ABSTRACT
The degradation kinetics of different sources of 2,4-dichlorophenoxyacetic (2,4-D) and atrazine by the natural strain Mo008 of the basidiomycete fungus Trametes versicolor (L.:Fr.) Pilát was studied, knowing that in this process the strain used produces an enzyme complex composed of manganese peroxidase (MnP), lignin peroxidase (LiP) and laccase, and the response on the global consumption rate and yield is dependent on the 2,4-D source, being more efficient with an analytical source and mixture with atrazine than amine salt; however, it was more efficient to degrade atrazine. The strain studied (Mo008) consumed 1000 ppm of 2,4-D analytical, 2,4-D amine, 2,4-D plus atrazine and atrazine in 750, 850, 650 and 550 h, respectively.
Key words: Trametes versicolor, degradation kinetics, biodegradation, 2,4-dichlorophenoxyacetic (2,4-D), atrazine.
Lignin is one of the most abundant polymers in nature and the fungi that cause white rot of wood are responsible for initiating its depolymerization (Buswell and Odier, 1987; Gold et al., 1989; Kirk and Farrell, 1987; Szklarz et al., 1989). Ligninolytic fungi such as Trametes versicolor, Phanerochaette chrysosporium and Pleurotus ostreatus, have been extensively studied in the recovery of effluents from various industries due to the production of an enzymatic system capable of degrading lignin and phenolic compounds (Yateen et al., 1998; Tortella et al., 2008), which is composed of the manganese peroxidase (MnP), lignin peroxidase (LiP) and laccase (Rothschild et al., 1999; Guo et al., 2000; Ullah et al., 2000; Gómez et al., 2005).
Diverse species of basidiomicetes have been studied in recent years, due to their ability to degrade lignin and phenolic compounds (Córdoba et al., 2012; Betancur et al., 2013; Stamatiu et al., 2015). Studies in biotechnology and industrial applications of lignolytic fungi are focused on the species that cause white rot of the wood as a group in general, although this is taxonomically and physiologically heterogeneous, which has overestimated the importance of the best studied fungus, P. chrysosporium and has left aside other species with better qualities for biotechnological applications (Peralta et al., 1998; Nyanhongo et al., 2007).
T. versicolor is a basidiomycete that has the ability to degrade compounds with varying kinetics, which is attributed to the production of enzymes that degrade lignin, particularly laccase and MnP, which are excreted in amounts that increase during lignolytic activity (Hobbs, 2004; Lin et al., 2008; Lau et al., 2004; Sedarati et al., 2003). In this sense, it is reported that at an initial concentration of 50 mg L-1, T. versicolor showed a complete removal of benzene after 14 h of exposure and by increasing it to 300 mg L-1 the removal took 42 h. Concentrations of 50 ml L-1 of toluene were removed in 4 and 36 h when increased to 300 ml L-1 (Demir, 2004). On the other hand, Megan et al. (2010) reported that this species degrades trifluralin and dieldrin in maximum amounts of 24.6 and 115 ml L-1 as well as the mixture of both in a concentration of 32.3 ml L-1 in a time of 480 h. Within this degradation process, T. versicolor excretes an enzyme that acts as a kind of Mn(II)-dependent peroxidase (Johansson and Nyman, 1987). MnP is able to catalyze the oxidation of phenanthrene, fluorene and other phenolic compounds (Collins and Dobson, 1996) suggesting that this enzyme is capable of degrading a large number of phenolic compounds as well as the LiP; however, the laccase enzyme, which is also present in this fungus, does not degrade phenanthrene and fluorene, but does have activity over a wide range of polyphenols (Collins and Dobson, 1996; Majcherczyk et al., 1998). Obtained data by Pozdnyakova et al. (2018) support the hypothesis that, the degree of degradation of the phenolic compounds can depend of the composition of the extracelular ligninolytic complex of strain used. In this regard, for the correct selection of fungal strains for remediation, it is necessary to study the activity of the basic ligninolytic enzymes. This will allow the development of a technological process to avoid the accumulation of toxic substances in the treated objects. On the basis of their degradative properties and the composition of the ligninolytic enzyme system, T. versicolor can be employed for detailed study and for the development of technologies of remediation of contaminated environments. The present research was carried out to determine the degradation kinetics of T. versicolor natural strain Mo008 as well as to know the enzimatic complex involved in the degradation process over acid 2,4-dichlorophenoxyacetic (2,4-D) and atrazine.
Strain
In the present investigation, the native strain of T. versicolor (L.:Fr.) Pilát isolated from Crescentia alata in Jojutla, Mor., México was used (Mo008).
