ABSTRACT
This study aims at bringing to light the preservative potential of essential oils of Thymus vulgaris, Cinnamomum zeylanicum and Mentha piperita in the stabilisation of lipids against oxidation. The essential oils were extracted by hydrodistillation and chemical composition were analysed by gas chromatography coupled with mass spectrometry. In vitro antioxidant potentials of the essential oils were tested by the 2, 2-diphenyl-1-picrylhydrazyl (DPPH) and hydrogen peroxide (H2O2) methods. The effects of essential oils on palm olein during accelerated storage for 20 days were carried out using the Shaal Oven test and indices of oxidation were measured. Essential oil of T. vulgaris was the most active oil with respect to the DPPH and H2O2 tests. T. vulgaris and C. zeylanicum showed varying degree of inhibition to palm olein oxidation within 20 days of storage at 60°C. Essential oils of T. vulgaris and C. zeylanicum at a concentration of 300 ppm showed comparable results to that of tert-butyl hydroquinone (TBHQ) applied at the recommended dose of 200 ppm. Palm olein samples treated with the essential oils did not show rancid characteristics at the end of storage. These essential oils significantly stabilised palm olein during accelerated storage conditions and may be exploited for use as preservatives in food products.
Key words: Antioxidant, essential oils, Thymus vulgaris, Cinnamomum zeylanicum and Mentha piperita, palm olein, food preservatives.
The presence of oxygen is a key factor in the susceptibility of lipid food to quality loss. Independent of the mechanism, the spontaneous reaction of lipids with atmospheric oxygen causes oxidation (Johnson and Decker, 2015), resulting in undesirable changes in flavour, texture, appearance and nutritional quality of food products (Waraho et al., 2011). This degradation does not only result in considerable loss of foodstuffs, but consumption of such foods may induce certain degenerative diseases and mortality due to the presence of products of oxidation (Addis and Warner, 1991; Wasowicz et al., 2004). Synthetic antioxidants used as food preservatives, have been found to be hypersensitive, allergenic and mutagenic (Anand and Sati, 2013).
Plants are continually being exploited for their biological virtues and can be used to resolve food insecurity, as well as the health of the consuming population. The biological properties of aromatic plants are attributed to essential oils (Hulin et al., 1998). These essential oils are natural molecules, generally recognised as safe and have broad spectrum of action and represent an undeniable hope in the resolution of these problems. It is widely reported that essential oils possess antioxidant potentials (Jazet et al., 2008, 2010; Goudoum et al., 2009; Dabire et al., 2012).
Thymus, Mentha and Cinnamum species are essential oil producing plants, and have been exploited for different applications. For instance, several studies have revealed the antioxidant, anti-viral, anti-inflammatory and antimicrobial potential of the essential oil of T. vulgaris (Amiri, 2012; Sessou et al., 2012). Essential oils of Mentha species are generally employed to flavour liquors, salads, soups and cheese, as well as ingredient in cosmetic products (Yadegarinia et al., 2006). While C. zeylanicum is known to lower blood low density lipo-protein concentration and hence cholesterol (Aynur and Sedef, 2008).
In a bid to search for alternative to synthetic food preservatives with local plants, this study was set up to exploit the preservative potentials of the essential oils of T. vulgaris, C. zeylanicum and M. piperita in the stabilisation of lipids against oxidation at accelerated storage conditions. This will contribute to food safety by using the essential oils of some Cameroon flora to preserve food.
The essential oils were extracted from fresh leaves and stem of T. vulgaris which was harvested in Dschang and fresh leaves of C. zeylanicum and leaves and stem of M. piperita were harvested in Mbouda. Dschang and Mbouda are found in the West Region of Cameroon. Palm Olein was purchased from an industrial producer.
Chemical composition analysis of essential oils
The essential oils, extracted by hydrodistillation, was analysed by gas chromatography coupled with mass spectrometry as reported by Ambindei et al. (2017).
In vitro antioxidant activities of essential oils
DPPH (2, 2-diphenyl-1-picrylhydrazyl) activity
It is known that DPPH readily produces free radicals as DPPH*, which are then neutralised by the test substance, RH in this case, the essential oil. Scavenging of the DPPH* is visualised by the disappearance of the purple colour of the DPPH solution to yellow as they are being neutralised to a stable form. The scavenging rate is read at 517 nm wavelength. The scavenging rate of the test substance was evaluated with respect to a molecule of reference, butylated hydroxytoluene BHT, which is a monophenolic synthetic antiradical (Jazet et al., 2010).
Antiradical potential of BHT:
1. DPPH solution (Solution A, SA): With the aid of an electronic balance, 19.7 mg of DPPH granules were weighed and dissolved in 50 mL ethanol. This solution served as the mother DPPH solution. The solution to be used for experimentation was prepared by the removal of 5 mL from the mother solution and was dissolved in 45 mL ethanol, giving a 1/10 dilution.
