ABSTRACT
Microbial diseases remain to be a major global public health challenge. Their devastating effects have been exacerbated by the development of drug resistant strains. On the other hand, the biodiversity of tropical Basidiomycetes fungi is well recognized as an untapped source of potential bioactive compounds for the development of novel antimicrobials. Thus, in the current study, Basidiomycetes’ fruiting bodies were collected from Mt. Elgon National Park forest in Kenya. The spores were cultured on Yeast Malt Agar media (YMG), leading to the establishment of 12 distinct pure fungal cultures of Basidiomycete strains, which were characterized using ribosomal internal transcribed spacer (ITS) DNA. They generally belonged to genera; Inonotus, Fomitiporia, Ganoderma, Skeletocutis, Perenniporia, Favolaschia, Hexagonia, Polyporus, Antrodia and Echinochaete. Fungal mycelia were further fermented in YMG, Q6½ (cotton-seed) and ZM½ (sugar-malt) liquid media for secondary metabolites’ production. These were extracted using ethyl-acetate and subjected to antimicrobial assays against Bacillus subtilis, Escherichia coli, Mucor plumbeus and Candida albicans. Antimicrobial activity was exhibited in 9 out of the 12 strains cultured, where antibacterial activity was more pronounced than fungal antagonism. Mycelial crude extracts from strains identified as Skeletocutis nivea and Favolaschia calocera demonstrated the highest activities against bacteria (B. subtilis) and fungal pathogen (C. tenuis), respectively with minimum inhibitory concentration (MIC) values of 4.69 µg/ml and <2.34 µg/ml, as compared to ciprofloxacin and nystatin controls which exhibited MIC values of <2.34 µg/ml each. In addition, crude extracts from Hexagonia sp and Inonotus pachyphloeus inhibited growth of E. coli at 300 µg/ml each, while M. plumbeus growth was inhibited by extracts from F. calocera and S. nivea at 37.5 and 300 µg/ml, respectively. These results clearly demonstrate that Basidiomycetes are a reservoir to antimicrobial fungal metabolites, which can be exploited as lead compounds to address drug resistance menace.
Key words: Drug resistance, basidiomycetes, antimicrobial, fungal metabolites.
Infectious diseases pose a huge social and economic burden in the developing countries, where they are responsible for one in every two deaths (WHO, 2014). The problem of infectious diseases has been
exacerbated by development of drug resistant fungal and bacterial pathogens (Danishuddin et al., 2012; Ling et al., 2015). As a result, there has been a recent rise in the total health care costs associated with the need for multidrug therapy, longer and more frequent hospital stays, as well as increased costs of research and development for alternative drugs in microbial disease management (Ventola, 2015).
The fungal kingdom forms the second most diverse group of organisms in the universe. However, only a very small fraction of about 100,000 has been described so far and explored for the production of bioactive compounds (Blackwell, 2011). Nevertheless, fungi are known to accumulate antibacterial and antifungal secondary metabolites in order to survive the hostility of their natural environment. Thus, some of the major successful antimicrobial drugs currently in the market were developed from fungal secondary metabolites. These include antibiotics namely penicillins, cephalosporins and fusidic acid as well as antifungal agents griseofulvin, strobilurins and echinocandins (De Silva et al., 2013; Kozlovskii et al., 2013).
Mushroom forming fungi mostly from phylum Basidiomycota have been used as remedies for various diseases traditionally, owing to their prolific production of secondary metabolites (De Silva et al., 2012). However, macro fungi of the phylum Basidiomycota, are less intensively investigated targets despite the fact that they contain compounds with potential antimicrobial activities. Novel compounds of different biogenetic origins isolated from Basidiomycota have been shown to have antibacterial and antifungal properties among other pharmacological activities (De Silva et al., 2012; Wasser 2011). Hence, these mushrooms make a vast yet untapped source of new antimicrobials.
In the present study, we screened pure cultures of phenotypically diverse Basidiomycetes fungal strains for antimicrobial compounds production, as a first step towards isolation of active or potential leads for the development of novel antimicrobial drug compounds that can offer an alternative to microbial disease management without the negative impact of synthetic drugs.
Fungal material preparation
Basidiocarps were collected from Mt. Elgon National Park forest in Kenya, located at 1.1635°N, 34.5930°E. A piece (7 mm) of the cap was sliced and stuck on the lid of the petri dish and the left overnight for the spores to fall into the YMG (Yeast-Malt-Medium) media (10 g/L malt extract, 4 g/L glucose, 4g/L yeast extract, 20 g/L agar and pH 6.3) amended with streptomycin sulphate (1.5 mg/ml) antibiotic. Sub-culturing of fungal mycelia into fresh media without antibiotics was thereafter performed to establish axenic cultures.
DNA extraction and amplification
Genomic DNA was extracted from the mycelia obtained from axenic cultures using EZ-10 Spin Column Fungal Genomic DNA Mini-Preps kit (BIO BASIC INC.), following the manufacturer’s instructions. The nuclear ribosomal DNA barcoding region Internal Transcribed Spacer (ITS), was amplified using the ITS1F (CTTGGTCATTTAGAGGAAGTAA) and ITS4 (TCCTCCGCTTATT GATATGC) forward and reverse primers, respectively (Gardes and Bruns, 1993; White et al., 1990). The amplification was conducted using a 25 µl polymerase chain reaction (PCR) reaction mix consisting of 2 µl (0.5 µg) fungi genomic DNA and 23 µl PCR master mix (12.5 µl JumpStart Taq Ready Mix (Sigma-Aldrich), 9.5 µl water, 0.5 µl (10 pmol) ITS1-F and ITS4 primers. The amplification was conducted using Eppendorf Mastercycler under the following program cycle; initial denaturation at 94°C for 5 min, followed by 34 cycles of denaturation, annealing and elongation for 2 min, at 94, 52 and 72°C, respectively and final extension at 72°C for 10 min. To confirm successful PCR amplification and the quality of ITS rDNA amplicons, 3 µl aliquots of PCR products were mixed with 2 µl Midori green loading dye and resolved on 0.8% agarose gel. Gel electrophoresis was conducted at 100v for 30 min in 1×TAE buffer and the gel viewed under non-hazardous Nippon Genetics White light LED trans-illuminator.