Detection of 2,4-D in liquid medium
To extract 2,4-D from a solution, a methodology proposed by Anonimo (1995) was used, the residues were collected from a steam rod at 50°C and 50 rpm and 50 mL of ethyl alcohol (95%) was added to concentrate the 2,4-D residues.
Calibration curve of 2,4-D, 2,4-D amine and atrazine
This was obtained using the methodology of Bhoi (2011), for which a standard solution of ethylic alcohol (95%) was prepared with 100 mg L-1 of 2,4-D analytical (SIGMA®) and 2,4-D amine (Hierbamina®). A solution in distilled water of 10 mg L-1 of atrazine was prepared too and from each standard solutions aliquot was taken to prepare a solution of 0, 2, 5, 10, 15 and 20 mg L-1 from both sources of 2,4-D and 0, 0.5, 1, 2, 3, 4, 5 and 10 mg L-1 of atrazine. The absorbance was measured in a spectrophotometer (Genesys 10uv®) at 287 nm for 2,4-D and 220 nm for atrazine.
Bioreactors for biodegradation of 2,4-D, 2,4-D amine and atrazine
PVC containers with 15 L-1 solution with 1000 ppm of 2,4-D (SIGMA®), 2,4-D amine (Herbamina®) and the mixture of 2,4-D amine plus atrazine (Atrazine 90®) at pH 5.0 and 25°C were used as bioreactors. Filters with steril polyurethane fiber support and 10 g of mycelium of T. versicolor strain Mo008 and previously developed for 15 days in malta-agar culture medium were constructed and adapted to each biorreactor. The strain was exposed for 1000 h at continuous flow. Every 50 h, 3 samples of 100 ml were collected from each reactor in amber glass bottles and stored at 0°C.
Degradation kinetics
The degradation kinetics was obtained by estimating yield of biomass-substrate and consumption global velocity (CGV) based on the methodology presented by Rubio (2005) and Marron et al. (2006). The yield represents the amount of mycelium produced by the fungus per each ppm consumed (Equation 1) and the CGV like decrease of the concentration of the substrate in a given time (Equation 2).
Where, Y is the yield; Xf is the final biomass; Xi is the initial biomass; Sf is the final concentration; Si is the initial concentration.
Where, CGV is the consumption global velocity; Is is the initial substrate; Sf is the final substrate; Tt is the total time.
Enzymatic analysis
Preparation of enzyme expression fluid
An enzyme expression fluid was prepared according to the methodology of Penninckx and Jiménez (1996) and Jiménez et al. (1999) which contained 1% of glucose, 0.02% of ammonium tartrate, 0.05% of MgSO4.7H2O, 0.01% of CaCl2.12H2O, 0.05% of Tween 80, 0.1 mg of thiamine chloride, veratryl alcohol 2.5 mM, 70 ml L-1 of trace elements (which contains per liter: 1.5 g of nitriloacetic acid, 3 g of MgSO4.5H2O, 1 g of NaCl, 0.1 g of FeSO4.7H2O, 0.1 g of CaCl2.2H2O, 0.1 g of ZnSO4.7H2O, 0.01 g of CuSO4.5H2O, 0.01 g of ALK (SO4).12H2O, 0.01 g of HBO3 and 0.01 g of Na2MoO4.2H2O) and brought to pH 4.5 with sodium tartrate buffer. In 500 ml Erlenmeyer flasks, 70 ml of expression fluid was added and sterilized at 120°C for 15 min. A gram of mycelium of the Mo008 strain was added with 15 days of development (Jiménez et al., 1997) and it was placed on a shaker at 150 rpm at room temperature. At 48 h, 40 ppm of Mn+2 was added and held for an additional 48 h on the shaker.
Preparation of extracts
Mycelium (1 g-1) developed in the enzyme expression fluid was centrifuged (Hermle®) at 5000 rpm for 5 min (Pennickx and Jimenez, 1996). The supernatant was stored at -15°C in total darkness for further use. This same methodology was used to obtain extracts of the mycelium developed in the filters of the bioreactors with the solution of 1000 ppm of 2,4-D amine after 1000 h of exposure in continuous flow.
Conditioning of extracts
The temperature was elevated to 20°C to each extracts, as each one of them was used for the detection of the enzymatic activity.