2. BHT solution: In the 50 mL methanol, 10 mg of BHT crystals were dissolved to give the mother BHT solution of concentration 1 g/l or 4.5 mmol/L.
3. Preparation of standard: From the BHT mother solution, standard solutions were prepared with the following concentrations: 0, 0.1, 0.2, 0.3 and 0.4 mg/mL.
From each concentration of standard, a fixed amount of the solutions was reacted with a given quantity of DPPH solution A. The reaction mixtures were left in the dark at room temperature and the absorbance read after an hour.
Antiradical potentials of samples
As in the BHT test, varying concentrations of essential oil were prepared: 0, 0.5, 1.5, 2, 2.5 and 3 mg/mL.
From each tube, 50 µL of essential oil solution was reacted with 950 µL DPPH solution A. The mixture from SA served as a negative control since no essential oil was added. A BHT mother solution and DPPH solution A were prepared, and this served as a positive control. The reaction mixtures were left in the dark at room temperature and the absorbance read after an hour on a multi-tube PC SPECTRONIC GENESYS 2PC spectrophotometer.
The blank solution was made of 950 µL ethanol and 50 µL methanol. The percentage scavenging capacity (SC) was calculated using the formula:
Where Aref = Absorbance at t = 60 min of control (without inhibitor); Asam = Absorbance at t = 60 min of sample and A100 = Absorbance at the end of reaction for total entrapment (positive control).
Hydrogen peroxide (H2O2) activity
The ferrous oxidation-xylenol orange (FOX) assay as described by Meisner and Gebicki (2009) was used with some modifications.
In an acidic medium, and in the presence of hydrogen peroxide (H2O2), ferrous ion (Fe2+) is oxidized to ferric ion (Fe3+). When the mixture is put in the presence of xylenol orange (3,3' - bis [N, N - di (Carboxymethyl) - aminomethyl] - o - cresolsulfone - phthaleine tetrasodium salt) ferric ions are fixed, forming a complex with a stable violet colouration which can be measured at 560 nm. The colour intensity of the complex is proportional to the quantity of ferric ions; the latter being proportional to the quantity of hydrogen peroxide present in the medium. This reaction can be represented thus:
Fe2+ + H2O2→ Fe3+ + HO• + OH¯
Fe3+ + XO → [Fe3+ - XO]
The [Fe3+-xylenol Orange] complex has an extinction coefficient of 1.5 × 104 M-1cm-1 at 560 nm when ferric ions are in direct contact with xylenol orange. In the presence of an antioxidant in the medium, non-specific oxidation of iron is prevented, thereby causing an increase in the extinction coefficient of the purple complex, and hence a better stability of the complex.
Procedure: In clinical centrifugal propylene tubes containing 50 µL of hydrogen peroxide, 50 µL of each essential oil concentration was added. The mixture was then homogenised with the aid of a vortex then incubated in the dark for 30 min. After incubation, 900 µL of FOX reagent (prepared according to the protocol suggested by Gay and Gebicki (2002)) was introduced in each tube. The resulting mixture was then homogenized and incubated in the dark for 30 min. A negative control was prepared in the same conditions, but essential oil was replaced with 50 µL HPLC grade methanol. The blank used for spectrophotometric measurements was 10 µL methanol (HPLC grade). Control essential oils were prepared under the same conditions as the tests with the only difference that hydrogen peroxide was replaced by 50 µL distilled de-aerated water. The absorbance of all the prepared and incubated tubes was read in a spectrophotometer at 560 nm with a test specimen of 400 µL, and stabilization temperature of 37°C. The percentage inhibitions of each concentration of essential oil were calculated using the formula (Meisner and Gebicki 2009):

Where

is percentage inhibition of hydrogen peroxide; OD
c is optical density (absorbance) of the negative control; OD
EOt is optical density in the presence of the test essential oil and DO
EOc is optical density of the control essential oil in the absence of hydrogen peroxide
Stabilisation of palm olein by essential oils at accelerated storage conditions
The Schaal oven test initially described by Slater (1984), reported and modified by Przybylski et al. (2013) was modified in terms of storage temperature, duration and sampling frequency. Essential oils and palm olein were blended at varying concentrations of EOs (0, 100, 200 and 300 ppm) and put in an oven at 60°C. Two control samples were prepared: One in which essential oil was replaced with a standard antioxidant, Tertiary butyl hydroquinone (TBHQ) at a concentration of 200 ppm and stored at 60°C. The second sample contained no antioxidant and was stored at room conditions to ascertain the effect of temperature on storage. The peroxide and p-anisidine values of samples were determined after every five days. The acid value and percentage free fatty acid (%FFA) were determined at the beginning and at the end of the experiment.