DNA sequencing and phylogenetic analysis
The PCR amplified ITS fragments were purified using EZ-10 Spin Column PCR product purification kit (BIO BASIC INC.) following the manufacturer’s instruction. These were then bidirectional-sequenced using Sanger technique. The consensus sequences were assembled in Geneious software version 11.0.4 (Kearse et al., 2012) and subjected to BLASTN search on the National Centre for Biotechnology Information (NCBI)-GenBank database (https://blast.ncbi.nlm.nih.gov/genbank/) to determine the identity of the axenic fungal strains. The evolutionary relatedness of the fungi, was established by multiple sequence alignment of the sequenced ITS rDNA fragments using Clustal X version 2.0 (Larkin et al., 2007) and sequence edition using Jalview version 2 (Waterhouse et al., 2009). A phylogenetic tree based on neighbor joining method with 1000 bootstrap replications, was then constructed using Geneious Tree Builder plugin implemented in Geneious software as described by Kearse et al. (2012), and viewed using iTOL (Interactive Tree of Life) version 3.6.1 online tool (http://itol.embl.de/) (Letunic and Bork, 2016).
Fermentation and extraction of fungal metabolites
The axenic fungi cultures were cultivated separately in liquid media for production of antimicrobial secondary metabolites based on the procedure described by Stadler et al. (2003). The cultivation media were YMG (without agar), ZM½ (Sugar-Malt-Medium; 5 g/L molasses, 5 g/L oatmeal, 4 g/L saccharose, 4 g/L mannitol, 1.5 g/L glucose, 1.5 g/L calcium carbonate, 0.5 g/L Edamin, 0.5 g/L ammonium sulphate and pH 7.2) and Q6½ (Cotton Seed Flour-Medium; 2.5 g/L glucose, 10 g/L glycerin, 5 g/L cotton seed flour and pH 7.2). The liquid media were autoclaved for 30 min at 121°C and 15 pa. For each axenic fungi culture, 5 mycelial fungal plugs (7 mm) were transferred into 500 ml Erlenmeyer flasks containing 200 ml of cultivation media and then propagated in a rotary shaker at 23.8°C and 140 rpm in the dark. Glucose exhaustion was tested using sterile glucose strips after every two days and the fungal metabolites harvested 3 days after glucose depletion.
Extraction of fungal metabolites was achieved by initial separation of the mycelial and supernatant (culture media) portions by filtration. The supernatant portion was then extracted using an equal volume of ethyl acetate solvent and filtered through anhydrous sodium sulphate. The resultant supernatant ethyl acetate extract was dried using a rotary evaporator and its weight determined.
The mycelial portion was also extracted with an equal volume of acetone in an ultrasonic bath for 30 min and the solvent removed in a rotary evaporator. The remaining portion was then partitioned with an equal amount of ethyl acetate and extracted in a similar manner to the supernatant portion to afford a dry ethyl acetate mycelial extract.
Antimicrobial activity determination using the serial dilution assay method
The minimum inhibitory concentration (MIC) was then determined for the supernatant and mycelial ethyl acetate crude extracts using the serial dilution assay method as described by Halecker et al. (2014) and Okanya et al., (2011), against selected microbial test pathogens. Fungal test pathogens used were M. plumbeus (filamentous fungi) MUCL 49355 and C. tenuis (yeast cells) MUCL 29982, whereas the bacterial test organisms used were B. subtilis (Gram-positive) DSM10 and E. coli (Gram-negative) DSM498. The serial dilution assays were conducted using 96-well microtiter plates, using liquid EBS (0.5% casein peptone, 0.5% glucose, 0.1% meat extract, 0.1% yeast extract, 50 mM (11.9 g/L) HEPES, pH 7.0) and YMG media for bacterial and fungal test organisms, respectively. Bürker Neubauer counting chamber was used to establish a microbial working cell concentration of 105 CFUs/ml. A multichannel pipette was then used to pipette 150 µl aliquots of microbial pathogen-media mixture into 96-well microtiter plates. An additional 130 µl pathogen-media mixture was added to the first row (A1-A12) of the assay plate. Then, 20 µl of the test samples (4.5 mg/ml) dissolved in methanol were loaded to the wells of the first rows, with the last two wells loaded with 20 µl of the negative (methanol) and positive controls. The positive controls used were 1mg/ml nystatin for fungal test organisms and 1mg/ml ciprofloxacin for bacterial pathogens. The contents of the first row (A) were then mixed using a multichannel pipette and 150 µl transferred to the adjacent row (B). Further, a 1:1 serial dilution was performed in subsequent rows (C-H) and 150 µl discarded after the last row (H). Hence, a decreasing extract and antibiotic concentration in the range of 300 µg/ml to 2.34 µg/ml and 100 µg/ml to 0.78 µg/ml from A-H, respectively were screened. The 96 well microtiter plate was then incubated at 30° C in a microplate-vibrating shaker (Heidolph Titramax 1000) at 450 rpm. The plates were checked for inhibition after 24 hours for bacteria and 48 hours for fungal test organisms and the MIC determined.
