ABSTRACT
One of the best options for African countries to meet rural energy needs is to grow care-free crassulacean acid metabolism plants on a massive scale in waste lands. This can enable bioenergy production without disrupting food supplies and hence sustainable energy supply for the future. Opuntia ficus indica is an ideal plant for arid regimes but has barely been studied as a potential bioenergy source. This study investigated the effect of aerobic pretreatment on methane yield of O. ficus indica biomass. This effect was investigated in batch bioreactors which were exposed to aerobic conditions by varying time from 3 to 72 h before the start of anaerobic digestion. Reducing sugar content and dissolved oxygen levels after pretreatment period was analyzed. Reducing sugar content in bioreactors increased with increase in pretreatment time from 12.22 ±0.69 to 59.08 ± 5.35 g/L in the untreated and 72 h pretreated batches, respectively. Methane yields after pretreatment were observed to range from 0.286 to 0.702 L/kg volatile solids at 9 and 72 h of pre-treatment, respectively. A 9 h pre-treatment of feedstock prior to anaerobic digestion yielded 123% higher methane yield when compared to that without pre-treatment. The findings that there was an increase in reducing sugar production and methane yield at 9 h of aerobic pre-treatment suggests that there was increased hydrolysis with pretreatment. Hence, short pre-treatment period could be an option to increasing solubilization of cladodes and promoting methane productivity. Therefore, pre-aeration of O. ficus indica, was shown to be an effective method for enhancing both its digestibility and improved methane yield during anaerobic digestion.
Key words: Anaerobic digestion, biogas, methane, Opuntia, pretreatment, spineless cacti.
One requirement for sustainable development, especially in the developing countries is the availability of adequate energy to satisfy basic needs, improving social welfare and achieving economic development (Rogner et al., 2004). Some of the issues associated with the current major sources of fuel include the fact that they are non-renewable and therefore can be exhausted, and that they contribute to generation of greenhouse gases leading to global warming, and consequently climate change and its negative environmental impacts (Moshi et al., 2015).
To date, corn and sugarcane are the main feedstock used in the biofuel production (Moshi et al., 2015; Cruz et al., 2018); this is attributed to their relatively simple conversion to biofuel as well as availability of infrastructure for planting, harvesting, and processing which are already in place. Nevertheless, their large scale cultivation for biofuel production is associated with issues such as decrease in food availability and dramatically increase in food prices worldwide (Calabr et al., 2017; Jigar et al., 2011).
Therefore, there is a need to find alternative sources in order to reduce competition of these natural materials which are also used as human food and animal feed. Presently, with the reality of global warming, crassulacean acid metabolism (CAM) plants that can withstand and resist drought have become more attractive as feedstock for anaerobic digestion (Cushman et al., 2015; Yang et al., 2015). Among these plants is the fast-growing Opuntia ficus indica, which is known to have high water use efficiency. O. ficus indica is the most widely distributed species of the cactus family (Nobel et al., 2002 and Bobich 2002). This plant have been reported to pose a great potential as source of lignocellulosic biomass with a yield of 10 to 50 tonne dry mass/(year·ha) (Calabr et al., 2017; Consoli et al., 2013).
These are desert plants that can survive where most of the plants cannot grow (Tarisse, 2008), hence suitable plant resource for climate change adaptation. Using spineless cacti as a potential energy-generating crop may offer serious perspectives to countries prone to drought and relying on imports for their energy consumption (Tarisse, 2008; Nobel et al., and Bobich 2002 2002). Moreover, the ability to grow on unfertile land will make use of the land that currently is not occupied with agricultural crops and hence improve land utilization. The fact that spineless cacti is not used as food in most areas would reduce the competition of food versus fuel use (Calabr et al., 2017).
There is an increasingly interest in evaluating the potential of O. ficus indica as feedstock for anaerobic digestion and biogas production (Jigar et al., 2011; Egigu, 2014; Calabr et al., 2017; Ramos-Suarez et al., 2014; Yang et al., 2015). Nevertheless, limited studies have dealt with pretreatment of the plant cladodes prior to anaerobic digestion and the effect they could have on both methane production and yield.
A pretreatment method of lignocellulosic material is needed for an anaerobic process to reduce the volume of material used, increase solubility and production of methane (Antonopoulou et al., 2015; Antognoni et al., 2013; Tizé et al., 2016). Pretreatment of biomass prior to anaerobic digestion has been shown to enhance hydrolysis which is known to be a rate limiting step during anaerobic digestion, without degrading carbohydrates or forming by-products that are inhibitory to other processes downstream (Montgomery and Bochmann, 2014; Taherzadeh and Karimi, 2008; Xolisa et al., 2007).
Pretreatment of O. ficus indica has been investigated earlier by Calabr et al. (2017) who evaluated the effect of the thermal, alkaline and acidic pretreatments on the composition and biochemical methane potential. The authors found that only the acidic pretreatment (HCl) had significantly increased methane generation, while neither thermal nor alkaline pretreatment produced noticeably affects methane yields (an average reduction of 8% was recorded). These pretreatment methods may have some draw backs such as the use of hydrochloric acid can lead to a very low final pH, which could have a negative effect on the anaerobic digestion process (Calabr et al., 2017).
