.
The first micronutrient was composed of (per liter) 0.222 g ZnSO4·7H2O, 0.044 g Co(NO3)2·6H2O, 0.079 g CuSO4, 2.86 g H3BO3 and 1.81 g MnCl2·4H2O. The second micronutrient was composed of 0.219 g/L Na2MoO4.
The media was prepared by adding the components in order listed earlier. Each component was fully dissolved before adding the next one. The volume of 1 L of both micro-nutriments was prepared in advance and stored at 4°C.
Sodium bicarbonate was added to culture media after autoclaving the media through a 0.22 µm pore size filter.
The culture medium B3NM was prepared on distilled water and was composed of (per liter) 6 mL of P-IV metal solution, 0.75 g NaNO3, 0.025 g CaCl2·2H2O, 0.075 g MgSO4·7H2O, 0.075 g K2HPO4, 0.175 g KH2PO4 and 40 ml of soil water. Vitamin B12 (0.135 mg/L) was added after autoclaving the culture medium.
P-IV metal solution was prepared on distilled water and was composed of (per liter) 0.75 g Na2EDTA·2H2O, 0.097 g FeCl3·6H2O, 0.041 g MnCl2·4 H2O, 0.005 g ZnCl2, 0.002 g CoCl2·6H2O and 0.004 g Na2MoO4·2H2O.
A stock solution of P-IV metal was prepared and stored at 4°C prior to use. Soil water was composed of 1 tsp of Greenhouse soil, 1 mg of CaCO3 and 200 mL of distilled water. It was prepared by combining and steaming the ingredients in a cover container for two consecutive days. The solution was cooled to room temperature and stored at 4°C.
For BBM and B3NM, pH was adjusted to 6.5 before being sterilized by autoclaving at 120°C for 20 min using a pH meter (BANTE instruments, China). All glassware used in the culture of microalgae was also autoclaved.
The volume of inoculum was chosen to give an initial density of 105 cells/ml. The room temperature of 22°C was regulated by air conditioner.
Samples were taken three times a week to analyze the change in pH, cell morphology and cell number using a counting chamber (hemocytometer).
Harvesting of microalgae
After the cultivation period, the supply of air was stopped; the microalgae were distributed into 5 L flocculation vessels and were allowed to settle in the dark overnight at room temperature. The sedimentation overnight was found to be the most appropriate to settle under the influence of gravity over 90% biomass from culture. The cell-free supernatants were gently discarded using a peristaltic pump. Thereafter the microalgae were harvested through centrifugation two times during 15 min at 5000 rpm (HERMLE LABORTECHNIK, Germany), lyophilized and stored at -20°C prior to lipid extraction.
Lipids extraction using Soxhlet
Approximately 5 g of lyophilized biomass were transferred to a cellulose cartridge and placed in the extraction chamber of a 250 mL Soxhlet extractor. The extractions were performed by a period of 5 h with an average cycle time of 15 min using 300 mL of Hexane. After extraction, the solvent was recovered by rotary-evaporation. The extracted lipid was then transferred to a bottle, and kept overnight under 40°C in an air-circulating oven to evaporate any residual amount of hexane. The next day, the sample was weighed to determine the extraction yield and stored at 4°C for chemical analysis.
The lipid content was calculated in relation to the dry weight of the lyophilized microalgae.
Determination of fatty acid composition
The FA composition of the total lipids was measured by Gas Chromatography (GC) analysis of FAME. Briefly, the lipids (0.3 g) were dissolved with 20 mL of iso-octane and gentle heating in a test tube. 1 mL of potassium hydroxide solution in methanol was then added and the tube was stirred thoroughly for 30 s. To neutralize potassium hydroxide, 5 g of sodium bisulfate monohydrate was added and the solution stirred. A volume of 1.5 µL of resulting FAME was then injected to GC (Agilent Technologies 6890, USA) equipped with a BPX70 capillary column (60 m × 0.32 mm × 0.25 μm). The temperature of the injector and FID detector was 220°C. GC oven temperature was set to 175°C. GC peaks were identified by FAME standard. The relative percentage of FAs was reported based on the peak area.