Fungal identification
Basidiomycete fruiting bodies collected had similar morphological characteristics with regards to sporocarp colour and shape with exception of a few (Supplementary Material 1). Out of 14 morphologically distinct fruiting bodies collected, axenic cultures were successfully prepared from 12 fungi following sliced sporocarp culture on YMG media. The 12 cultures differed in their cultural growth rates, ranging from 15 to 70 days to attain full-plate growth. Generally, the colour of mycelia cultures growing on YMG media was either white, cream, brown, or a combination of the colors (Supplementary Material 2).
PCR amplification of genomic DNA obtained from the 12 fungi using ITS1F and ITS4 primers, produced expected amplicons of between 500-700bp. The PCR amplicons (ITS) were sequenced and their sizes ranged between 527 and 688 nucleotides long (Supplementary Material 3). Alignment using Clustal X revealed great variation of the rDNA ITS region nucleotide residues. Furthermore, BLASTN analysis of the sequences revealed that all the strains were from phylum/division Basidiomycota and class Agaricomycetes (Table 1). The identities of the 12 strains were based on the best matches with sequence similarity of ≥ 97-100%, query coverage ≥ 80% and e-values of zero. However, the best matches for strains KE/16-101 corresponding to Inonotus pachyphloeus and KE/16-198 to Echinochaete brachypora, had lower query coverages of 76 and 74%, respectively. Generally, BLAST search revealed that the fungi collected from Mt. Elgon were of the genera; Skeletocutis, Perenniporia, Echinochaete, Ganoderma, Hexagonia, Fomitiporia, Antrodia, Favolaschia, Inonotus and Polyporus (Table 1).
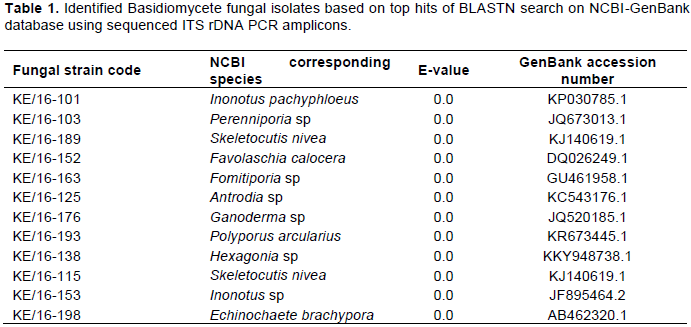
The evolutionary history of the 12 study sequences and 58 related sequences obtained from GenBank inferred using Neighbor Joining (NJ) method, confirmed that three taxonomic orders were represented by the 12 fungi strains collected from Mt. Elgon forest. These were; Hymenochaetales, Polyporales and Agaricales. The tree also resolved into various fungal families including; Hymenochaetaceae (Inonotus sp and Fomitiporia aethiopica), Polyporaceae (Polyporus arcularius, Hexagonia sp, Ganoderma sp, Skeletocutis nivea), Mycenaceae (Favolaschia calocera) and Fomitopsidaceae (Antrodia sp) (Figure 1).
MIC determination using fungal crude extracts
MIC assay against B. subtilis and E. coli
In the MIC assay, antimicrobial activities of secondary metabolites extracted from mycelium and supernatant was observed in 9 out of the 12 fungal strains. Secondary metabolites from KE/16-175, KE/16-103 and KE/16-153 did not show any antimicrobial activities against all the tested microorganisms. However, irrespective of the source (mycelium or supernatant), secondary metabolites extracted from strains KE/16-101, KE/16-198, KE/16-163, KE/16-125, KE/16-138, KE/16-189 and KE/16-115 fermented in Q6½ and YMG media were active against B. subtilis (Table 2). Notably, the mycelial extracts from KE/16-189 and KE/16-198 strains fermented in Q6½ medium demonstrated the highest inhibitory activities at concentrations of 4.69 and 9.38 µg/ml, respectively. The supernatant extracts from these strains demonstrated inhibitory activities against B. subtilis at relatively lower concentrations of 9.38 and 18.75 µg/ml for KE/16-198. In addition, extracts from the strain KE/16-125 strain fermented in Q6½ medium demonstrated antimicrobial activity at 37.5 µg/ml (mycelial extract) and 300 µg/ml (supernatant extracts) against B. subtilis. Interestingly, for the strain KE/16-101, only the mycelial extracts inhibited growth of B. subtilis at higher concentration of 300 µg/ml.