Though O. ficus indica have been studied for its potentials as animal feedstock and other agronomic applications, limited studies have exploited its potentials in anaerobic digestion (Jigar et al., 2011; Calabr et al., 2017). Currently, there is no scientific report on biological pretreatment of Opuntia prior to anaerobic digestion, most specifically aerobic pretreatment. This study therefore aims to characterize O. ficus indica as lignocellulosic feedstock for anaerobic digestion and evaluate effect of aerobic pretreatment on digestibility and methane potential of O. ficus indica.
O. ficus indica (L.) mill raw material
O. ficus indica used as feedstock was obtained from Dar es Salaam region in Tanzania, which is located at 6°48' South, 39°17' East (World Bank Group, 2011). The cladodes (green, plate-like sections) were the plant part of interest for this study. O. ficus indica in this study were sampled on non-irrigated and non-cultivated land (Figure 1). The cladodes were manually chopped into pieces averaging 1 cm3 with a sharp knife, blended at maximum speed using kitchen blender (Philips HR2067/04 600 W) placed in closed plastic containers and stored at 5°C until its further use.
Substrate inoculum
Cow rumen fluid obtained from Vingunguti abattoir, Ilala Municipal Dar es Salaam, Tanzania was used as a source of inoculum for this study. The fresh rumen fluid was collected and filtered through a sieve of 2 mm pores (Endecott’s Test Sieve Limited, BS 410, England) to separate solid content from the slurry. Twenty liters plastic containers with airtight lids were used to carry the inoculum at ambient temperature (31±1°C) to the laboratory. Prior to use, the inoculum was left to mature for 16 days at 31±1°C to remove the easily degradable volatile solid present in inoculum (Liew, 2011).
Determination of physico-chemical properties of the Opuntia biomass
Volatile solids (VS) and total solids (TS) of the substrate were determined by the oven-drying and ignition methods, respectively according to standard methods (APHA, 2006). Total carbon determination was done by using the Walkley-Black Potassium Dichromate method as described by Nelson and Sommers (1996). Total nitrogen determination was carried out using Kjeldahl method described by APHA (2006). The total carbohydrates, cellulose and hemicellulose determination were carried out using the procedure described by Allen (1989). The determination of acid detergent fiber followed the procedure described by Van Soest et al. (1991). Reducing sugar was determined using the Hagedorn-Jenson method based on quantitative oxidation by potassium ferricyanide (C6N6FeK3) and titration with sodium thiosulphate (Na2S2O3) as described by Allen (1989). Determination of pH of the biomass and effluent was determined before and after anaerobic digestion using a pH meter (HANNA HI 2211).
Batch bioreactor configuration
Anaerobic digesters were constructed in bench-scale experiments where biogas was produced out of the degradation of organic matter in 500 ml bioreactors consisting of wide mouth Erlenmeyer conical flasks which was connected to gas-tight aluminium-reinforced via a gas tight-plastic tubes for biogas collection (Figure 2). Gas sampling port was fitted in the bioreactor with n-butyl stoppers and sealed with aluminium caps as explained by Mshandete et al. (2005). Each bioreactor had a sampling septum made of rubber stopper for taking biogas samples and a gastight bag for collecting the gas.
Experimental set up of bioreactors
The experiment, which was carried out in a laboratory at a temperature of 31±1°C was set up in twenty-six bioreactors organized into two sets. The first set had six digesters without aeration including three which had untreated cladodes; the other three had inoculum only as controls. The second set of bioreactors comprised bioreactors with aeration in five different time intervals consisting of four bioreactors each.
Determination of the effect of aeration pretreatment on methane yield
Investigation of the effect of aerobic pretreatment on anaerobic digestion of O. ficus indica cladodes was done in anaerobic batch bioreactors using the rumen fluid as inoculums. To examine the effect of pre-treatment on the subsequent performance of batch anaerobic digestion of Opuntia biomass, experiments were carried out at five different times of aerobic exposure: 3, 9, 24, 48 and 72 h.
For each of the bioreactor, 20 g of the feedstock was added followed by inoculum to make up to 250 ml of working volume. Surface aeration was achieved by using a shaking incubator (Orbital Incubator S150, Stuart Scientific, UK), whereby bioreactors were left open and shaken at 130 rpm and 31°C for the aerobic period (3, 9, 24, 48 and 72 h). This allowed regular mixing of bioreactor contents and suitable condition for growth of hydrolytic bacteria. After every pretreatment period relative amount of dissolved oxygen was determined with an oximeter (OXI 3205, Weilheim 2009, Germany) and samples for the analysis of sugars content were centrifuged at 2000 g for 5 min (Thermofisher scientific, 41930819, Germany) and the supernatant was used to analyze reducing sugars as described by Allen (1989).
Immediately after the aeration periods, the content in each digester was flushed with nitrogen (N2) gas for 3 min to replace the oxygen and provide anaerobic conditions. Subsequently, the bioreactors’ openings were closed with stoppers to ensure gas tightness. Bioreactors were kept at a temperature of 31±1°C and shaken manually for 1 min, twice a day to provide regular substrate-inoculums distribution. These bioreactors were compared in terms of its biogas and methane content after every 72 h. All the tests were performed in triplicate 30 days.
Methane yield determination
The methane content was estimated by the concentrated alkaline absorption method described by Erguder et al. (2001) where concentrated potassium hydroxide (KOH) stock solution (20 g/L) at atmospheric pressure and 5 ml sample of the biogas was used. In this method, only methane was determined while other biogas components such as carbon dioxide (CO2) and hydrogen sulphide (H2S) were dissolved in the potassium hydroxide solution. The volume of biogas formed during the experiment was measured using a graduated 100 mL glass syringe (SGE International Pty Ltd., Ringwood, Australia) according to Pham et al. (2013).