The peroxide value, expressed as milli-equivalent oxygen per kilogram oil (meq O2/kg), gives an indication of primary oxidation products (hydroperoxides).
The anisidine value gives a measure of secondary oxidation, that is, when hydroperoxides decompose to carbonyl compounds notably aldehydes. These secondary products give the rancid characteristic smell of oils.
The Totox value indicates oil’s overall oxidation state. The lower the PV, the lower the AV and hence the lower the Totox value, the better the quality of oil (AOAC, 1990).
The acid value, which is approximately twice the % FFA value, measures how many fatty acids are cleaved from their parent molecules, the triglycerides or phospholipids. Cleavage of a free fatty acid from a parent molecule shows hydrolytic breakdown and is often used in whole biological systems as an indication of stress (Miller, 2015).
The peroxide, p-anisidine and acid values were determined according to the AOAC official methods (1990).
Fatty acid profile of refined palm olein
Palm Olein was first methylated to fatty acid methyl esters (FAMEs) prior to fatty acid profile analysis.
The FAMEs were prepared by dissolving the oil sample (20 mg) in 1 mL hexane and refluxed for 3 h with 2% methanolic sulphuric acid (2 mL). Extraction was then done with 1 mL hexane three times. The hexane layer was washed 2 to 3 times with 3% sodium bicarbonate and then with distilled water. The resulting FAME solution was transferred into graduated stoppered vials and stored in the refrigerator
The fatty acid composition of oil was determined by subsequent analysis of the fatty acid methyl esters (FAMEs) using gas chromatography (GC-2010, Shimadzu Japan) equipped with nitrogen flame ionization detector, in a DB23 capillary column of 30 m length, 0.32 mm id wide bore, 0.25 µm film thickness (Agilent technologies USA). From an initial temperature of 80°C the oven was programmed to 120°C, for 2 min, then to 240°C at a rate of 5°C/min and held at 280°C for a further time. The fatty acid composition was analysed comparing with standard FAMEs and were expressed as relative area percentages.
Peroxide value
AOAC official method 965.33: PV of oils and fats (1990) was employed.
Reagents
1. Acetic acid–chloroform solution: 3 volumes of glacial acetic acid with 2 volumes of chloroform were mixed.
2. Potassium Iodide (KI) solution, saturated: Excess KI was dissolved in freshly boiled water. Excess solid must remain (Saturated KI: approximately 10 g KI in 6 mL water).
3. Sodium thiosulphate standard solutions: 0.1 and 0.01N was prepared with freshly boiled and cooled water.
Determination
Five grams of palm olein sample were weighed in a 250 mL glass-stoppered flask. To this, 30 mL of acetic acid-chloroform was added and the flask swirled to enable dissolution. With occasional shaking, 30 mL of water was then added, giving an almost yellow colouration.
The resulting solution was slowly titrated with 0.1N sodium thiosulphate with vigorous shaking, until the yellow colour was almost gone. About 0.5 mL of 1% starch solution was added, changing the colour of the solution to blue. With vigorous shaking so as to release all the iodine from chloroform layer, titration was continued until the blue colour turned colourless. When titrated volume was less than 0.5 mL, 0.1 N Na2S2O3 was used. A blank experiment was conducted daily and must be ≤ 0.1 mL 0.1 N Na2S2O3 which was subtracted from sample titration.
The peroxide value in milli-equivalent peroxide per kilogram sample was calculated using the formula:
S = mL of Na2S2O3 (blank corrected); N = Normality of Na2S2O3; W = weight of sample in g.
p-Anisidine value
Preparation of anisidine reagent
For 50 mL solution, 0.125 g of p-anisidine was weighed and dissolved in glacial acetic acid in a 50 mL volumetric flask, avoiding strong light.
Preparation of test sample: To the nearest 1 mg, a sufficient mass of the prepared test sample (palm olein) was weighed directly into a 25 mL volumetric flask. The sample was dissolved in iso-octane and the volume completed with the same solvent.
Unreacted test solution: By means of a pipette, 5 mL of the test solution were transferred into a test-tube and 1 mL of glacial acetic acid was added. The tube was then stoppered and well shaken. The test-tube was kept in the dark for eight minutes, and within a further two minutes the absorbance was read.
Reacted test solution: With the aid of a pipette, 5 mL of the test solution were transferred into a test-tube and 1 mL of freshly prepared p-anisidine reagent was added. The tube was stoppered and well shaken. The test-tube was kept in the dark for eight minutes, and within a further two minutes the absorbance was read.
Blank: The blank was prepared in the same way as the reacted test solution but the test solution was replaced with an equi-volume of iso-octane.