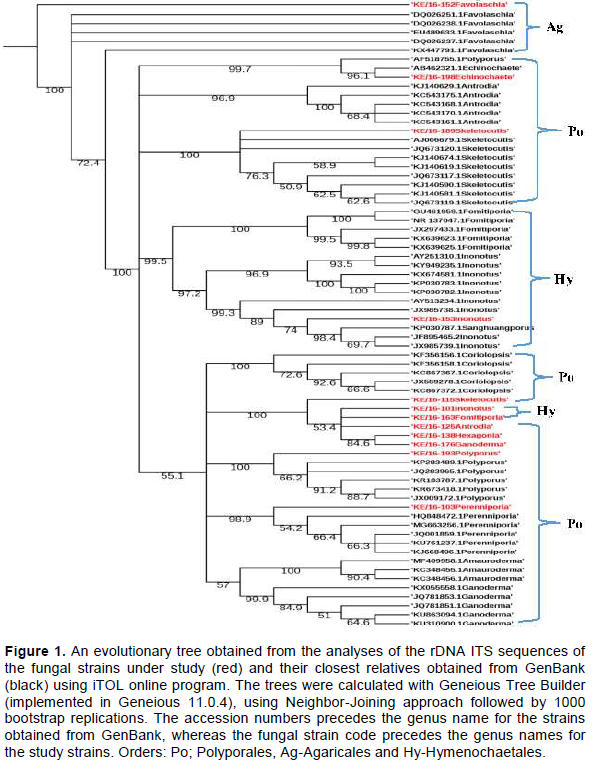
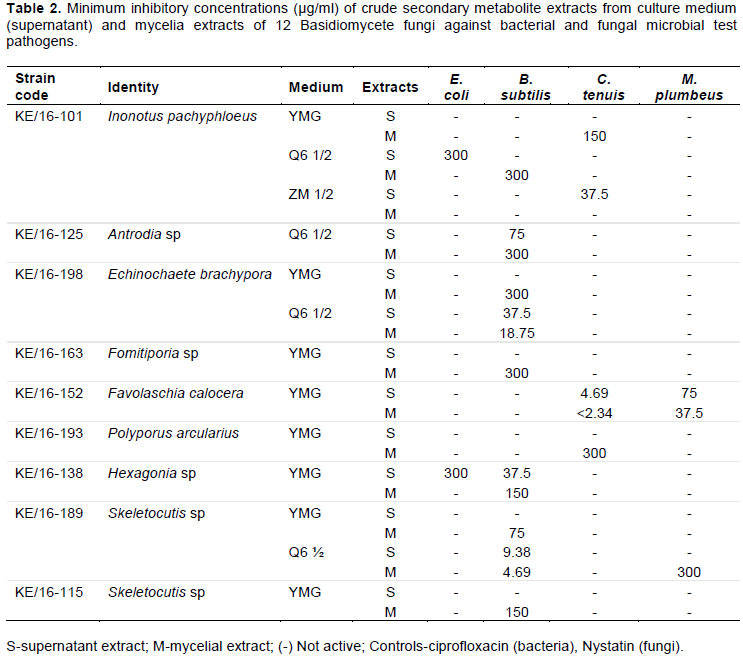
Generally, mycelial extracts from fungi strains fermented in YMG medium were more active against B. subtilis than from supernatant. The mycelial extracts obtained from strains KE/16-189, KE/16-138, KE/16-163 and KE/16-198 showed activity at MIC of 75, 150 and 300 µg/ml, respectively against the B. subtilis. However, only the YMG supernatant extracts from strain KE/16-138 demonstrated minimum inhibitory activity against the B. subtilis at 37.5 µg/ml. The positive control (ciprofloxacin) gave an MIC value of <2.34 µg/ml in all the tests that were performed against B. subtilis. Similar to B. subtilis assay, secondary metabolites extracted from fungi fermented in YMG and Q6½ media showed activity against E. coli. However, unlike the former where the mycelial and supernatant extracts were active, only the supernatant extracts obtained from KE/16-138 and KE/16-101 demonstrated inhibitions against E. coli at 300 µg/ml. The positive control (ciprofloxacin) gave an MIC value of <2.34 µg/ml in all the tests that were performed against E. coli (Table 2).
MIC assay against M. plumbeus and C. tenuis
M. plumbeus and C. tenuis growth was inhibited by secondary metabolites fermented using Q6 ½, YMG andZM ½ media. Notably, the mycelial and supernatant extracts from KE/16-152 fermented in YMG media demonstrated very low MIC values of <2.34 and 9.38 µg/ml against C. tenuis, respectively, unlike for M. plumbeus where higher MIC values of 150 µg/ml and 75 µg/ml were obtained for mycelial and supernatant extracts, respectively. The ZM ½ supernatant extracts from strain KE/16-101 also demonstrated a remarkable low MIC value of 37.5 µg/ml against C. tenuis, whereas the strain’s YMG mycelial extracts showed activity against C. tenuis at 150 µg/ml. In addition, YMG mycelial and supernatant extracts from strain KE/16-193 and Q6 ½ mycelial extract from KE/16-189 demonstrated mild activities at 300 µg/ml against C. tenuis and M. plumbeus, respectively. The positive control, nystatin produced MIC values of <2.34 µg/ml for C. tenuis and 18.75 µg/ml for M. plumbeus (Table 2).
Fungal identification
Morphological-based identification of fungi species for a long time has been the basis of fungal taxonomy. However, during collection and culturing of axenic Basidiomycetes fungi, phenotypic characteristics could not be relied upon due to similarities in cap colors and shapes as well as cultural characteristics. Therefore, the more reliable molecular identification techniques based on sequencing of the ITS1-5.8S-ITS2 rDNA region flanked by ITS1F and ITS4 primers was used. The ITS rDNA reliability and effectiveness in Basidiomycetes’ identification had earlier been demonstrated by various studies (Schoch et al., 2012; Pryce et al., 2003). Therefore, the successful amplification of the respective ITS regions clearly demonstrated the efficiency of ITS1F and ITS4 primers in identification of fungi belonging to Basidiomycetes group, this is in line with earlier reports by Blaalid et al. (2013). In addition, other studies have also reported that ITS1 and ITS5 primer pairs are also biased towards the identification of Basidiomycetes, while pairing primers ITS2 or ITS3 with ITS4 have preferentially identified Ascomycetes (Bellemain et al., 2010).