Effect of residence time on biomass and lipid production
In order to determine the best residence time of the culture inside the PBR for biomass and lipid production, microalga P. kessleri selected as a control strain and three native microalgae strains were isolated and cultivated in 25 L PBR under five different residence times.
As shown in Table 1, by increasing the residence time, fresh biomass productivity increases and reach a maximum of 51.16 g/l after 45 days of culture time, whereas 15 days of culture time in PBR was sufficient to have a maximum of 18.20% of lipid content.
Although the daily fresh biomass increases slightly when the residence times pass from 15 to 30 days then to 45 days, the total harvested fresh biomass increases significantly.
Furthermore, as shown in Table 1, an increase in residence time from 5 to 15 days causes a high lipid productivity during the exponential phase.
The reason for going up to 60 days of residence time is to study the effect of nutrient starvation on lipid accumulation by P. kessleri.
The results show that the productivity of P. kessleri is high and reach 2 g/l/day during the first 5 days of culture (Table 1). A slight increase of daily fresh biomass production was observed between 5 and 45 days of culture time. After 45 days of residence time, the productivity of P. kessleri decrease to 0.38 g/l/day.
Interestingly, 15 days is an optimal residence time of P. kessleri to accumulate lipids up to 18.20% (w/w) of the dry matter. When the residence time increases from 15 to 45 days, lipid production decreases to its minimum of 1.75% (w/w). At 60 days of residence time, lipid content increases to 3.80% which may be due to the decrease of biomass production rate.
Over all the investigated strains in this study, the biomass productivity in our homemade PBRs was around 1 g/l/day.
The lipid content of Cyclotella spp. was measured as well at different residence times. Three culture times were tested and the highest lipid content was 33.06% (w/w) after 15 days in PBR (Table 2). The optimal residence time that allows P. kessleri and Cyclotella spp. to produce the maximal of lipid productivity was 15 days. For Chlorella and Closterium spp., residence time was set to 15 days and the measured lipid productivity was comparable, 2.5 and 2.62% (w/w), respectively (Table 2).
Since the residence time of 15 days was optimal for having high lipid productivity for P. kessleri and Cyclotella spp., only this residence time was tested for Chlorella and Closterium spp.
Effect of residence time on fatty acids composition
The lipid profile using Gas Chromatography revealed that the FA compositions of the four strains are similar. Their profile shows a higher amount of oleic acid followed by linoleic, palmitic and stearic acids, respectively (Tables 3 to 5).
On the other side, residence time has an effect on FA proportions, mainly between 5 and 15 days. No changes of the FA compositions were observed over 15 days of residence time.
For P. kessleri (Table 3), when the residence time increases from 5 to 15 days, the FA proportions decrease from 70.15 to 47.88% and from 13.78 to 12.65% for oleic and palmitic acids, while they increase from 12.57 to 32.81% and from 3.50 to 5.36% for linoleic and stearic acids, respectively.
For Cyclotella spp. (Table 4), when the residence time increases from 5 to 15 days, the FA amounts decrease from 28.46 to 26.69%, from 18.15 to 15.37% and from 5.91 to 4.38% for linoleic, palmitic and stearic acids, respectively, for oleic acid the FA proportion increases from 47.47 to 53.56%.
For Chlorella spp. (Table 5), the highest fatty amount is oleic acid (C18:1) 46.80% followed with palmitic acid (C16:0) 22.81%, linoleic acid (C18:2) 18.00% and stearic acid (C18:0) 12.39%.
For Closterium spp. (Table 5), the highest fatty amount is oleic acid (C18:1) 40.81% followed with linoleic acid (C18:2) 21.60%, palmitic acid (C16:0) 15.90%, linoenic acid (C18:3) 9.18%, stearic acid (C18:0) 5.29% and gadoleic acid (C20:1) 2.85%.