Spectrophotometric measurements
The spectrophotometer was adjusted to zero absorption with iso-octane at 350 nm, and the following measurements were carried out against iso-octane:
A1: Reacted test solution which should be between 0.2 and 0.8 or adjust test sample
A0: Unreacted sample
A2: Blank sample which should be ≤ 0.2 or purify anisidine reagent
Results were recorded to one decimal place per the formula:
AV = p-anisidine value; m = mass of test portion in grams; V = volume in which the test sample was dissolved in mL (V = 25 mL); Q = sample content of the measured solution based on which the anisidine value is expressed, in grams per mL as Q = 0.01 g/mL; 1.2 = correction factor for the dilution of the test solution with 1 mL of glacial acetic acid.
Totox value
The Totox value was calculated using the formula AV + 2PV to indicate the oil’s overall oxidation state.
Acid value and percentage free fatty acid
The acid value is the number of mg of KOH/NaOH required to neutralize the free fatty acids in 1g of fat. The acid value was determined by the AOAC Official Method 940.28 (1990).
Procedure
Approximately 2 g of palm olein, (the test sample) were weighed in a 250 mL beaker. To this, 30 mL of chromatographic grade methanol was added, and the resulting solution heated till boiling. A drop of phenolphthalein indicator was added, and the solution titrated against 0.1 N alkali (potassium hydroxide).
The acid value was calculated using the formula:
M = molar mass of KOH; N = normality of KOH; V = volume of KOH used; W = weight of sample
The percentage free acid, as oleic acid, was calculated from the acid value using the relation:
Percentage FFA (%) = Acid value / 1.99.
Chemical composition of the essential oils
The compounds and their respective percentages present in the different essential oils as analyzed by GC/MS are shown in Table 1.
Antioxidant activities of the essential oils
The DPPH test
BHT activity: Figure 1 shows the percentage inhibition of BHT at varying concentrations on the DPPH*. The degree of inhibition of the DPPH radical is dependent on the concentration of BHT. Graphically, the minimum concentration of BHT needed to inhibit or scavenge 50% of DPPH* (SC50) was 0.07 ± 0.01 mg/mL of BHT.
Essential oils activity
The result of the antiradical properties of the essential oils of T. vulgaris, C. zeylanicum and M. piperita are presented in Figure 2.
Percentage inhibition of DPPH* increases as concentration of essential oil increases. The SC50 of essential oil and the reference antioxidant, BHT are shown in Table 2.
The SC50 values for the essential oils were respectively 0.75±0.07, 0.88 ± 0.04 and 1.08 ± 0.04 mg/mL for T. vulgaris, C. zeylanicum and M. piperita as against 0.07±0.01 mg/mL for BHT. The T. vulgaris essential oil was more active than C. zeylanicum which in turn was more active than M. piperita as revealed by their SC50 values. These differences in activity are a function of the chemical composition of the different essential oils.
Compared to BHT, the three essential oils were less active, as BHT had the smallest SC50 value of 0.07±0.01 mg/mL. In fact, BHT was 10 times more active than T. vulgaris, about 13 times more active than C. zeylanicum and about 15 times more active than M. piperita.
The hydrogen peroxide test
The result of the inhibition of hydrogen peroxide by the essential oils of T. vulgaris, C. zeylanicum and M. piperita are shown in Figure 3.
Inhibition of peroxide ions increased with increased concentration of essential oil. At a concentration of 2.5 mg/mL, T. vulgaris showed the highest inhibition (94.35 ± 2.29%) followed by ascorbic acid (90.47 ± 2.02%), C. zeylanicum (89.19 ± 0.71%) and by M. piperita (56.80 ± 2.96%). At all concentrations, the essential oil of T. vulgaris showed a higher antioxidant activity than the reference antioxidant, ascorbic acid. The concentrations of antioxidants necessary to scavenge 50% of the peroxide radicals are as recorded in Table 3.
Within the test concentration range of 0 to 2.5 mg/mL, all the three essential oils scavenged at least 50% of the peroxide radical. The T. vulgaris essential oil recorded the least IC50 value of 0.85 ± 0.05 mg/mL. There was no statistical difference (p < 0.05) between the IC50 values of C. zeylanicum (1.19 ± 0.03 mg/mL) and ascorbic acid (1.12 ± 0.08 mg/mL). The M. piperita essential oil was the least active (2.31 ± 0.09 mg/mL) of all three essential oils, and also had a lower activity than ascorbic acid.
In relative terms, T. vulgaris was about 1.3 times more active than ascorbic acid and C. zeylanicum, and about 3 times more active than M. piperita.
The high antioxidant activity of T. vulgaris compared to the other essential oils may be due to the presence of thymol and eugenol that have been shown to be very effective compared to cinnamaldehylde (in C. zeylanicum) and menthol and menthone (in M. piperita) (Wei and Shibamoto, 2010).