Comparing the sequenced ITS rDNA fragments using CLUSTAL/W confirmed that
the 12 axenic fungi cultures were indeed different strains, since there was no full sequence
similarity albeit a few short sequences. The BLASTN search tool used after fungal ITS rDNA sequencing aided in the successful identification of 6 out of the 12 fungal strains namely; KE/16-103 (Perenniporia sp), KE/16-153 (Inonotus sp), KE/16-176 (Ganoderma sp), KE/16-138 (Hexagonia sp), KE/16-125 (Antrodia sp) and KE/16-163 (Fomitiporia sp) to the genus level. Similarly, it revealed the identity of only 6 cultured strains to the species level (KE/16-198 (Echinochaete brachypora), KE/16-193 (P. arcularius), KE/16-189 (Skeletocutis nivea), KE/16-115 (Skeletocutis nivea), KE/16-152 (Favolaschia calocera) and KE/16-101 (Inonotus pachyphloeus)). The use of BLASTN search tool in identification of the axenic fungi collected from Mt Elgon exhibited drawbacks, and this could be due to databases limited taxonomic scope for the available rDNA ITS sequences. In addition, fungal databases have also been reported to contain inaccurately identified sequences (Ko et al., 2011). For these reasons, there are documented examples of failure of BLAST hits reliability in fungi identification, hence leading to wrong conclusions (Christen, 2008). The NJ method used in deducing the evolutionary relationship of the axenic fungi generated a well resolved tree. The tree revealed that all the axenic fungal strains cultured belonged to phylum Basidiomycota. Furthermore, it also revealed that they can be grouped into 3 Orders namely; Hymenochaetales (KE/16-101-Inonotus pachypholeus, KE/16-153-Inonotus sp and KE/16-163-Fomitiporia sp), Polyporales (KE/16-125-Antrodia sp, KE/16-138-Hexagonia sp, KE/16-198-Echinochaete brachypora, KE/16-175-Ganoderma sp, KE/16-193 P. arcularius, KE/16-103-Perenniporia sp and Skeletocutis nivea (KE/16-115, KE/16-189) and Agaricales (KE/16-152-Favolaschia calocera) (Figure 1). Interestingly, similar taxa (Orders) had been reported previously in Eastern Africa (Decock and Bitew, 2012; Decock et al., 2005; Wagner and Fischer, 2002; Ryverden and Johansen, 1980). Despite the fact that strains KE/16-115 and KE/16-189 could not be distinguished morphologically, whereas BLAST search associated them to S. nivea, phylogenetic analyses revealed that they were different species (Figure 1). S. nivea has not been reported in Africa unlike in South America, Europe and Asia (Robledo and Rajchenberg, 2007). The use of the rDNA ITS region in identification of the fungal strains is not also devoid of short-comings due to the high variability among the ITS sequences, however, it has been useful in separation of species (Lindahl et al., 2013). For instance, in our study KE/16-115 and KE/16-189 belonging to the same genus (Skeletocutis), and non-distinguishable due to their similar morphological characteristics did not cluster together on the phylogram (Figure 1). This clearly demonstrates that they are likely to be two distinct species of the same genus. However, the rDNA ITS region is too variable to cater for evolutionary relatedness at higher taxonomic ranks such as orders and families (Lindahl et al., 2013). Therefore, this probably explains why, it was difficult to attain perfect alignment of sequences at the family level of taxonomy for some strains; that is, KE/16-101 and KE/16-163 associated with Inonotus sp and Fomitiporia sp respectively which belong to Hymenochaetaceae family, as well as KE/16-125 identified as Antrodia sp (Fomitopsidaceae), which instead clustered on the phylogram with fungi of family Polyporaceae (Figure 1). Nevertheless, there are reports suggesting that the use of nuclear large subunit (LSU) rDNA primers could be a better alternative due to its resolution at higher levels of taxonomy compared to ITS (Porter and Golding, 2012).
Antimicrobial assays
Antimicrobial activities against bacteria were demonstrated by fungi strains associated with genera Hexagonia, Skeletocutis, Echinochaete, Inonotus, Antrodia and Fomitiporia after BLASTN search on GenBank database (Table 2). In addition, fungal test organisms were also inhibited by the extracts from strains identified as Favolaschia calocera, Inonotus pachyphloeus, S. nivea and P. arcularius. The variation of antagonism per growth medium formulation and source either mycelia or supernatant was evident, suggesting that the production of bioactive secondary metabolites to some extent was influenced by various growth media. The variation of culture media contents has been shown to have significant impact on the diversity and quantity of bioactive fungal compounds (Pu et al., 2013) and thus in line with the diverse antimicrobial activities as observed in this study.
Fungi strain (KE/16-189 and KE/16-198) which were identified as S. nivea and Echinochaete brachypora seemed to produce secondary metabolites that inhibit Gram positive bacteria (B. subtilis), as demonstrated by their low MIC values of 4.69 and 18.75 µg/ml, respectively, for mycelia cultured in Q6½ as compared with ciprofloxacin positive control (<2.34 µg/ml). E. brachypora was taxonomically described by Ryvarden and Johansen (1980), however, there are no previous reports on the bioactivities of the strain. Ethyl acetate extracts from KE/16-138 cultured in YMG media were also highly active against B. subtilis. Similarly, a study conducted by Rosa et al. (2003), reported a high growth inhibition of B. cereus by Hexagonia hynoides. Interestingly, in the present study, the supernatant extracts were more active compared to the mycelial extracts, suggesting that the secondary metabolites secreted into the growth media during fermentation contained more potent active compounds. In fact, only the supernatant extracts of KE/16-138 inhibited E. coli in this study (Table 2). In addition a study by Al-Fatimi et al. (2013), also demonstrated that Hexagonia velutina had a considerable higher antifungal activity against Trichophyton mentagrophytes than Nystatin reference antibiotic. However, in the present study KE/16-138 did not demonstrate any antifungal activity.