Statistical analysis
Data was expressed as mean ± standard error (SE) of the triplicate measurements. Differences between mean values were examined by one-way analysis of variance (ANOVA) and significance was set at P = 0.05. All statistical analyses were performed using Prism version 6.01 for Windows.
Physico-chemical properties of the O. ficus indica biomass
The concentrations of various physico-chemical parameters of O. ficus indica feedstock are shown in Table 1. Moisture content of the biomass was quite high, around 88.05±2.23%. Total solid and volatile solid contents of O. ficus indica biomass obtained were 11.95 and 73.95%, respectively. The O. ficus indica cladodes were found to have 59.65±0.36% carbohydrates content. Initial pH readings for all digesters were around 7.5. Hemicellulose and cellulose were 33.10±0.11 and 8.39±0.01, respectively. The amount of carbon and nitrogen content in the feedstock made a ratio of around 30.6:1.
Reducing sugar contents in O. ficus indica biomass after pre-treatment
The amount of reducing sugar in the bioreactors during aerobic pre-treatment was between 12 and 59 g/L on the untreated and 72 h pretreated batch bioreactors, respectively. The increase of reducing sugar amount steadily increased as the pretreatment time increased reaching the highest amount on the bioreactor pretreated for 72 h as shown in Figure 3. The rise in reducing sugar contents can be seen as early as the 3 h pretreatment time. There was slight reduction in the increase of reducing sugar content on 24 and 48 h pretreatment time. Measurements were done in triplicates and values are represented as mean plus or minus standard error (represented as error bars).
Total methane production and methane yield during the incubation period
Methane yield (as meter cubic of methane per kilogram of volatile solids used) from the different pretreatment periods during the anaerobic digestion period is shown in Table 2. As seen from the results, the methane yield varied with pretreatment time of O. ficus indica feedstock. The highest and lowest methane yields were obtained from 9 and 72 h pretreatment, respectively. From these results, pretreatment increased the methane yield till around 9 h of pretreatment and then leveled off to around 0.286 L/kg VS for the 72 h pretreatment time. It can also be observed that there is no significant change after 24 h pretreatment in methane yield with further increase of exposure period to oxygen prior to anaerobic digestion.
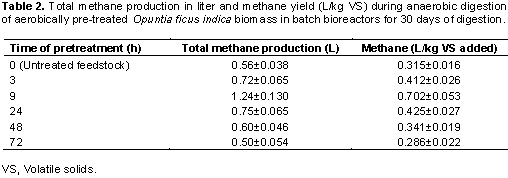
Using one way ANOVA from Prism version 6.01, the difference in methane yield among the pretreated groups was found to be of statistical significance with P value < 0.05. Pairwise comparison revealed that the difference was of statistical significance between the untreated group and the group pretreated for 9 h.
Effect of aeration pretreatment on methane yield potential of O. ficus indica feedstock
An increase in methane yield potential of 123% was recorded for the 9 h pretreatment time compared to the untreated O. ficus indica feedstock (Figure 4). The bioreactors which were pretreated in 3, 9, and 24 h showed high potential in increasing methane yield (greater than 30%) as compared to the bioreactor with no pretreatment on the other hand bioreactors pretreated for 48 and 72 h produced low methane yield 0.341±0.019 and 0.286±0.022 L/kg VS added, respectively compared to the control with the lowest amount on 72 h which showed potential 9% decrease in methane yield when compared with the batch without pretreatment.
Physical chemical properties
A great percentage of the O. ficus indica biomass obtained in this study was in moisture content of about 88.05%. The values obtained here are comparable with studies done on these plants elsewhere such as those in the study reported by Jigar et al. (2011) who worked with Opuntia in Ethiopia and reported the moisture content of 86%. Filho et al. (2016) evaluated moisture content of various Opuntia plants and reported a moisture content in the range of 88.7±1.2 to 92.6±0.7. This result shows that the moisture content of O. ficus indica is relatively high, which can aid anaerobic digestion as it can increase the degree of digestion since microorganisms in the digestate can easily access liquid substrate for relevant reactions to take place (Sadaka and Engler, 2003).
As a feedstock for anaerobic digestion in this study, the ratio of carbon and nitrogen of feedstock was 30.6 (Table 1). Nitrogen and carbon are among the key components needed by microorganisms in the development of their cell structures (Zhang et al., 2011; Ajay et al., 2011). Without forgetting the role played by nitrogen in cell growth which is very crucial in methane production but there is also buffering capacity by releasing ammonium cation contributed by nitrogenous compounds (Zheng et al., 2014). This ratio does not fall far from the carbon to nitrogen ratio of 20 to 32 recommended in anaerobic digestion of organic biomasses to provide enough nutrients for microorganisms performing anaerobic digestion (Costa et al., 2012; Zeshan et al., 2012; Wang et al., 2014). Nevertheless, the ratio obtained under this study is similar to that reported by Jigar et al. (2011) who obtained a range of 24 to 28 and Calabr et al. (2017) who obtained around 27:1 when dealing with Opuntia in the anaerobic digestion. It has been shown that various feedstocks used for anaerobic digestion can be optimized at a C/N ratio of approximately 6:1 to 30:1 (Costa et al., 2012); the optimum point of this ratio differs from feedstock to feedstock depending on the composition of feedstock in question.