Stabilisation of refined palm olein
After subjecting palm olein to accelerated oxidation conditions, the potential of the essential oils of T. vulgaris and C. zeylanicum to stabilise or prevent oxidation was evaluated using them. The two essential oils were considered due to their relative high antioxidant activities with respect to the DPPH and hydrogen peroxide tests.
Fatty acid profile
The relative percentage of the different fatty acids in the Palm Olein oil used is shown in Table 4. The higher the degree of unsaturation of a fatty acid, the more prone the fatty acid is liable to oxidation. The most abundant fatty acid is the mono unsaturated oleic acid (18:1) constituting 46% of the total oil composition. Palmitic acid, a 16-carbon saturated fatty acid, constitutes 37% of the total oil composition. The double unsaturated fatty acid, linoleic acids (18:2) and occupied 11.5%. The palm olein under study had about 57.5% unsaturated fatty acids. The relative high amount of saturated fatty acids of about 42%, notably palmitic acid confers a more stable structure when stored at room temperature. Linoleic acid (11.5%), with double unsaturation will be more liable to oxidation, followed by oleic acid. This level of unsaturated fatty acids makes the palm olein a suitable substrate for oxidation studies.
Peroxide value (PV) of test samples within storage duration
Within the 20 storage days under accelerated storage conditions, the PV of the different samples are shown in Figures 4 and 5.
Generally, the peroxide values increased with the storage duration time but a fluctuation in the peroxide values of the negative control was noticed. The PV increased rapidly up to the 10th day, then a reduction in the rate of increase was noticed between the10th and 15th day. The slow increasing rate in PV between the 10th and 15th day may probably be due to an increase in the rate of secondary oxidation. Peroxide radicals, which are products of primary oxidation, are further oxidised to secondary oxidation products such as aldehydes, ketones, acids among others, thereby leading to a drop in PV as reported by Gordon (2001).
Statistical analysis showed significant differences between the concentrations of each essential oil, and in some cases, the same concentrations of the two essential oils had statistically similar results (p < 0.05). Thus, the stabilisation potential of TBHQ at 200 ppm was generally comparable to that of the essential oils at 300 ppm (Figures 4 and 5). Throughout the experiment, palm olein without essential oil stored at room temperature showed very little variation, with its PV being less than 3 meq.O2/kg oil. According to AOAC (1990), a PV below 10 meq.O2/kg oil is acceptable, and higher values are indication of deterioration. From the results obtained in this study, peroxide values were more or less in the acceptable range after 15 days of accelerated storage. At a concentration of 300 ppm, the essential oils prevented oxidation significantly as the treated palm olein still had peroxide values within the acceptable range.
The decrease in PV upon the addition of essential oil in the present study concords with the findings of Sana et al. (2012) who worked on the essential oils of Thymus, Salvia and Rosemarinus on the stability to autoxidation of refined oils. Dabire et al. (2012) used essential oil of Ocimum basilicum as a natural antioxidant at concentrations of 500 and 1000 ppm to prevent deterioration of cottonseed oil. Also, Cinnamon essential oil was found to significantly lower peroxide values of hazelnut and poppy oils (Özcan and Arslan 2011).
Para-Anisidine value (p-AV) of test samples within storage duration
Para-AV is a reliable measurement of the amount of secondary oxidation products (Zhang et al., 2010) giving a characteristic off-flavour, the state of rancidity at AV>10. The results of p-AV values of the samples within the test period are shown in Figures 6 and 7.
The p-AV of palm olein samples stored at 60°C increased with increase in storage duration, but reduced with increasing concentration of the essential oil. palm olein not treated with antioxidant and stored at room temperature was stable as all AV were statistically not different (p < 0.05). The essential oil of T. vulgaris at 300 ppm had similar results with TBHQ at 200 ppm. All samples treated with the essential oils had AV less than 10 by the 15th storage day. This implies a strong antioxidant effect of the essential oils with respect to the negative control. By the 20th day, at concentrations of 200 and 300 ppm, the essential oils had acceptable AV, that is, less than 10, implying a strong antioxidant effect of the essential oils as the peroxides were prevented from oxidation as reported by Zhang et al. (2010).
The variations in AV were like those of Turan (2013) who found T. vulgaris essential oil as the most active with respect to S. officinalis, R. officinalis and L. nobilis on the stability of canola oil during accelerated storage conditions.
Totox value (TV) of test samples within storage duration
The variation in TV is shown in Figures 8 and 9. The lower the TV, the better the oil quality (AOAC, 1990). Generally, TV decreased with increasing concentration of the essential oils. The essential oils at 300 ppm especially T. vulgaris, showed comparable results with that of the reference, TBHQ. After 20 days of storage, the TV of T. vulgaris at 300 ppm and TBHQ at 200 ppm were not statistically different (p < 0.05). The highest TV value was obtained with the palm olein sample without essential oils and stored at 60°C, while palm olein without essential oils stored at room temperature had the least TV. This implies that high temperatures during storage accelerate oxidation and hence hasten the deterioration of vegetable oil. The relatively high percentage of phenolic compounds and reactive benzene rings such as thymol, cavarcrol and cinnamaldehyde found in these essential oils could explain their strong antioxidant activity.