Antrodia daedaleiformis was first reported in East Africa by Ryvarden and Johansen (1980). However extensive published works have only been performed on Antrodia camphorata which is predominantly used as medicine in Asia (Geethangili and Tzeng, 2011). In the present study, supernatant and mycelial ethyl acetate extracts from KE/16-125 belonging to genus Antrodia in Q6½ media showed activity against the gram positive B. subtilis. The antimicrobial activities of the fungal genus have been attributed to presence of terpenoid compounds predominant in the fruiting bodies and mycelial cultures (Geethangili and Tzeng, 2011). On the other hand, Fomitiporia aethiopica was first reported in the Ethiopian highlands and described by Decock et al. (2005). The secondary metabolites isolated from KE/16-163-Fomitiporia sp exhibited moderate antibacterial activity against B. subtilis, in particular YMG mycelial extracts, although there is no previous documented research on the bioactivities of the genus. Nevertheless, various studies have reported the association of some species of genus Fomitiporia with Esca disease of the grapevine (Graniti, 2006; Fischer et al., 2005).
KE/16-152 identified as Favolaschia calocera demonstrated the highest antifungal activities against both filamentous fungi (<2.34 µg/ml) and yeast cells (9.38 µg/ml) (Table 2). Similar results were reported for F. calocera from a previous study by Chepkirui et al., (2016). The strong antifungal activity had previously been attributed to presence of four oxostrobilurins derivatives obtained from the YMG mycelial extracts. However, in the current study, both the supernatant and mycelial extracts of YMG media showed activity against the fungal test pathogens. In addition, mild antifungal activity was shown against the C. tenuis by KE/16-193 identified as P. arcularius in the current study. This is in line with an earlier report indicating that crude extracts isolated from a fungus belonging to genus Polyporus, have been used in treatment of various ailments such as urinary tract infections, edema and diarrhea (Zhao, 2013). Furthermore, methanolic extracts of P. squamous demonstrated excellent antagonism against Pseudomonas aeruginosa without toxicity to hepatocytes (Fernandes et al., 2016). The antimicrobial activities of the fungal genus have been majorly attributed to steroids, anthraquinones, polysaccharides and nucleosides (Zhao, 2013).
Although, antimicrobial activities have not been reported so far on Inonotus pachyphloeus, the fungus strain KE/16-101 identified as I. pachyphloeus, exhibited mild antimicrobial activity on E. coli and moderate inhibitory effects on C. tenuis in this study. Nevertheless, reports from previous studies on related species I. hispidus and I. obliquus demonstrated their antimicrobial potential (GlamoÄlija et al., 2015; Suay et al., 2000). I. hispidus was reported to contain phenolic compounds hispolon and hispidin, which could be responsible for its high activity against a human isolate Aspergillus fumigatus (Suay et al., 2000). In addition, I. obliquus ethanolic and aqueous extracts exhibited lower antibacterial activity against Pseudomonas aeruginosa, as compared to the control antibiotics. I. obliquus extracts also demonstrated a higher activity compared to ketoconazole antifungal against Trichoderma viridae and Penicillin oochrochloron (GlamoÄlija et al., 2015).
Although the extracts from strains KE/16-175, KE/16-103 and KE/16-153 identified as Ganoderma sp, Perenniporia sp and Inonotus sp respectively, were not active against any of the microbial test pathogens used, antimicrobial activities have been reported for some species. Nonetheless, Ganoderma lucidium was reported as the most famous traditionally used medicinal mushroom (Alves et al., 2012). Also, its various extracts have been found to be effective in a similar level to gentamycin sulphate against bacterial pathogens, with the acetone extract being the most potent. In addition, highest activities against both Gram positive and Gram negative bacteria especially Micrococcus luteus were obtained with the aqueous extracts. Moderate inhibitions against B. subtilis and S. aureus have also been reported (Quereshi et al., 2010). Perenniporia sp has been described taxonomically by studies done by Decock and Bitew (2012) however, there are no documented bioactivities of the strain. Therefore, absence of any significant antimicrobial activity by KE/16-103 was also noted in this study.
The results obtained in the current study, clearly demonstrated that the crude extracts obtained from Basidiomycetes’ cultures possessed significant antibacterial and antifungal activities. The fungal metabolite output was shown to be enhanced by media contents variation owing to diverse nutrient preferences by different strains. There also exists a great diversity among Basidiomycetes fungi in the undisturbed sections of tropical forests such as the Mt. Elgon. Therefore, large scale fermentation, fractionation, purification and toxicological studies of the compounds present in other bioactive Basidiomycetes fungal strains as well as those reported herein can be carried out. This will lead to the identification of novel secondary metabolites responsible for the antimicrobial activities, hence their exploitation as leads in the discovery of antimicrobial drug compounds to keep up pace with the evolution of ‘superbugs’.
The authors have not declared any conflict of interests.
The authors are grateful to the Kenya Wildlife Services (KWS), who provided guidance and allowed collection of samples from Mt. Elgon National Park Forest. Also, they are grateful to the mycology department of Helmholtz Centre for Infection Research, Braunschweig, Germany for their assistance with the laboratory resources used in fungal identification and the bioassays. They also remain indebted to Dr. Cony Decock of Université Catholique de Louvain, Belgium for his assistance through the collection and identification of the fungal specimen.