TS and VS contents of feedstock used in this study were 11.95 and 73.95%, respectively. The values obtained from experiment were similar to the values reported by Jigar et al. (2011) who obtained the total solids and volatile solids contents of cladode as 14 and 78%, respectively. Determination of total solids and volatile solids of the feedstock is one of the important steps as these measures are not only used in the substrate loading but also represents part of the feedstock that may be transformed into biogas and methane (Motte et al., 2013; Ajay et al., 2011). The volatile solids obtained here indicating that the O. ficus indica have relatively large fraction which is biodegradable suitable for anaerobic digestion. A study conducted by Costa et al. (2012) working on methane production from poultry litter reported less productivity of the digestion of substrates having 7.6% total solids.
Feedstock used in this study was found to have 59.65±0.36% (Table 1) of carbohydrate which does not fall very far from that found by Malainine et al. (2003) who obtained roughly 69% total carbohydrates. Substrates hydrolyzed in the first stages of anaerobic digestion consist of carbohydrates, lipids, and proteins (Azman et al., 2015; Gerardi, 2003). In the anaerobic digestion process, structural carbohydrates are divided into their component sugars known as monosaccharides such as fructose and glucose that can be utilized by micro-organisms (Gerardi, 2003; Ajay et al., 2011). Hence, the amount of carbohydrate content of feedstock is of importance in the evaluation of the viability of the biomass as a feedstock for anaerobic digestion.
Cellulose content of O. ficus indica in this study was found to be 8.39±0.01%. This value falls in close range to those reported in literature such as 7.7±0.41 and 11%, reported by Calabr et al. (2017) as well as 7.95 to 13.73% reported by Ben-Thlija (2002) both of them working on Opuntia species. Cellulose is one among the major components of lignocellulosic plant materials contributing to about half to one third of plant tissues (Jorgensen et al., 2007). Cellulose is resistant to hydrolysis by enzymes or acids because of their structure and the lignin barrier, hence, the necessity of using pre-treatments at the initial stages of hydrolysis (Gujer and Zehnder, 1983). Pretreatment before aerobic digestion increased the accessibility of cellulose by microbial community by breaking the seal made by lignin (Antonopoulou et al., 2015).
In this study, the amount of acid detergent fiber was found to be 17.45± 0.044% of TS which falls in a very close range with that reported by Ben-Thlija (2002) which was in the range of 11.29 to 18.98% of TS; relatively low acid detergent fiber proportion gives them an appreciable digestibility level. Acid detergent fiber represents the least digestible fiber portion of feedstock and includes lignin, cellulose, silica and insoluble forms of nitrogen but not hemicellulose. Feedstock with high acid detergent fiber is low in energy (Von Cossel et al., 2018; Ben-Thlija, 2002).
Initial pH of the bioreactor contents for all digesters was around 7.5 (Table 1), this pH is suitable and within the range recommended for proper functioning of microorganisms in anaerobic digestion (Ward et al., 2008). This value is in agreement with a pH range of input mixture in the digester between 6.25 and 7.50, reportedly suitable for most methanogenic bacteria by Mahanta et al. (2004) and Ajay et al. (2011). While the pH of 3, 9 and 48 h pretreatment remained relative around the initial pH value (7.7, 7.62, and 7.63, respectively); there was noticeable increase in the pH of the batch without pretreatment and those of 24 and 72 h pretreatment (8.1, 8.08, and 8.15, respectively) after the completion of the incubation period.
The observed increase in pH value can be caused by various factors within the bioreactors. Amongst them being the production of alkali compounds, such as ammonium ions during the degradation of organic compounds in the digester (Gerardi, 2003). During fermentation, carbonic gas is formed and when combined with water it forms carbonates in anaerobic environment and hence can be a reason for the increase in pH. It is also possible that the formation of methane results in an increase in pH Jensen et al. (2017). Gray et al. (1971) also showed that pH value increased by accumulation of ammonia during degradation of protein whereas accumulation of volatile fatty acids from degradation of organic matter decreased the pH value. Though microorganisms can still be functioning, values of Ph methane-forming bacteria (Gerardi, 2003; Ajay et al., 2011).
Changes in reducing sugar contents in O. ficus indica biomass after pre-treatment
Variations were observed in the sugar concentration during pretreatment period, where the sugar levels increased with increase in pretreatment time from 12 to 59 g/L (Figure 3); this rise can be accounted for by the hydrolysis of polysaccharides to monosaccharides (Montalvo et al., 2016). The amount of sugar formed is also affected by the hydrolytic conditions and the microbial community present (Ahn et al., 2014). The low (22.17±0.47 g/L) amount of sugar content obtained during the initial hours of pretreatment can be due to the consumption of the sugars by the aerobic micro-organisms, which are active due to the provided aerobic conditions (Ahn et al., 2014). As the pretreatment is stopped the amount of sugar used is reduced and hydrolytic enzymes they produced are still active and continue to make soluble sugar available for the proper functioning of aerobic microorganism for aerobic digestion (Botheju et al., 2010).
Granados-Arvizu et al. (2017) obtained the highest amount of reducing sugar after pretreatment (about 80 g/L) while using corn pericarp under acid and temperature pretreatment. Montalvo et al. (2016) also obtained increase in concentration of total sugar during the first 48 h of aeration, where an increase of 192% was obtained with respect to its initial value.