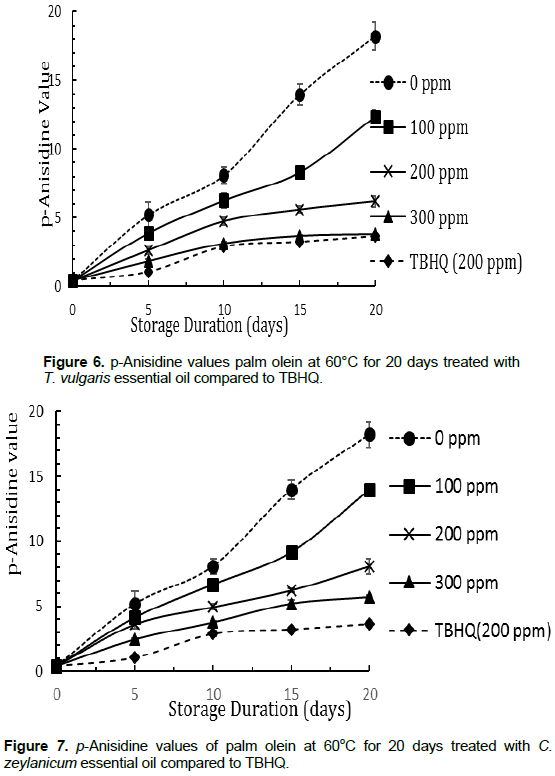
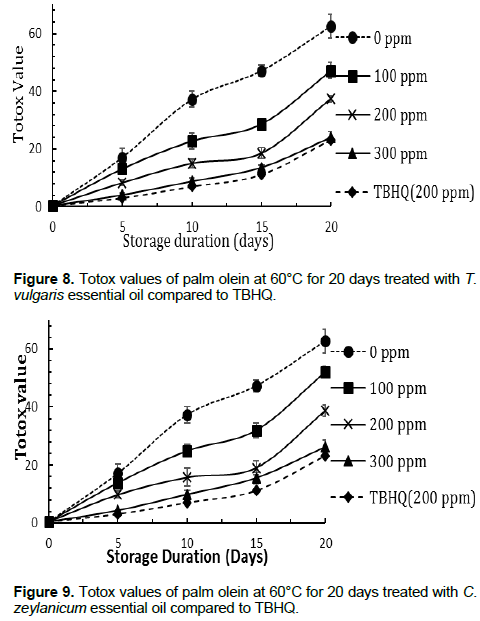
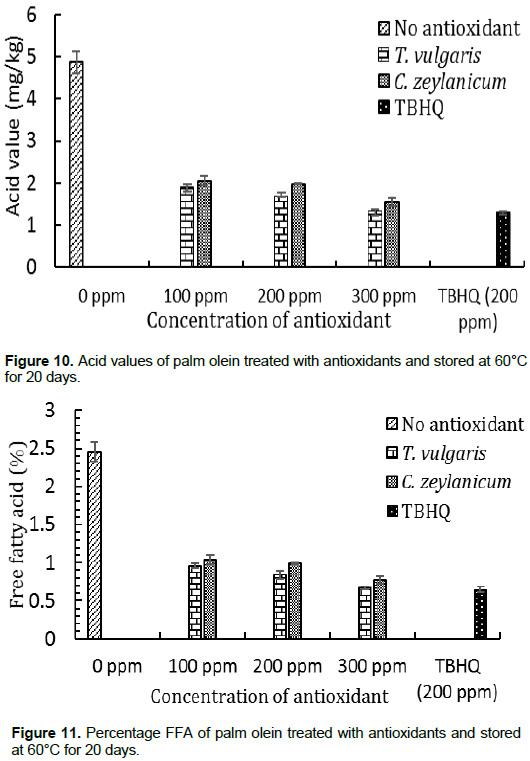
All samples subjected to accelerated storage conditions showed an increase in the acid values and hence the %FFA. These values increased with decreasing concentration of the essential oils. The negative control sample was not consumable at the end of the experiment, with an acid value of 4.88 ± 0.26 mg/kg and a %FFA of 2.45 ± 0.13%. These values are higher than the stipulated standards of 4 mg/kg and 1% respectively (AOAC, 1990). All the other samples were below 4 mg/kg, hence were consumable. However, the sample incorporated with C. zeylanicum essential oil at 200 ppm had a %FFA of 1.04 ± 0.06%, which is at the limit of the maximum required value.