Microalgae are among the most promising non-food-crop-based biomass for biodiesel application (Hannon et al., 2010). However, current technology does not economically support large-scale sustainable production (Musa et al., 2019). The biofuel production process includes the upstream and downstream processing stage, with several technological limitations (Musa et al., 2019).
In order for the microalgae biotechnology to be sustainable, realizable and economically affordable, it is necessary to develop efficient culturing systems for the production of higher amount of biomass (Khan et al., 2018). Among factors that are determinant in microalgae culture are nutrients ((N, P, K), temperature, pH, salinity, inorganic carbon, oxygen, light intensity, and CO2 (Mata et al., 2010). Further factors could affect the success of cell growth such as stirring and mixing, the bioreactor geometry, culture time, and harvest repetition (Khan et al., 2018).
The lipid composition of most strains was published but mainly for cultures at the end of the stationary growth phase. It has not been studied with for different culture time.
This paper examines the effect of residence time of microalgae in PBR on lipid production and fatty acid composition, and to the best of our knowledge, this factor has never been reported on any study.
Our results show that the lipid contents and biomass productivity are inversely dependent, which is in concordance with reported studies and is associated by the amount of energy consumed as the result of lipid biosynthesis (Demirbas, 2009; Eloka-Eboka and Inambao, 2017).
The investigated microalgae, in this study, produce small amount of lipids during the first 5 days, because in the lag phase the microalgae, as reported by Jiang et al. (2012), are dividing actively, accumulating carbon in protein. In stationary phase (15 days), the reduction of available nutrients induces a gradual decrease of the cell division which causes an accumulation of cell products under lipid form.
Further, the microalgae lipids were extracted using hexane, however, using one solvent (polar or non-polar) is not potent enough to break the lipid bond with proteins, and the measured lipid content is underestimated. As reported by Halim et al. (2012), a mixture of polar solvent allows the formation of hydrogen bonds that enhance the lipid bond breaking with proteins.
Compared to our results, previous studies have reported relatively higher lipid content values using chloroform and methanol mixture as extraction solvents; 30% for P. kessleri, 30% for Chlorella spp., and 20% for Closterium spp. (Taleb et al., 2016; Karima et al. 2018). However, for Cyclotella spp., lower lipid content (25 %) has been reported using a similar mixture of extraction solvents (Graham et al., 2012).”
Of the organisms studied, lipid productivity varies greatly from one species to another and with culture time, and it seems that Cyclotella spp. strain featured, indeed, the highest lipid production among all the investigated microalgae.
Although the Soxhlet method using single solvent produces lower extraction yields than the ones obtained with a mixture of polar solvents (Li et al., 2014), it was used in this study to evaluate the efficiency of our homemade PBRs to produce biomass and to weigh out lipid content of some local microalgae strains.
It is obvious from our results that to achieve higher lipid productivity with an efficient photoconversion, the residence time of the culture inside the PBR should be optimum in a narrow range, otherwise the production decrease systematically.
Lipid compositions were also measured by changing the residence times. First of all, the dominant fatty acid in the investigated strains was oleic acid. The FA profile of P. kessleri was essentially identical after 15 days of residence time inside the PBRs. However, during growth phase (5 days), MUFA accounted for more than 70% of FAs, followed by SFA (17.28%) and PUFA (12.57%) (Table 3). It seems that there is a conversion from oleic acid to linoleic acid when residence time increases from 5 to 15 days.
In fact, oleic acid may play a role as a precursor for linoleic acid, hence growing phase convert the MUFA to a more highly unsaturated acid (Yuan and Bloch, 1961).
For Cyclotella spp. while the maximum MUFA was observed at a residence time of 15 days, the PUFA decreased with residence time (Table 4). However, SFA composition was not residence time dependent, it decreased from 24.06 to 19.75% and increased to 26.54% when residence time increased from 5 to 15 days and from 15 to 30 days.
Comparisons of fatty acid groups between Chlorella and Closterium spp. show that their MUFA compositions are essentially identical after 15 days of residence time (Table 5).
On the other hand, while Chlorella spp. produce high proportion of SFA (35.20%) than PUFA (18%), Closterium spp. contains low proportion of SFA (22.81%) than PUFA (30.78%).