REFERENCES
Al-Fatimi M, Schröder G, Kreisel H, Lindequist U (2013). Biological activities of selected basidiomycetes from Yemen. Die Pharmazie-An International Journal of Pharmaceutical Sciences 68(3):221-226.
|
|
Alves MJ, Ferreira IC, Dias J, Teixeira V, Martins A, Pintado M (2012). A review on antimicrobial activity of mushroom (Basidiomycetes) extracts and isolated compounds. Planta Medica 78(16):1707-1718.
Crossref
|
|
|
Bellemain E, Carlsen T, Brochmann C, Coissac E, Taberlet P, Kauserud H (2010). ITS as an environmental DNA barcode for fungi: an in silico approach reveals potential PCR biases. BMC Microbiology 10(1):189-196.
Crossref
|
|
|
Blaalid R, Kumar S, Nilsson RH, Abarenkov K, Kirk PM, Kauserud H (2013). ITS1 versus ITS2 as DNA metabarcodes for fungi. Molecular Ecology Resources 13(2):218-224.
Crossref
|
|
|
Blackwell M (2011). The Fungi: 1, 2, 3… 5.1 million species? American Journal of Botany 98(3):426-438.
Crossref
|
|
|
Chepkirui C, Richter C, Matasyoh JC, Stadler M (2016). Monochlorinated calocerins AD and 9-oxostrobilurin derivatives from the basidiomycete Favolaschia calocera. Phytochemistry 132:95-101.
Crossref
|
|
|
Christen R (2008). Global sequencing: a review of current molecular data and new methods available to assess microbial diversity. Microbes and Environments 23(4):253-268.
Crossref
|
|
|
Danishuddin M, Kaushal L, Baig MH, Khan AU (2012). AMDD: Antimicrobial drug database. Genomics, Proteomics & Bioinformatics 10(6):360-363.
Crossref
|
|
|
De Silva DD, Rapior S, Fons F, Bahkali AH, Hyde KD (2012). Medicinal mushrooms in supportive cancer therapies: an approach to anti-cancer effects and putative mechanisms of action. Fungal Diversity 55(1):1-35.
Crossref
|
|
|
De Silva DD, Rapior S, Sudarman E, Stadler M, Xu J, Alias SA, Hyde KD (2013). Bioactive metabolites from macrofungi: ethnopharmacology, biological activities and chemistry. Fungal Diversity 62(1):1-40.
Crossref
|
|
|
Decock C, Bitew A (2012). Studies in Perenniporia (Basidiomycota). African taxa VI. A new species and a new record of Perenniporia from the Ethiopian Afromontane forests. Plant Ecology and Evolution 145(2):272-278.
Crossref
|
|
|
Decock C, Bitew A, Castillo G (2005). Fomitiporia tenuis and Fomitiporia aethiopica (Basidiomycetes, Hymenochaetales), two undescribed species from the Ethiopian highlands: taxonomy and phylogeny. Mycologia 97(1):121-129.
Crossref
|
|
|
Fernandes Â, Petrović J, Stojković D, Barros L, GlamoÄlija J, Soković M, Martins A, Ferreira IC (2016). Polyporus squamosus (Huds.) Fr from different origins: Chemical characterization, screening of the bioactive properties and specific antimicrobial effects against Pseudomonas aeruginosa. LWT-Food Science and Technology 69:91-97.
Crossref
|
|
|
Fischer M, Edwards J, Cunnington JH, Pascoe IG (2005). Basidiomycetous pathogens on grapevine: a new species from Australia-Fomitiporia australiensis. Mycotaxon 92:85-96.
|
|
|
Gardes M, Bruns TD (1993). ITS primers with enhanced specificity for basidiomycetesâ€application to the identification of mycorrhizae and rusts. Molecular Ecology 2(2):113-118.
Crossref
|
|
|
Geethangili M, Tzeng YM (2011). Review of pharmacological effects of Antrodia camphorata and its bioactive compounds. Evidence-Based Complementary and Alternative Medicine 2011:1-17.
Crossref
|
|
|
GlamoÄlija J, Ćirić A, Nikolić M, Fernandes Â, Barros L, Calhelha RC, Ferreira IC, Soković M Van Griensven LJ (2015). Chemical characterization and biological activity of Chaga (Inonotus obliquus), a medicinal "mushroom". Journal of Ethnopharmacology 162:323-332.
Crossref
|
|
|
Graniti A (2006). From 'Fire Esca' to' Esca of Grapevine'. Phytopathologia Mediterranea 45(4):5-11.
|
|
|
Halecker S, Surup F, Kuhnert E, Mohr KI, Brock NL, Dickschat JS, Junker C, Schulz B, Stadler M (2014). Hymenosetin, a 3-decalinoyltetramic acid antibiotic from cultures of the ash dieback pathogen, Hymenoscyphus pseudoalbidus. Phytochemistry 100:86-91.
Crossref
|
|
|
Kearse M, Moir R, Wilson A, Stones-Havas S, Cheung M, Sturrock S, Buxton S, Cooper A, Markowitz S, Duran C, Thierer T (2012). Geneious Basic: an integrated and extendable desktop software platform for the organization and analysis of sequence data. Bioinformatics 28(12):1647-1649.
Crossref
|
|
|
Ko TWK, Stephenson SL, Bahkali AH, Hyde KD (2011). From morphology to molecular biology: can we use sequence data to identify fungal endophytes? Fungal Diversity 50(1):113-120.