Generally, T. vulgaris was the most active essential oil, with its acid value and % FFA at 300 ppm not significantly different from the reference antioxidant TBHQ. Palm Olein sample stored at room temperature without antioxidant showed minor variation in its acid value and %FFA at the end of the experiment compared to the initial values. Therefore, increased temperature during storage accelerated deterioration, and the presence of essential oils inhibited oxidation.
According to IUPAC (1987) as well as AOAC (1990), refined vegetable oil is considered edible if its PV is less than or equal to 10 meq of O2/kg, and its AV is less than 10, its acid value is less than or equal to 4 mg/kg, and its acidity or FFA is less than 1%. Taking into consideration the PV, AV, acid value and %FFA, a fat may have a high value of PV without any characteristic of rancidity. The PV is related to peroxidation and not to rancidity, as the latter is highlighted by the determination of secondary oxidation products (Dabire et al., 2012). Therefore, despite values of PV greater than 10 meq O2/kg, all samples treated with the essential oils could be considered consumable by the 15th day, and even at the end of the experiment as their AV, acid values and %FFA fell below the maximum values of 10, 4 mg/kg and 1% respectively.
The antioxidant potential of these essential oils is due to the presence of compounds such as thymol, eugenol, carvacrol, safrole, menthol, 1,8-cineole, α-terpineol, p-cymene, cinnamaldehyde that endow these essential oils as powerful antioxidants (Zeghad and Merghem, 2013). The phenolic group and / or benzene ring or reactive functional group they possess renders them very reactive and susceptible to bond formation. These essential oils reported in this study can be used in agro-food as natural antioxidants to conserve fatty foods or in all formulations containing fats.
The essential oils of T. vulgaris and C. zeylanicum remarkably stabilised palm olein against oxidation at accelerated storage conditions. Despite values of PV greater than 10 meq O2/kg, all samples treated with the essential oils could be considered consumable at the end of the experiment as their p-anisidine values, acid values and percentage FFA, which are true indicators of rancidity, were below the maximum values of 10, 4 mg/kg and 1%, respectively. These essential oils can, therefore, be exploited as suitable alternatives to synthetic chemicals as food preservatives. They can not only be exploited in food storage, but can as well be exploited as food additives for their antioxidant properties both in the food and eventually in the body of consumers.
The authors have not declared any conflict of interest.
REFERENCES
Addis PB, Warner GJ (1991). The potential health aspects of lipid oxidation products in food. in: Free Radicals and Food Additives (eds. Auroma OI, Halliwell B). Taylor and Francis, London, pp. 77-119.
|
|
Ambindei AW, Jazet DPM, Tatsadjieu NL, Priya P, Manilal VB, Krishnakumar B, Amvam ZPH (2017). Effect of the essential oils of Thymus vulgaris, Cinnamomum zeylanicum and Mentha piperita on fungal growth and morphology. African Journal of Biotechnology 16(9):388-399.
Crossref
|
|
|
Amiri H (2012). Essential oils composition and antioxidant properties of three Thymus species. Evidence-Based Complementary and Alternative Medicine 2012:728065.
Crossref
|
|
|
Anand SP, Sati N (2013). Artificial Preservatives and their harmful effects: Looking toward nature for safer alternatives. International Journal of Pharmaceutical Sciences and Research 4(7):2496-2501.
|
|
|
AOAC (Association of Analytical Chemist) (1990). Official Method of Analysis 925.10. 15th Edition. (Ed. Kenneth Helrich). Suite 400, 2200 Wilson Boulevard, Arlington, Virginia 22201 USA.
|
|
|
Aynur B, Sedef NE (2008). "Determination of In Vitro Antidiabetic Effects, Antioxidant Activities and Phenol Contents of Some Herbal Teas". Plant Foods for Human Nutrition 63(1):27-33
Crossref
|
|
|
Dabire CM, Nebie RCH, Pale E, Nacro M (2012). Cottonseeds oil conservation test by using Ocimum basilicum essential oil as natural antioxidant. Der Chemica Sinica 3(5):1305-1310.
|
|
|
Gay C, Gebicki JM (2002). Perchloric acid enhances sensitivity and reproducibility of the ferric-xylenol orange peroxide assay. Analytical Biochemistry 304(1):42-46.
Crossref
|
|
|
Gordon MH (2001). The development of oxidative rancidity, in: Antioxidants in Food - Practical Applications (eds. J. Pokorny, N. Yanishlieva, M. Gordon). CRC Press, Washington, pp. 7-22.
Crossref
|
|
|
Goudoum A, Ngamo TLS, Ngassoum MB, Mbofung CM (2009). Antioxidant activities of essential oils of Clausena anisata (Rutaceae) and Plectranthus glandulosus (Labiateae) plants used against stored grains insects in North Cameroon. International Journal of Biological and Chemical Sciences 3(3):567-577.