Fatty acids composition of P. kessleri contains significantly higher proportions of C16:0 followed by C18:0 (Taleb et al., 2016), which is in contrast to our results where the higher FA was C18:1 followed by C18:2. Furthermore, the data in Table 6 show that in the investigated microalgae, the large amount of measured FA is generally the MUFA, which is in contrast to previous study that reported significantly higher PUFA content of the green algae (Stansell et al., 2012). In terms of MUFA content and based on Graham et al. (2012) work’s, the investigated microalgae strain, mainly Cyclotella spp., shows a good balance between most crucial fuel properties such as cetane number and cold filter plugging point (Stansell et al., 2012).
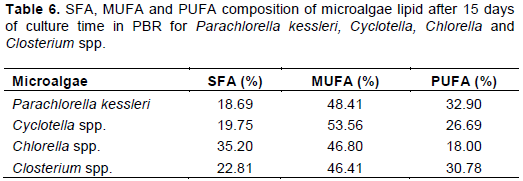
The reason for the discrepancy between reported microalgae FA compositions and our findings is the extraction procedures (Certik et al., 1996). Indeed, the polarities of solvent as well as the extraction method have an effect on either the amount of double bonds or length of carboxyl chain.
Our results are in accordance with reported study based on the polarity of individual or mixtures solvents (Certik et al., 1996). Thus less polar solvents like hexane are appropriate for the extraction of fatty acids with longer carbon chains.
While the properties of biodiesel are determined by the composition of its FAs, the properties of single FA depend on its structure such as the chain length, the number of double bonds and branch chains (Cao et al., 2014). For example, the energetic value of biodiesel increases with FA chain length, but the fluidity decreases with chain length. The longer the chain, the greater the viscosity. Therefore, in order to get good quality biodiesel, an appropriate proportion must be between MUFA, SFA and PUFA on produced microalgae lipids.
According to reported works, the perfect biodiesel would be made mostly from MUFA and have fewer PUFA and SFA, respectively (Cao et al., 2014). From our results (Table 6), Cyclotella spp. represents, somehow a promising feedstock for biodiesel production.
This study with freshwater microalgae from Agadir region in Morocco revealed that Cyclotella spp. has potential for lipid-rich biomass production. The optimum residence time in PBR for this microalga to reach the maximum lipid productivity with higher MUFA was 15 days. The cultivation and harvesting of this strain should be regulated to facilitate its use for biofuel production. Parameters including light intensity, carbon dioxide, salinity stress, temperature and nutrient starvation or limitation should be optimized to enhance microalgae lipid production. Further investigations are necessary as well to enhance lipid production and the unsaturation of fatty acids using either nutritional or environmental stresses.
As outlined in previous studies (Li et al., 2014), the fatty acids profile and productivity are also highly dependent on cultivation conditions, biomass harvesting, cell lysis and strain selection in addition to solvent polarity and extraction methods.
The variation in lipid production and fatty acids profile as a result of changes in residence time represents an important factor to microalgae strain selection and should provide suggestions for further research.
The authors have not declared any conflict of interests.
REFERENCES
Allen CAW, Watts KC, Ackman RG, Pegg MJ (1999). Predicting the viscosity of biodiesel fuels from their fatty acid ester composition. Fuel 78:1319-1326.
Crossref
|
|
Arguelles ED, Martinez-Goss MR (2020). Lipid accumulation and profiling in microalgae Chlorolobion sp. (BIOTECH 4031) and Chlorella sp. (BIOTECH 4026) during nitrogen starvation for biodiesel production. Journal of Applied Phycology.
Crossref
|
|
|
Arif M, Wang L, Salama ES, Hussain MS, Li X, Jalalah M, Al-Assiri MS, Harraz FA, Ji MK, Liu P (2020). Microalgae Isolation for Nutrient Removal Assessment and Biodiesel Production. BioEnergy Research 2020:1-13.