It should be well noted that even though methane producing microorganism can hydrolyze insoluble carbohydrates, lower methane yields can be observed when the lignocellulosic materials are utilized without any kind of treatment (Zheng et al., 2014).
Indeed, the pretreatment increased the solubility of polysaccharide present in the bioreactor (Antonopoulou et al., 2015), as a result, increasing sugar levels. On one hand, these consequences may facilitate the anaerobic digestion by increasing the accessibility of these sugars to microorganisms. On the other hand, if pretreatment is carried out by increasing period of time above optimum, this could become problematic in systems as significant amounts of organic material were aerobically degraded before anaerobic digestion period is started (Ahn et al., 2014). Therefore, a compromise between increasing the solubility of substrate by aerobic pretreatment and prevention of overconsumption of soluble sugars due to consumption by microorganisms prior to anaerobic incubation needs to be found.
Effect of aeration pretreatment on methane yields
Methane yields after pre-aeration were observed to range from 0.286 to 0.704 L/kg VS, whereas for the untreated one was 0.315 L/kg VS (Table 2). Methane yields were higher with 9 h pre-aerated batch as compared to the others and lower on 72 h. When compared with the batch without pretreatment, potential increase of methane yield in the 9 h bioreactor was about 123% (Figure 4). This increase was significantly high (P = 0.0025) and is comparable to that which was observed by (Montalvo et al., 2016) who reported an increase of 110% in the production of methane when applied to the mixed sludge aerobic hydrolysis as compared to the digestion process of non-aerated sludge. It can be seen that pre aeration increased the methane yield till around 9th hour of pretreatment and then leveled off to around 0.286 L/kg VS a decrease which is about 9% for the pretreatment time of 72 h (Figure 4).
Since no research findings on aerobic pre-treatment of O. ficus indica feedstock are available, no direct comparison can be made. Nevertheless, various researchers have reported increase in methane content in biogas with the incorporation of aerobic process in anaerobic digestion (Rafieenia et al., 2017; Jang et al., 2014; Ahn et al., 2014). Results obtained in this study differ a bit from those of Ahn et al. (2014), who worked on anaerobic digestion of aerobic pretreated sewage sludge samples and obtained the highest methane yield after 24 h of pretreatment. The difference in pretreatment time which produced the highest methane yield could have been attributed to differences in the type of feedstock used, as maximum methane yield of an anaerobic digestion vary depending on several factors including feed composition (Botheju et al., 2010). Ahn et al. (2014) used sewage sludge which contains a complex mix of protein, lipids and carbohydrate. On the other hand, the results are similar to those obtained by Mshandete et al. (2005) who worked on anaerobic digestion of aerobic pretreated sisal pulp waste samples and obtained the highest methane yield after 9 h of pretreatment. Both of these studies showed no significant increase in methane potential with further increase in pretreatment time above the optimum obtained (9 and 24 h).
Increased methane yield can be attributed to improve biodegradability of aerobically pretreated O. ficus indica feedstock that came as a result of biological role played by microbes (Ahn et al., 2014; Conklin et al., 2007). It has been shown in some studies that short-term oxygen exposure of bioreactor does not affect methanogenic activity and methanogens can survive longer than previously reported (Conklin et al., 2007). The increase in methane yield as a result of increase of methanogenic activity can also be due to an improvement in the growth of facultative anaerobes, which can keep a low redox potential, providing the best conditions for the growth of strict anaerobes (Montalvo et al., 2016).
The experiments revealed that the methane yield is not significantly different with further increase of exposure period to oxygen prior to anaerobic digestion. Potential decrease of 9% was observed at 72 h pretreatment (Figure 4). These results are in agreement with some author’s findings who showed that long time exposure to oxygen does not significantly improve the methane yield (Xu et al., 2014; Botheju et al., 2009). In this study, dissolved oxygen measured ranged from 0.13 to 0.16 mg/L; the highest and the lowest value at 3 and 72 h pretreatment. In a study done by Ahn et al. (2014) reported that the dissolved oxygen values higher than 0.15 mg/L can start to inhibit methanogenic activities. Methane yield was the lowest on 72 h pretreatment (0.286 L/kg VS). Inhibition of the activities of methane forming bacteria and decrease in the methane yield can occur under longer exposure to oxygen as reported by Xu et al. (2014). This decrease in methane yield observed here could have been as a result of several factors such as the substrate oxidation of readily available substrates by facultative acidogenic organisms and the partial inhibition of the activity of strictly anaerobic biomass (Botheju et al., 2009). Methane consumption by aerobic methanotrophs (Fu et al., 2015) can also be a contributing factor to the decrease in methane yield observed.
The reduction of methane yield in bioreactors, which were pre aerated for longer periods have been reported by other researches (Fu et al., 2015; Ahn et al., 2014). Reduction in methane yield at 26 and 37% following pre-treatment for 48 and 72 h, respectively has been reported by Mshandete et al. (2005) while Ahn et al. (2014) obtained reduced methane yield at 48 and 96 h of pre-aeration which was linear with the period of pre-aeration. Botheju et al. (2010) also reported decreased amounts of methane generation under increased aeration conditions as a linear reduction within the oxygenation range of 0 to 10.1%.