Calibration curves for the substrates used
To measure the enzymatic activity of LiP, a calibration curve was performed for concentrations from 0 to 5 mM of veratril alcohol in a sodium tartrate buffer 50 mM pH 4.5 and 25°C. For the case of Manganese Peroxidasse (MnP), the curve was estimated for concentrations from 0 to 0.5 mM of phenol red [0.01%] in sodium succinate buffer 0.1 M and for laccase, concentrations from 0 to 9 mM of ABTS 5 mM in 0.1 M sodium acetate buffer pH 5.0. The absorbance was recorded at 310, 610 and 420 nm for each substrate, respectively. From the absorbance data recorded for each of the enzymes studied, the concentration of the substrate (mM) was estimated from the equation obtained from the calibration curve, the extinction coefficient and the enzymatic activity (mM/min/ml). From these results, the Michaelis-Menten kinetic model was obtained as well as the Lineweaver-Burk representation to obtain the maximum reaction velocity (Vm) and the concentration of the substrate for which the reaction speed is half the speed maximum (km)
Determination of enzymatic activity
The activity of LiP was measured using the method proposed by Tien and Kirk (1983), which records the increase in absorbance at 310 nm due to the oxidation of veratril alcohol to veratryl aldehyde. The reaction employs 2.2 ml of sodium tartrate buffer (50 mM, pH 4.5 at 25°C), 40 μl veratryl alcohol (2 mM) and 240 μl of the culture extract. The reaction is initiated by adding 20 μl H2O2 (0.2 mM) and the absorbance is measured at 310 nm. The activity of MnP was recorded following the methodology described by Glenn and Gold (1985). This method is based on the oxidation of Mn (II) to Mn (III) and uses as a substrate 2.5 ml of red phenol (0.01%) and MnSO4 (0.1 mM) in sodium succinate buffer (0.1 M). The reaction mixture contains 2.5 ml of substrate and 200 μl of the culture extract. The reaction is started by adding 20 μl of H2O2 (0.1 mM), after incubation for 2 min at 30°C and the absorbance WAS measured at 610 nm. On the other hand, laccase activity was recorded using the method described by Bourbonnais et al., (1995) which records the oxidation of 2,2'-azino-bis (3-ethylbenzothiazoline-6-sulphonic acid) (ABTS substrate, 5 mM). After dissolving the substrate in 2.5 ml of sodium acetate buffer (0.1 M, pH 5.0), 100 μl of the extract was added, the mixture was incubated for 2 min at 30°C and after that time the absorbance was recorded at 420 nm. For all cases, the absorbance was measured at intervals of 30 s for 5 min.
All analysis had three replicates and the variance analysis and Tukey test (a=0.01) was made with SAS® Stadistical Software.
Calibration curve for detection of analytical 2,4-D, 2,4-D amine and atrazine
The obtained curves are represented by a linear model with a correlation coefficient that allows to affirm that the detected absorbance is a direct function of concentration in each one of the samples (Figure 1).
Degradation kinetics of T. versicolor
Specifically, 2,4-D is one of the herbiides most used to control boradleaf weeds; the active ingredient has chronic health effects, and lethal on the soil because it has a residual effect. It has been shown that there are microorganisms that have the capacity to degrade these phenolic compounds, which is called bioremediation (Akintui et al., 2015). The degradation kinetics in bioreactors was determined according to the yield of the biomass-substrate, which expresses the increase of biomass of T. versicolor for each ppm of consumed substrate (Rubio, 2005), with a response differentiated by the source 2,4 -D (Table 1) as well as the mixture of this with atrazine (Figure 2), confirming a differentiation in the yield for each substrate (Figure 3A), which coincides with Field et al. (1992), Bhalerao and Puranik (2007) and Siddique et al. (2003) who attribute the development of filamentous fungi on different substrates which directly influence respiration and biomass as the only carbon source. Stamatiu et al. (2015) found that some strains exposed to chlorpyrifos and endosulfan outgrow the mycelial development of their respective controls after a period of inhibition (3 to 16 days). Phanerochaete chrysosporium, P. ostreatus, Bjerkandera adusta and T. versicolor are the most commonly used for the degradation of such compounds owing to their production of ligninolytic enzymes such as LiP, MnP and laccase (Pozdnyakova et al., 2018).