Crossref
|
|
|
Cao Y, Liu W, Xu X, Zhang H, Wang J and Xian M (2014). Production of free monounsaturated fatty acids by metabolically engineered Escherichia coli. Biotechnology for Biofuels 7:59-69.
Crossref
|
|
|
Certik M, Andrasi P, Sajbidor J (1996). Effect of Extraction Methods on Lipid Yield and Fatty Acid Composition of Lipid Classes Containing y-Linolenic Acid Extracted from Fungi. Journal of the American Oil Chemists' Society 73:357-365.
Crossref
|
|
|
Chisti Y (2007). Biodiesel from microalgae. Biotechnology Advances 25:294-306.
Crossref
|
|
|
Cobos M, Paredes JD, Maddox JD, Vargas-Arana G, Flores L, Aguilar CP, Marapara JL, Castro JC (2017). Isolation and Characterization of Native Microalgae from the Peruvian Amazon with Potential for Biodiesel Production. Energies 10:224-239.
Crossref
|
|
|
Demirbas A (2009). Progress and recent trends in biodiesel fuels. Energy Conversion and Management 50:14-34.
Crossref
|
|
|
Dunn RO (2008). Crystallization behavior of fatty acid methyl esters. Journal of the American Oil Chemists' Society 85:961-972.
Crossref
|
|
|
El Arroussi H, Benhima R, El Mernissi N, Bouhfid R, Tilsaghani C, Bennis I, Wahby I (2017). Screening of marine microalgae strains from Moroccan coasts for biodiesel production. Renewable Energy 113:1515-1522.
Crossref
|
|
|
Eloka-Eboka AC, Inambao FL (2017). Effects of CO2 sequestration on lipid and biomass productivity in microalgal biomass production. Applied Energy 195:1100-1111.
Crossref
|
|
|
Fernandes B, Teixeira J, Dragone G, Vicente AA, Kawano S, Bišová K, PÅ™ibyl P, Zachleder V, Vítová M (2013). Relationship between starch and lipid accumulation induced by nutrient depletion and replenishment in the microalga Parachlorella kessleri. Bioresource Technology 144:268-274.
Crossref
|
|
|
Ganesan R, Manigandan S, Samuel MS, Shanmuganathan R, Brindhadevi K, Lan Chi NT, Anh Duc P, Pugazhendhi A (2020). A review on prospective production of biofuel from microalgae. Biotechnology Reports 27:e00509.
Crossref
|
|
|
Graham JM, Graham LE, ZulkiXy SB, PXeger BF, Hoover SW, Yoshitani J (2012). Freshwater diatoms as a source of lipids for biofuels, Journal of Industrial Microbiology and Biotechnology 39:419-428.
Crossref
|
|
|
Halim R, Danquah MK, Webley PA (2012). Extraction from oil from microalgae for biodiesel production: A Review. Biotechnology Advances 30:709-732.
Crossref
|
|
|
Hannon M, Gimpel J, Tran M, Rasala B, Mayfield S (2010). Biofuels from algae: challenges and potential. Biofuels 1:763-784.
Crossref
|
|
|
Imahara H, Minami E, Saka S (2006). Thermodynamic study on cloud point of biodiesel with its fatty acid composition. Fuel 85:1666-1670.
Crossref
|
|
|
Jiang Y, Yoshida T, Quigg A (2012). Photosynthetic performance, lipid production and biomass composition in response to nitrogen limitation in marine microalgae. Plant Physiology and Biochemistry 54:70-77.
Crossref
|
|
|
John DM, Whitton BA, Alan J, Brook AJ (2002). The freshwater algal flora of the British isles. An identification guide to freshwater and terrestrial algae. Cambridge University Press.
|
|
|
Karima A, Silalahi MDS, Rinanti A (2018). Increasing content of lipid in tropical microalgae Chlorella sorokiniana and Closterium sp. with variation of nitrogen content and extraction temperature. MATEC Web of Conferences 197.
Crossref
|
|
|
Khan MI, Shin JH, Kim JD (2018). The promising future of microalgae: current status, challenges, and optimization of a sustainable and renewable industry for biofuels, feed, and other products. Microbial Cell Factories 17(1):36.