Crossref
|
|
|
Kozlovskii AG, Zhelifonova VP, Antipova TV (2013). Fungi of the genus Penicillium as producers of physiologically active compounds (Review). Applied Biochemistry and Microbiology 49(1):1-10.
Crossref
|
|
|
Larkin MA, Blackshields G, Brown NP, Chenna R, McGettigan PA, McWilliam H, Valentin F, Wallace IM, Wilm A, Lopez R, Thompson, JD (2007). Clustal W and Clustal X version 2.0. Bioinformatics 23(21):2947-2948.
Crossref
|
|
|
Letunic I, Bork P (2016). Interactive tree of life (iTOL) v3: an online tool for the display and annotation of phylogenetic and other trees. Nucleic Acids Research 44(W1):W242-W245.
Crossref
|
|
|
Lindahl BD, Nilsson RH, Tedersoo L, Abarenkov K, Carlsen T, Kjøller R, Kõljalg U, Pennanen T, Rosendahl S, Stenlid J, Kauserud H (2013). Fungal community analysis by highâ€throughput sequencing of amplified markers–a user's guide. New Phytologist 199(1):288-299.
Crossref
|
|
|
Ling LL, Schneider T, Peoples AJ, Spoering AL, Engels I, Conlon BP, Mueller A, Schäberle TF, Hughes DE, Epstein S, Jones M (2015). A new antibiotic kills pathogens without detectable resistance. Nature 517(7535):455-459.
Crossref
|
|
|
Porter TM, Golding GB (2012). Factors that affect large subunit ribosomal DNA amplicon sequencing studies of fungal communities: classification method, primer choice, and error. PLoS One 7(4):e35749.
Crossref
|
|
|
Pryce TM, Palladino S, Kay ID, Coombs GW (2003). Rapid identification of fungi by sequencing the ITS1 and ITS2 regions using an automated capillary electrophoresis system. Medical Mycology 41(5):369-381.
Crossref
|
|
|
Pu X, Qu X, Chen F, Bao J, Zhang G, Luo Y (2013). Camptothecin-producing endophytic fungus Trichoderma atroviride LY357: isolation, identification, and fermentation conditions optimization for camptothecin production. Applied Microbiology and Biotechnology 97(21):9365-9375.
Crossref
|
|
|
Quereshi S, Pandey AK, Sandhu SS (2010). Evaluation of antibacterial activity of different Ganoderma lucidum extracts. Journal of Scientific Research 3:9-13.
|
|
|
Robledo GL, Rajchenberg M (2007). South American polypores: first annotated checklist from Argentinean Yungas. Mycotaxon 100:5-9.
|
|
|
Rosa LH, Machado KMG, Jacob CC, Capelari M, Rosa CA, Zani CL (2003). Screening of Brazilian basidiomycetes for antimicrobial activity. Memórias do Instituto Oswaldo Cruz 98(7):967-974.
Crossref
|
|
|
Ryvarden L, Johansen I (1980). A preliminary polypore flora of East Africa. Oslo, Norway: Fungiflora.
|
|
|
Schoch CL, Seifert KA, Huhndorf S, Robert V, Spouge JL, Levesque CA, Chen W, Bolchacova E, Voigt K, Crous PW, Miller AN (2012). Nuclear ribosomal internal transcribed spacer (ITS) region as a universal DNA barcode marker for Fungi. Proceedings of the National Academy of Sciences 109(16):6241-6246.
Crossref
|
|
|
Stadler M, Tichy HV, Katsiou E, Hellwig V (2003). Chemotaxonomy of Pochonia and other conidial fungi with Verticillium-like anamorphs. Mycological Progress 2(2):95-122.
Crossref
|
|
|
Suay I, Arenal F, Asensio FJ, Basilio A, Cabello MA, Díez MT, García JB, Del Val AG, Gorrochategui J, Hernández P, Peláez F (2000). Screening of basidiomycetes for antimicrobial activities. Antonie van Leeuwenhoek 78(2):129-140.
Crossref
|
|
|
Ventola CL (2015). The antibiotic resistance crisis: part 1: causes and threats. Pharmacy and Therapeutics 40(4):277-283.
|
|
|
Wagner T, Fischer M (2002). Proceedings towards a natural classification of the worldwide taxa Phellinus sl and Inonotus sl, and phylogenetic relationships of allied genera. Mycologia 94(6):998-1016.
Crossref
|
|
|
Wasser SP (2011). Current findings, future trends, and unsolved problems in studies of medicinal mushrooms. Applied Microbiology and Biotechnology 89(5):1323-1332.
Crossref
|
|
|
Waterhouse AM, Procter JB, Martin DM, Clamp M, Barton GJ (2009). Jalview Version 2—a multiple sequence alignment editor and analysis workbench. Bioinformatics 25(9):1189-1191.
Crossref
|
|
|
White TJ, Bruns T, Lee SJWT, Taylor JL (1990). Amplification and direct sequencing of fungal ribosomal RNA genes for phylogenetics. PCR Protocols: A Guide to Methods and Applications 18(1):315-322.
|
|
|
World Health Organization (2014). Antimicrobial Resistance: Global Report on Surveillance. Avenue Appia, Geneva: World Health Organization.
|
|
|
Zhao YY (2013). Traditional uses, phytochemistry, pharmacology, pharmacokinetics and quality control of Polyporus umbellatus (Pers.) Fries: A review. Journal of Ethnopharmacology 149(1):35-48.
Crossref
|
|