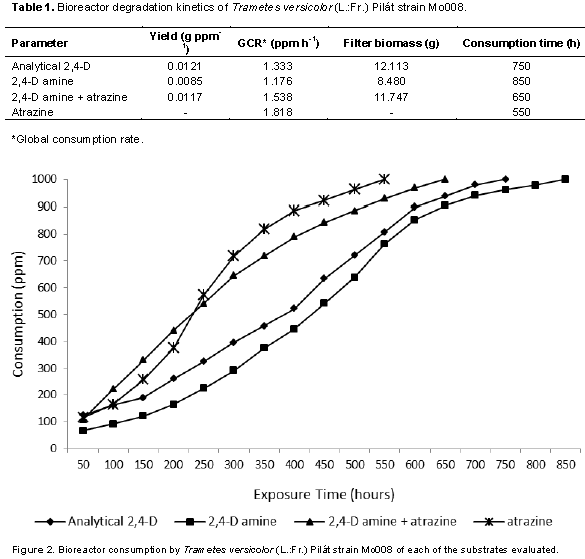
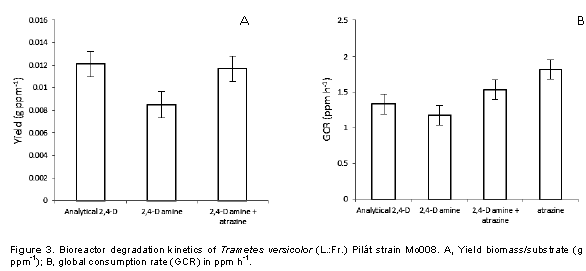
On the other hand, Dutta et al. (2010) reported a stimulus in basal respiration and in microbial biomass when chlorpyrifos like substrate is used in comparison with the control, whereas Das and Mukherjee (2000) and Eisenhauer et al. (2009) mentioned that soil micro-organisms treated with organophosphorus and organochlorine pesticides increase their population. The global consumption rate (GCR) that expresses the amount of ppm degraded by T. versicolor per hour indicates that when 2,4-D amine is mixed with atrazine, it tends to be higher (Figure 3B) than when only analytical 2,4-D and amine salt are used, and the studied strain is more efficient to degrade atrazine than 2,4-D; the 1000 ppm added to the bioreactor was degraded in a time of 550 h with a GCR of 1,818. These results indicate that T. versicolor (Mo008) can be used as a biodegradation tool for this type of compounds in high concentrations (1000 ppm) as compared to what has been reported by Demir (2004), Wu and Yu (2006), Srivastava et al. (2008), Kumar et al. (2009), Megan et al. (2010) and Pozdnyakova (2018).
Chlorophenols are the most common organic compounds which are widely used in agricultural industry and public health. The most important pollution sources of chlorophenols are the waste waters from pesticide, paint, solvent, pharmaceutics, wood-preserving chemicals, paper and pulp industries and water disinfecting processes. Because these types of products are toxic, resistant to microbial degradation and can accumulate in the food chain, many countries have restricted or banned their production or use and have designated them as priority pollutants in their own list of hazardous wastes (Ruiying and Jianlong, 2007). T. versicolor has the ability to produce different enzymes that can degrade recalcitrant compounds, for this reason, it is used in biotechnology for bioremediation studies, likewise it can be use to remove Cu2+, Pb2+, Cd2+, Ni2+ and Zn2+ from organic textile dyes (Congeevaram et al., 2007; Fu and Viraraghavan, 2001; Baldrian, 2003; BayramoÄŸlu et al., 2003; Solis et al., 2015).
Calibration curves for the measurement of enzymatic activity in degradation of 2,4-D amine and the mixture of 2,4-D amine and atrazine
The calibration curves for each extracts used to detect enzymatic activity are represented by linear models with correlation coefficients above 0.99 as shown in Figure 4.