Based on these findings, the best pretreatment time can be said to lie between 9 and 24 h as these provide enough time for the microorganisms during pretreatment to thrive and produce their effect. It can be seen that pre-aeration had an advantage in increasing the methane yield and more optimization of the pretreatment time with other factors is required to ensure maximum yield of methane from biomass and avoid the inhibition of microbial activities involved in methane production.
Aerobic pre-treatment of O. ficus indica feedstock prior to anaerobic digestion, showed significant effect on enhancing subsequent digestion of the substrate in batch anaerobic bioreactors using cow rumen fluid as an inoculum. Nine hours of pre-treatment showed 123% more methane yield potential than with untreated O. ficus indica feedstock. Prolonged pre-treatment periods of 48 and 72 h showed no increase in the methane yield potential from O. ficus indica feedstock, rather a decrease of 9% as compared to the untreated obtained at 72 h pretreatment. Above 24 h, methane production per kg of volatile solids added decreased with increase in pretreatment periods, this is possibly due to inhibition of activities of methane forming bacteria and substrate oxidation of readily available substrates by facultative acidogenic organisms.
Using aerobic pre-treatment for the period of time around 9 to 24 h has great potential to promote hydrolysis as well as solubilization of feedstock, as a result enhance the anaerobic digestion of O. ficus indica feedstock. Biological pretreatment (including aerobic) of lignocellulosic material for anaerobic digestion has received increased focus during recent years driven more by negative effect of other form of pretreatments including chemicals to environment. To reducing the overall production costs and avoiding aeration expenses, low level of oxygen applied in this study can be adopted and offer an added advantage in enhancing anaerobic digestion. Further studies of this pretreatment effect can be done at pilot level and analyze its applicability at industrial scale. Integrated two stage digestion system comprising aerobic pretreatment followed by anaerobic digestion have been reported (Global Renewables, 2014; Montgomery and Bochmann, 2014). The cultivation of O. ficus indica in marginal and waste lands will guarantee availability of feedstock, at the same time reduce land competition with food crops. This in turn will lead to reduction in prices of staple foods used as feedstock and hence generating fuel and more food rather than choosing between the two.
The authors have not declared any conflict of interests.
The authors gratefully acknowledge the Pan African University Institute of Basic Science, Innovation and Technology for the financial support.
REFERENCES
Ahn YM, Wi J, Park JK, Higuchi S, Lee NH (2014). Effects of Pre-aeration on the Anaerobic Digestion of Sewage Sludge. Environmental Engineering Research 19(1):59-66.
Crossref
|
|
Ajay KJ, Jianzheng L, Loring N, Liguo Z (2011). Research advances in dry anaerobic digestion process of solid organic wastes. African Journal of Biotechnology 10(65):14242-14253. DOI:10.5897/ajb11.1277
Crossref
|
|
|
Allen SE (1989). Chemical analysis of ecological materials. (2nd edn). Blackwell Scientific Publications, Oxford. pp. 166-173.
|
|
|
Antognoni S (2013). Potential effects of mechanical pre-treatments on methane yield from solid waste anaerobically digested. International Journal of Environmental Bioremediation & Biodegradation 1(1):20-25.
|
|
|
Antonopoulou G, Dimitrellos G, Vayenas D (2015). Different physicochemical pretreatment methods on lignocellulosic biomass: The effect on biochemical methane potential. In: Proceedings of the 14th International Conference on Environmental Science and Technology, Rhodes (Greece).
|
|
|
American Public Health Association (APHA) (2006). Standard Methods for the Examination of Water and Wastewater, 21st ed. American Public Health Association, Washington DC, USA. York, USA: American Public Health Association.
|
|
|
Azman S, Khadem AF, van Lier JB, Zeeman G, Plugge CM (2015). Presence and Role of Anaerobic Hydrolytic Microbes in Conversion of Lignocellulosic Biomass for Biogas Production. Critical Reviews in Environmental Science and Technology 45(23):2523-2564.
Crossref
|
|
|
Ben-Thlija A (2002). Nutritional value of several Opuntia species. Master Thesis, Oregon State University, Corvallis/USA, 1987; cit. in: Nefzaoui A, Ben Salem H. Forage, fodder, and animal nutrition, in: Nobel PS. (Ed.), Cacti. Biology and Uses, University of California Press, Berkeley, Los Angeles, London pp.199-210.
|
|
|
Botheju D, Lie B, Bakke R (2009). Oxygen effects in anaerobic diges¬tion. Modeling Identification and Control 30(4):191-201.
Crossref
|
|
|
Botheju D, Samarakoon G, Chen C, Bakke R (2010). An Experimental Study on the Effects of Oxygen in Bio-gasification-Part 2. Paper Presented at International Conference on Renewable Energies and Power Quality (ICREPQ10), Granada, Spain. https://doi.org/10.24084/repqj08.732
Crossref
|
|
|
Calabr AP, Catal A, Eva FA, Sanchez A, Komilis D (2017). Effect of three pretreatment techniques on the chemical composition and on the methane yields of Opuntia ficus-indica (prickly pear) biomass. Waste Management and Research 36(1):17-29.
Crossref
|
|
|
Conklin A, Bucher R, Stensel HD, Ferguson J (2007). Effects of oxy¬gen exposure on anaerobic digester sludge. Water Environment Research 79(4):396-405.