Crossref
|
|
|
Knothe G (2008). "Designer" biodiesel: optimizing fatty ester composition to improve fuel properties. Energy Fuels 22:1358-1364.
Crossref
|
|
|
Knothe G (2009). Improving biodiesel fuel properties by modifying fatty ester composition. Energy and Environnemental Science 2:759-766.
Crossref
|
|
|
Lapuerta M, Rodrıguez-Fernandez J, Font de Mora E (2009). Correlation for the estimation of the cetane number of biodiesel fuels and implications on the iodine number. Energy Policy 37:4337-4344.
Crossref
|
|
|
Li Y, Naghdi FG, Garg S, Adarme-Vega TC, Thurecht KJ, Abdul Ghafor W, Tannock S, Schenk PM (2014). A comparative study: the impact of different lipid extraction methods on current microalgal lipid research. Microbial Cell Factories 13:14.
Crossref
|
|
|
Li X, PÅ™ibyl P, Bišová K, Kawano S, Cepák V, Zachleder V, ÄŒížková M, Brányiková I, Vítová M (2013). The microalga Parachlorella kessleri - a novel highly efficient lipid producer. Biotechnology and Bioengineering 110:97-107.
Crossref
|
|
|
Lopes JCA, Boros L, Krähenbühl MA, Meirelles AJA, Daridon JL, Pauly J, Marrucho IM, Coutinho JAP (2008). Prediction of cloud points of biodiesel. Energy Fuels 22:747-752.
Crossref
|
|
|
Mata TM, Martins AA, Caetano NS (2010). Microalgae for biodiesel production and other applications: a review. Renewable and Sustainable Energy Reviews14:217-232.
Crossref
|
|
|
Mizuno Y, Sato A, Watanabe K, Hirata A, Takeshita T, Ota S, Sato N, Zachleder V, Tsuzuki M, Kawano S (2013). Sequential accumulation of starch and lipid induced by sulfur deficiency in Chlorella and Parachlorella species. Bioresource Technology 129:150-155.
Crossref
|
|
|
Musa M, Ayoko GA, Ward A, Rösch C, Brown RJ, Rainey TJ (2019). Factors Affecting Microalgae Production for Biofuels and the Potentials of Chemometric Methods in Assessing and Optimizing Productivity. Cells 8:851-874.
Crossref
|
|
|
Pruvost J, Van Vooren G, Cogne G, Legrand J (2009). Investigation of biomass and lipids production with Neochloris oleoabundans in photobioreactor. Bioresource Technology 100:5988-5995.
Crossref
|
|
|
Ramos MJ, Fernandez CM, Casas A, Rodriguez L, Perez A (2009). Influence of fatty acid composition of raw materials on biodiesel properties. Bioresource Technology 100(1):261-268.
Crossref
|
|
|
Stansell GR, Gray VM, Sym SD (2012). Microalgal fatty acid composition: implications for biodiesel quality. Journal of Applied Phycology 24:791-801.
Crossref
|
|
|
Takeshita T, Ota S, Yamazaki T, Hirata A, Zachleder V, Kawano S (2014). Starch and lipid accumulation in eight strains of six Chlorella species under comparatively high light intensity and aeration culture conditions. Bioresource Technology 158:127-34.
Crossref
|
|
|
Taleb A, Kandilian R, Touchard R, Montalescot V, Rinaldi T, Taha S, Takache H, Marchal L, Legrand J, Pruvost J (2016). Screening of freshwater and seawater microalgae strains in fully controlled photobioreactors for biodiesel production. Bioresource Technology 218:480-490.
Crossref
|
|
|
Tong D, Hu C, Jiang K, Li Y (2010). Cetane number prediction of biodiesel from the composition of the fatty acid methyl esters. Journal of the American Oil Chemists' Society 88:415-423.
Crossref
|
|
|
Yuan C, Bloch K (1961). Conversion of Oleic Acid to Linoleic Acid, Journal of Biological Chemistry 236:1277-1279.
|
|