Enzymatic activity in the degradation of 2,4-D amine and 2,4-D amine plus atrazine
The complex enzymatic involved in the degradation of phenolic compounds (LiP and MnP) was detected in the micelium extracts exposed to 2,4-D amine as well as to the mixture of this with atrazine in continuous flow bioreactor and phenol oxidases or polyphenolic oxidases as laccase. For each enzyme detected, the mycelial extract developed in the enzymatic expression fluid (Control) showed a higher affinity than the mycelial extract developed in the bioreactor (Table 2); however, the development of T. versicolor in 2,4-D amine as well as in the mixture with atrazine indicate that this fungus produces the enzymatic complex involved for the degradation of both compounds (Karam and Nicell, 1997; Duran, 1997; Duran and Esposito, 2000) and that the degradation process was greater when the 2,4-D amine was mixed with atrazine than when it was exposed individually due to the values of speed maximum detected in the last process (Figure 5). Oxidative enzymes play an important role in the decontamination of effluents and soil and T. versicolor possesses a complex mechanism involving enzymes that attack lignin directly, like LiP, MnP and laccase (Córdoba et al., 2012); these enzymes can be used in the management of environmental pollutants such as textile effluents, pulp effluents, organochloride agrochemicals and crude oil residues (Kantharaj, 2017) which were detected in the present study. The genus Trametes, which belongs to the White-rot fungi, is assumed to be one of the main producers of laccases. T. versicolor produces laccase and MnP as major lignolytic enzymes; however, and in a particular case, the role of these enzymes in decolorization of azo dyes is not yet clear. Laccase and/or MnP activities in culture fíltrate of T. versicolor were not able to decolorize azo dyes, thus indicating a role of other enzymes or cell-bound components in azo dye degradation (Swamy and Ramsay, 1999). The use of enzymes for the treatment of contaminants has been proposed by numerous researchers, however, most of these investigations are focused on demonstrating the decrease of several contaminantsby biological organisms that produce the enzyme complex studied as a basis for future remediation engineering projects, a fundamental step for its implementation (Gianfreda et al., 1999; Heitzer, 1993; Aitken, 1993; Heitzer, 1998). This enzyme complex is mainly composed of the so-called lignolytic enzymes that include laccasa, MnP and LiP. These enzymes catalyze the oxidation of lignin, but its non-specific nature allows the degradation of xenobiotic compounds with a chemical structure similar to lignin (Dominguez et al., 2010). T. versicolor (L.:Fr.) Pilát is a basidiomycete that produces extracellular enzymes that participate in the degradation of lignin in a nonspecific way (García and Torres, 2003) and has the possibility of using it in a broad spectrum of recalcitrant substances that show structural similarities with lignin. However, Katharaj (2017) reports that MnP degrades the lignin mainly by attacking phenolic lignin component. In the presence of H2O2, this enzyme oxidizes the phenolic structures by converting Mn2+ to Mn3+. Oxalato and malonate are the mediators that produce carbon centered radicals, peroxyl radicals and superoxide radicals which improve the effective lignin-degradings system.
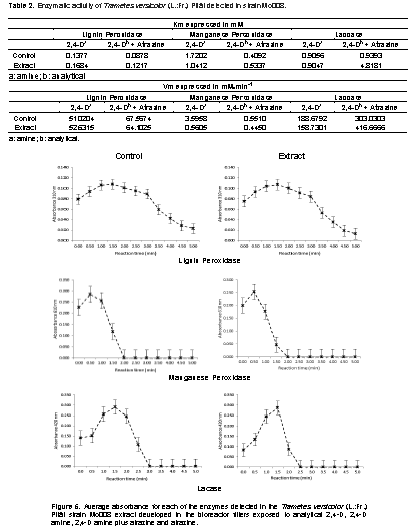
MnP is an essential component to certain basidiomycetes and some wood decaying white-rot fungi, which secrete MnP in several forms into their environment. Laccases are the copper containing polyphenol oxidases which enable degradation of phenolic compounds and also reduce molecular oxygen to wáter (Arora et al., 2010; Divya et al., 2013). Laccases oxidize the phenolic units in lignin to phenoxy radicals, which can lead to aryl-C cleavage (Kawai et al., 1988). Laccase can also oxidize non-phenolic substrates in the presence of certain auxiliary substrates (Call and Muncke, 1997; Kantharaj et al., 2017). Compounds such as chloro-phenols, polychlorinated biphenyls (PCBs), DDTs, dioxins, polycyclic aromatic hydrocarbons (PAH's), alkyl halides, nitrotoluenes, azo dyes and polymers can be modified or degraded to varying extents (Linn et al., 1993; García, 2001; Raimbault, 1998; Gold and Allic, 1993; Karam and Nicell, 1997; Kuhad et al., 1997; Majcherezyk et al., 1998); in addition, 2,4-D amine and atrazine can be efficiently degraded by this species as shown in this investigation.
The enzymes produced by the fungi T. versicolor was also employed for the detoxification of aromatic pollutants like agrochemicals and industrial effuents (Kantharaj et al., 2017). In recent years, the presence of micropollutants such as pharmaceuticals, industrial chemicals, personal care products and many other chemical compounds in the aquatic enviroment have become a significant problem worldwide (Doruk et al., 2018). Sahadevan et al. (2016) reported that lignin-degrading enzymes, LiP, MnP and laccase can be used like appropriate biological substitute to treat highly alkaline effluents like pulp, paper industry and waste water and various non-steroidal, anti- inflammatory drugs such as naproxen, ketoprofen and ibuprofen (Marco et al., 2009; Marco et al., 2010a, 2010b).