Crossref
|
|
|
Consoli S, Inglese G, Inglese P (2013). Determination of evapotranspiration and annual biomass productivity of a cactus pear (Opuntia ficus indica L. Mill) orchard in a semi-arid environment. Journal of Irrigation and Drainage Engineering 139(8):680-690.
Crossref
|
|
|
Costa JC, Barbosa SG, Alves MM, Sousa DZ (2012). Thermochemical pre- and biological co-treatments to improve hydrolysis and methane production from poultry litter. Bioresource Technology 111:141-147
Crossref
|
|
|
Cruz G, Santiago PA, Braz CEM, Seleghim P, Crnkovic PM (2018). Investigation into the physical–chemical properties of chemically pretreated sugarcane bagasse. Journal of Thermal Analysis and Calorimetry 132(2):1039-1053.
Crossref
|
|
|
Cushman JC, Davis SC, Yang X, Borland AM (2015). Development and use of bioenergy feedstocks for semi-arid and arid lands. Journal of Experimental Botany 66(14):4177-4193.
Crossref
|
|
|
Egigu M (2014). Efficiency of biogas production from cactus fruit peel co-digestion with cow dung. International Journal of Advanced Research 2(7):916-923.
|
|
|
Erguder T, Tezel U, Güven E, Demirer G (2001). Anaerobic biotransformation and methane generation potential of cheese whey in batch and UASB reactors. Waste Management 21(7):643-650.
Crossref
|
|
|
Filho De Souza, PF, Ribeiro VT, Santos ES, Macedo GR (2016). Simultaneous saccharification and fermentation of cactus pear biomass evaluation of using different pretreatments. Industrial Crops and Products 89:425-433.
Crossref
|
|
|
Fu SF, Wang F, Yuan XZ, Yang ZM, Luo SJ, Wang CS, Guo RB (2015). The thermophilic (55°C) micro aerobic pretreatment of corn straw for anaerobic digestion. Bioresource Technology 175:203-208.
Crossref
|
|
|
Gerardi MH (2003). The microbiology of anaerobic digesters. A John Wiley and Sons pp. 99-103.
Crossref
|
|
|
Global Renewables (2014). The UR-3R process. Information.
|
|
|
Granados-Arvizu JA, Amaro RA, García-Almendárez BE, Gracida-Rodríguez JN, Regalado C (2017). Optimization of dilute acid pretreatment of corn pericarp by response surface methodology. Bioresource 12(4):7955-7963.
|
|
|
Gray KR, Sherman K, Biddlestone AJ (1971). A Review of Composting Part 1. Process Biochemistry 6:32-36.
|
|
|
Gujer W, Zehnder AJ (1983). Conversion processes in anaerobic digestion. Water science and Technology 15(8-9):127-167.
Crossref
|
|
|
Jang HM, Cho HU, Park SK (2014). Influence of thermophilic aerobic digestion as a sludge pre-treatment and solids retention time of mesophilic anaerobic digestion on the methane production, sludge digestion and microbial communities in a sequential digestion process. Water Resource 48:1-14.
|
|
|
Jensen TR, Lastra Milone T, Petersen G, Andersen HR (2017). Accelerated anaerobic hydrolysis rates under a combination of intermittent aeration and anaerobic conditions. Water Science and Technology 75(8):1944-1951.
Crossref
|
|
|
Jigar E, Hameed S, Araya A (2011). Study on renewable biogas energy production from cladodes of Opuntia ficus indica. Journal of Food and Agriculture Science 1(3):44-48.
|
|
|
Jorgensen H, Kristensen JB, Felby C (2007). Enzymatic conversion of lignocellulose into fermentable sugars: challenges and opportunities. Biofuels, Bioproducts and Biorefining 1(2):119-134. DOI:10.1002/bbb.4119-134.
|
|
|
Liew LN (2011). Solid State Anaerobic Digestion of Lignocellulosic Biomass for Biogas Production. MSc Thesis, School of Graduate Studies of the Ohio State University, Ohio, USA.
|
|
|
Mahanta P, Dewan A, Saha UK, Kalita P (2004). Influence of Temperature and total solid concentration on the gas production rate of biogas digester. Journal of Energy in Southern Africa 15(4):112-117.
|
|
|
Malainine ME, Dufresne A, Dupeyre D, Mahrouz M, Vuong R, Vignon MR (2003). Structure and morphology of cladodes and spines of Opuntia ficus indica cellulose extraction and characterization. Carbohydrate Polymers 51(1):77-83.
Crossref
|
|
|
Montalvo S, Ojeda F, Huili-ir C, Guerrero L, Borja R, Castillo A (2016). Performance evaluation of micro-aerobic hydrolysis of mixed sludge: Optimum aeration and effect on its biochemical methane potential. Journal of Environmental Science and Health Part A Toxic/Hazardous Substances & Environmental Engineering 51(14):1269-1277.
Crossref
|
|
|
Montgomery LFR, Bochmann G (2014). Pretreatment of feedstock for enhanced biogas production. IEA Bioenergy, Dublin, Ireland.
|
|
|
Moshi AP, Nyandele JP, Ndossi HP, Sosovele ME, Hosea KM (2015). Feasibility of bioethanol production from tubers of Dioscorea sansibarensis and Pyrenacantha kaurabassana. Bioresource Technology 196:613-620.
Crossref
|
|
|
Motte JC, Trably E, Escudié R, Hamelin J, Steyer JP, Bernet N, Dumas C (2013). Total solids content: a key parameter of metabolic pathways in dry anaerobic digestion. Biotechnology for Biofuels 6(1):164.