Crossref
|
|
|
Hulin V, Mathot AG, Mafart P, Dufosse L (1998). Les propriétés antimicrobiennes des huiles essentielles et composes d'arômes (Antimicrobial properties of essential oils and flavour compounds). Sciences des Aliments 18(6):563-582
|
|
|
IUPAC (International Union of Pure and Applied Chemistry) (1987): Standard methods for the analysis of oils, fats and derivatives. 7th revised and enlarged ed., Paquot C., Hautfenne A., Eds., Blackwell. Scientific publication.
|
|
|
Jazet DPM, Kuate J, Ngouana V, Damesse F, Tchinda E, Amvam ZPH, Menut C (2008). Analyse comparative des propriétés antiradicalaires et anti-inflammatoires des huiles essentielles de Citrus reticulata var. Madagascar et Citrus sinensis var. Casagrande du Cameroun. Fruits 63(4):201-208.
Crossref
|
|
|
Jazet DPM, Tchoumbougnang F, Ndongson DB, Agwanande W, Amvam ZPH, Menut C (2010). Chemical characterization, antiradical, antioxidant and anti-inflammatory potential of the essential oils of Canarium schweinfurthii and Aucoumea klaineana (Burseraceae) growing in Cameroon. Agriculture and Biology Journal of North America 1(4):606-611.
|
|
|
Johnson DR, Decker EA (2015). The Role of Oxygen in Lipid Oxidation Reactions: A Review. Annual Review of Food Science and Technology 6:171-190
Crossref
|
|
|
Meisner P, Gebicki JL (2009). Determination of hydroperoxides in aqueous solutions containing surfactants by the ferrous oxidation-xylenol orange method. Acta Biochimica Polonica 56(3):523-527.
Crossref
|
|
|
Miller M (2015). Oxidation of food grade oils. Plant and Food Research, 300 Wakefield Quay, Port Nelson, New Zealand. 2 p.
|
|
|
Özcan MM, Arslan D (2011). Antioxidant effect of essential oils of rosemary, clove and cinnamon on hazelnut and poppy oils. Food Chemistry 129(1):171-174.
Crossref
|
|
|
Przybylski R, Wu J, Eskin NAM (2013). A Rapid Method for Determining the Oxidative Stability of Oils Suitable for Breeder Size Samples. Journal of the American Oil Chemists' Society 90(7):933-939.
Crossref
|
|
|
Sana M, Sifi S, Chammem N, Sifaoui I, Mejri A, Hamdi M, Abderrabba M. (2012). Antioxidant effect of essential oils of Thymus, Salvia and Rosemarinus on the stability to autoxidation of refined oils. Annals of Biological Research 3(9):4259-4263.
|
|
|
Sessou P, Souaïbou F, and Sohounhloué D (2012). Major component and potential applications of plant essentials oils as natural food preservatives: a short review research results. International Journal of Biosciences 8(2):45-57.
|
|
|
Slater TF (1984). Overview of methods used for detecting lipid oxidation. Methods in Enzymology 105:283-293. doi: 10.1016/S0076-6879(84)05036-9
Crossref
|
|
|
Turan S (2013). Efficiency of various plant essential oils in stabilization of canola oil and of its purified triacylglycerols. Journal of Essential Oil Research 26(3):166-176.
Crossref
|
|
|
Waraho T, McClements JD, Decker EA (2011). Mechanisms of lipid oxidation in food dispersions. Trends in Food Science and Technology 22(1):3-13.
Crossref
|
|
|
Wasowicz E, Gramza A, Hes M, Jelen HH, Korczak J, Malecka M, Mildner-Szkudlarz S, Rudziñska M, Samotyja U, Zawirska-Wojtasiak R (2004). Oxidation of Lipids in Foods. Polish Journal of Food and Nutrition Sciences 13/54(SI1):87-100.
|
|
|
Wei A, Shibamoto T (2010). Antioxidant/lipoxygenase inhibitory activities and chemical compositions of selected essential oils. Journal of Agricultural and Food Chemistry 58(12):7218-7225.
Crossref
|
|
|
Yadegarinia D, Gachkar L, Rezaei MB, Taghizadeh M, Astaneh SA, Rasooli I (2006). Biochemical activities of Iranian Mentha piperita L. and Mentha communis L., essential oils. Phytochemistry 67(12):1249-1255
Crossref
|
|
|
Zeghad N, Merghem R (2013). Antioxidant and antibacterial activities of Thymus vulgaris L. Medicinal and Aromatic Plant Research Journal 1(1):5-11
|
|
|
Zhang Y, Yang L, Zu Y, Chen X, Wang F, Liu F (2010). Oxidative stability of sunflower oil by carnosic acid compared with synthetic antioxidants during accelerated storage. Food Chemistry 118(3):656-662.
Crossref
|
|