T. versicolor (Natural strain Mo008) efficiently degraded atrazine and 2,4-D, being more efficient in the degradation of 1000 ppm of atrazine (550 h) than of 2,4-D (850 h); however, the mixture of both herbicides was consumed in a time of 650 h. Likewise, in the biodegradation of both, alone and as a mixture, the studied strain presented the activity of the enzymatic complex which was composed of laccase, LiP and MnP which have an important role in the degradation of phenolic compounds and other recalcitrants wastes due to their similiar structure to lignin. T. versicolor can be employed as a bioremediation tool for water contaminated with acid 2,4-D and atrazine and others phenolics compounds as well as pharmaceuticals wastes.
The authors thank the National Council of Science and Technology of Mexico (CONACyT), Department of Agricultural Sciences of the Autonomous University of Morelos State and Antonio Segura Miranda, Researcher of the Department of Agricultural Parasitology of the Autonomous University of Chapingo for the facilities granted to carry out the present research.
The authors have not declared any conflict of interests in this research and its publication.
REFERENCES
Aitken MD (1993). Waste, treatment applications of enzymes. Opportunities and obstacles. Chemical Engineering Journal 52:B49.
Crossref
|
|
Akintui M, Alcántara H, Alva A, Castillo H, Hueyán J, Llenque L (2015). Pseudomonas flourescens de suelos agrícolas degradadora del herbicida ácido 2,4 Diclorofenoxiacético. Rebiolest 3(2):49.
|
|
|
Anónimo (1995). 2,4-D-Pesticide Fact Sheet. U.S. Department of Agriculture. Forest Service. s/p.
|
|
|
Arora DS, Sharma RK (2010). Ligninolytic fungal laccases and their biotechnological applications. Applied Biochemistry and Bioltechnology 160(6):1760-1788.
Crossref
|
|
|
Baldrian P (2003). Interactions of heavy metlas with white rot fungi. Enzyme and Microbial technology 32(1):78-91.
Crossref
|
|
|
BayramoÄŸlu G, Bektas S, Arica MY (2003). Biosorption of beavy metal ions on immobilized white-rot fungus Trametes versicolor. Journal of Hazardous Materials 10(13):285-300.
Crossref
|
|
|
Betancur CB, Pino N, Pe-uela GA, Cardona GS (2013). Biorremediación de suelo contaminado con pesticidas: caso DDT. Gestión y Ambiente 16(3):119-135.
|
|
|
Bhalerao TS, Puranik PR (2007). Biodegradation of organochlorine pesticide, endosulfan, by a fungal soil isolate, Aspergillus niger. International Biodeterioration Biodegradation 59(4):315-321.
Crossref
|
|
|
Bhoi AG (2011). Estimation of herbicide 2,4-D in commercial preparations using spectrophotometry.
|
|
|
Buswell JA, Oider E (1987). Lignin degradation. Critical Reviews in Biotechnology 6:1-60.
Crossref
|
|
|
Bourbonnais R, Paice MG, Reid ID, Lanthier P, Yaguchi M (1995). Lignin oxidation by laccase isozymes from T.versicolor and role of the mediator ABTS in kraft lignin depolymerization. Applied and Environmental Microbiology 61:1876-1880.
|
|
|
Call HP, Muncke I (1997). History, overview and applicaations of mediated lignolytic systems, especially laccase-mediator systems (lignozyme (R)-process). Journal of Biotechnology 53:163-202.
Crossref
|
|
|
Collins PJ, Dobson ADW (1996). Oxidation of fluorine and phenathrene by Mn(II) dependent peroxidasa activity in whole cultures of Trametes (Coriolus) versicolor. Biotechnology Letters 18(7):801-804.
Crossref
|
|
|
Congeevaram S, Dhanarani S, Park J, Dexilin M, Thamaraiselvi, K (2007). Biosorption of chromium and nickel by heavy metal resistant fungal and bacterial isolates. Journal of Hazardous Materials 146:270-277.
Crossref
|
|
|
Córdoba M, Kett A, Ríos HA (2012). Biotechnological applications and potential uses of the mushroom Trametes versicolor. Vitae 9(1):70-76.
|
|
|
Das AC, Mukherjee D (2000). Soil application of insecticides influences microorganism and plant nutrients. Applied Soil Ecology 14:55-62.
Crossref
|
|
|
Demir G (2004). Degradation of toluene and benzene by Trametes versicolor Journal of Environmental Biology 25:19-25.
|
|
|
Divya LM, Prasanth GK, Sadasian C (2013). Isolation of a salt tolerant laccase secreting strain of Trichoderma sp NFCCI-2745 and optimization of culture conditions and assessing its effectiveness in treating saliine phenolic effuents. Journal of Environmental Sciences. 25(12):2410-2416.