Crossref
|
|
|
Mshandete A, Björnsson L, Kivaisi AK, Rubindamayugi ST, Mattiasson B (2005). Enhancement of anaerobic batch digestion of sisal pulp waste by mesophilic aerobic pre-treatment. Water Research 39(8):1569-1575.
Crossref
|
|
|
Nelson DW and Sommers LE (1996). Total carbon, organic carbon, and organic matter. In Methods of soil analysis, 3: 961-1010.
|
|
|
Nobel PS, Bobich EG (2002). Environmental biology Cacti: Biology and Uses pp. 57-74.
|
|
|
Pham CH, Triolo JM, Cu T, Pedersen L, Sommer SG (2013). Validation and Recommendation of Methods to Measure Biogas Production Potential of Animal Manure. Asian-Australasian Journal of Animal Sciences 26(6):864-873.
Crossref
|
|
|
Prism version 6.01 for Windows, Graph Pad Software, La Jolla California USA.
|
|
|
Rafieenia R, Girotto F, Peng W, Cossu R, Pivato A, Raga R, Lavagnolo MC (2017). Effect of aerobic pre-treatment on hydrogen and methane production in a two-stage anaerobic digestion process using food waste with different compositions. Waste Management 59:194-199.
Crossref
|
|
|
Ramos-Suarez JL, Martinez A, Carreras N (2014) Optimization of the digestion process of Scenedesmus sp. and Opuntia maxima for biogas production. Energy Conversion and Management 88:1263-1270.
Crossref
|
|
|
Rogner H, Fritz B, Maritess C, Andre F, Marc G, David H, Vladimir K, Serguei K, Thierry L, Roberto M, Notstaller R, Peter O, Martin T (2004). World energy Assessment. Energy and the Challenge of Sustainability. Energy Resources 5:136
|
|
|
Sadaka SS, Engler CR (2003). Effects of Initial Total Solids on Composting of Raw Manure with Biogas Recovery. Compost Science and Utilization 11(4):361-369.
Crossref
|
|
|
Taherzadeh MJ, Karimi K (2008). Pretreatment of lignocellulosic wastes to improve ethanol and biogas production: A review. International Journal of Molecular Sciences 9:1621-1651.
Crossref
|
|
|
Tarisse A (2008). Natural gas consumption of Turkey and the strategic use of drought tolerant energy crops for biogas production, Thesis, ESE-IER-Lyon III University - September 2008.
|
|
|
Tizé KJ, Sinbai M, Darman RD, Albert N (2016). Assessment of biofuel potential of dead neem leaves (Azadirachta indica) biomass in Maroua town, Cameroon. African Journal of Biotechnology 15(34):1835-1840.
Crossref
|
|
|
Van Soest PJ, Robertson JB, Lewis BA (1991). Methods for dietary fiber, neutral detergent fiber, and nonstarch polysaccharides in relation to animal nutrition. Journal of Dairy Science 74(10):3583-3597.
Crossref
|
|
|
Von Cossel M, Möhring J, Kiesel A, Lewandowski I (2018). Optimization of specific methane yield prediction models for biogas crops based on lignocellulosic components using non-linear and crop-specific configurations. Industrial Crops and Products 120:330-342.
Crossref
|
|
|
Wang X, Lu X, Li F, Yang G (2014). Effects of Temperature and Carbon-Nitrogen (C/N) Ratio on the Performance of Anaerobic Co-Digestion of Dairy Manure, Chicken Manure and Rice Straw: Focusing on Ammonia Inhibition. PLoS ONE 9(5):e97265.
Crossref
|
|
|
Ward AJ, Hobbs PJ, Holliman PJ (2008). Review: Optimization of the anaerobic digestion of agricultural resources. Bioresource Technology 99:7928-7940.
Crossref
|
|
|
World Bank group (2011). Dar es Salaam case study: overview climate change, disaster risk and the urban poor: cities building resilience for a changing world.
|
|
|
Xolisa M, Roman T, Jo B (2007). Anaerobic digestion of fungal pre-treated wine distillery wastewater. African Journal of Biotechnology 6(17):1990-1993.
Crossref
|
|
|
Xu S, Selvam A, Wong JW (2014). Optimization of micro-aeration intensity in acidogenic reactor of a two-phase anaerobic digester treating food waste. Waste Management 34(2):363-369.
Crossref
|
|
|
Yang L, Carl S, Lu M, Mayer J, Cushman J, Tian E, Lin H (2015). Biomass characterization of Agave and Opuntia as potential biofuel feedstocks. Biomass and Bioenergy 76:43-53
Crossref
|
|
|
Zeshan K, Karthikeyan OP, Visvanathan C (2012). Effect of C/N ratio and ammonia-N accumulation in a pilot-scale thermophilic dry anaerobic digester. Bioresource Technology 113:294-302
Crossref
|
|
|
Zhang L, Lee YW, Jahng D (2011). Anaerobic co-digestion of food waste and piggery wastewater: focusing on the role of trace elements. Bioresource Technology 102(8):5048-5059
Crossref
|
|
|
Zheng Y, Zhao J, Xu F, Li Y (2014). Pretreatment of lignocellulosic biomass for enhanced biogas production. Progress in Energy and Combustion Science 42:35-53.
Crossref
|
|