Crossref
|
|
|
Domínguez O, Ramos LM, Manzano AM, Sánchez MI, Sánchez A, Torres G, Arguelles J, Romeu A, Palacios J, Dierksmeier G, Guerra G (2010). Biodegradación de DDT por dos cepas nativas de hongos de la podredumbre blanca. Ciencias Biológicas 41:1-12.
|
|
|
Duran N (1997). Siderophores on wood degradation: reduction of molecular oxygen to hydroxyl radical and ferric iron to ferrous iron, in: Melo EHM, Pimentel MCB (Eds). Proccedings of the Fourth Brazilian Symposium on Chemical Lignins other Wood Comp. Brazil, 5:164. Chemical Abstract 126:100752.
|
|
|
Duran N, Esposito E (2000). Potencial apllications of oxidative enzymes and phenoloxidase-like compounds in wastewater and soil treatment: a review. Applied Catalysis B: Environmental 28 (2):83-99.
Crossref
|
|
|
Duran N, Esposito E (2000). Potencial apllications of oxidative enzymes and phenoloxidase-like compounds in wastewater and soil treatment: a review. Applied Catalysis B: Environmental 28 (2):83-99.
Crossref
|
|
|
Doruk AY, Göker H, Cihangir N (2018). Biodegradation of diclofenac with fungal strains. Archives of Environmental Protection 44(1):55-62.
|
|
|
Dutta M, Sardar D, Pal R, Kole RK (2010). Effect of chlorpyrifos on microbial biomass and activities in tropical clay loam soil. Environmental Monitoring and Assessment 160:385-391.
Crossref
|
|
|
Eisenhauer N, Klier M, Partsch S, Sabais CWA, Scherber C, Weisser WW, Scheu S (2009). No interactive effects of pesticides and plant diversity on soil microbial biomass and respiration. Applied Soil Ecology 42:31-36.
Crossref
|
|
|
Field JA, De Jong E, Feijoo CG, De Bont JAM (1992). Biodegradation of polycyclic aromatic hydrocarbons by new isolates of white rot fungi. Applied and environmental microbiology 58:2219-2226.
|
|
|
Fu Y, Viraraghavan T (2001). Fungal decolorization of dye wastewaters: a review. Bioresource Technology 79:251-262.
Crossref
|
|
|
García A (2001). Producción de enzimas lignolíticas por Basidiomycetes mediante la técnica de fermentación en sustrato sólido. Tesis. Univ. Industrial de Santander. Escuela de Química. Bucaramanga.
|
|
|
García TAM, Torres RG (2003). Producción de enzimas lignolíticas por basidiomicetes mediante la técnica de fermentación en sustrato sólido. Rev. Colombiana de Biotecnología 5(1):56-64.
|
|
|
Gold MH, Wariishi H, Valli K (1989). Extracellular peroxidasas involved in lignin degradation by the white rot basidiomycete Phanerochaette chrysosporium. ACS Symposium Series 389:127-140.
|
|
|
Gold MH, Allic M (1993). Molecular biology of the lignin-degrading basidiomycete Phanerochaette chrysosporium. Reviews Microbiology 57:605-622.
|
|
|
Gómez A, Kong JY, Parhar K (2005). Ceramide-1-phosphate promotes cell survival through activation of the phosphatidylinositol 3-kinase/protein kinase B pathway. FEBS Letters 579(17):3744–3750.
Crossref
|
|
|
Guo L, Jury WA, Wagenet RJ, Flury M (2000). Dependence of pesticide degradation on sorption: nonequilibrium model and application to soil reactors. Journal of Contaminant Hydrology 43(1):45-62.
Crossref
|
|
|
Heitzer GS (1993). Monitoring the efficacy of bioremediation. Trends Biotechnology 11:334-338.
Crossref
|
|
|
Heitzer GS (1998). Microbiological, molecular and biochemical methods for environmental risk analysis and bioprocess evaluation. Journal of Microbiological Methods 32:89-93.
|
|
|
Hobb C (2004). Medicinal value of Turkey tail fungus Trametes versicolor (L.:Fr.) Pilát (Aphyllophoromycetidae). International Journal of Medicinal Mushrooms 6(3):24-30.
|
|
|
Jiménez GA, Penninckx MJ, Lejeune R (1997). The relationship between pellet size and production of Mn(II) peroxidasa by Phanerochaette chrysosporium in submerged culture. Enzyme Microbial Technology 21: 537-542.
Crossref
|
|
|
Jiménez TGA, Mejía GAI, López OBL (1999). Actividad de las enzimas lingninolíticas del Phanerochaette chrysosporium y su variación con la adición de Mn+2. Rev. Acad. Colomb. Cienc. 23(89):587-594.
|
|
|
Johansson T, Nyman PO (1987). A manganese (II)-dependent extracellular peroxidase from the white-rot fungus Trametes versicolor. Acta Chemica Scandinavica B 41:762-765.
Crossref
|
|
|
Kantharaj P, Boobalan B, Sooriamuthu S, Mani R (2017). Lignocellulose degrading enzymes from fungi and their industrial applications. International Journal of Current Research 9(21):1-12.
|
|
|
Karam, J, Nicell J (1997). Potencial application of enzymes in waste treatment. Journal of Chemical Technology Biotechnology 69:141-153.
Crossref
|
|
|
Kawai S, Umezawa T, Higuchi T (1988). Degradation mechanisms of pehnolic B-1 lignin substructure model compounds by laccase of Coriolus versicolor. Archieves of Biochemistry Biophysics 262(1):99-110.
Crossref
|
|
|
Kirk K, Farrell R (1987). Enzymatic combustion. The microbial degradation of lignin. Annual Reviews of Microbiology 41:465-505.
Crossref
|
|
|
Kuhad RC, Sing A, Eriksson KEL (1997). In: Biotechnology in the pulp and paper industry (Ed. K.E.L. Eriksson). Springer Verlag, Heidelberg, Germany pp. 45-125.
Crossref
|
|
|
Kumar NS, Boddu VM, Krishnaiah A (2009). Biosoption of phenolic compounds by Trametes versicolor polyporus fungus. Adsorpton Science Technology 27(1):31-46.
Crossref
|
|
|
Lau CB1, Ho CY, Kim CF, Leung KN, Fung KP, Tse TF, Chan HH, Chow MS. (2004). Cytotoxic activities of Coriolus versicolor (Yunzhi) extract on human leukemia and lymphoma cells by induction of apoptosis. Life Sciences 75(7):797-808.
Crossref
|
|
|
Linn DM, Carski TH, Brusseau ML, Chang FH (1993). Sorption and degradation of pesticides and organic chemicals in soil. Soil Science Society of America, Madison WI, P 260.
|
|
|
Lin F, Lai Y, Yu H, Che N, Chang C (2008). Effects of Lycium barbarum extract on production and immunomodulatory activity of the extracellulalr polysaccharopeptides from submerged fermentation culture of Coriolus versicolor. Food Chemistry 110(2):446-453.
Crossref
|
|
|
Megan N, Fragoeiro S, Bastos C (2010). Environmental factors and bioremediation of xenobiotics using white rot fungi. Mycrobiology 38(4):238-248.
Crossref
|
|
|
Majcherezyk A, Johannes C, Hüttermann A (1998). Oxidation of polycyclic aromatic hydrocarbons (PAH) by laccase of Trametes versicolor. Enzyme Microbial Technology 22:335-341.
Crossref
|
|
|
Marco UE, Pérez TM, Vincet T, Caminal G (2009). Ability of white-rot fungi to remove selected pharmaceuticals and identification of degradation products of ibuprofen by Trametes versicolor. Chemosphere 74:765-772.
Crossref
|
|
|
Marco UE, Pérez TM, Blánquez P, Vicenta T, Caminalc G (2010a). Biodegradation of the analgesic naproxen by Trametes versicolor and identification of intermediates using HPLC-DAD-MS and NMR. Bioresourse Technology 1:2159-2166.
Crossref
|
|
|
Marco UE, Pérez TM, Cruz MC, Caminal G, Vincent T (2010b). White-rot fungus-mediated degradation of the analgesic ketoprofen and identification of intermiediates by HPLC-DAD-MS and NMR. Chemosphere 78:474-481.
Crossref
|
|
|
Marrón MNE, Ruíz OC, Rubio GC, Juárez R, Galíndez MCJ (2006). 2,4-D degrading bacterial consortium isolation, kinetic characterization in batch and continuous culture and application of bioaugmenting an activated sludge microbial community. Process Biochemistry 41:1521-1528.
|
|
|
Nyanhongo GS, Gübitz G, Sukyai P, Leitner C, Haltrich D, Ludwig R (2007). Oxidoreductases from Trametes spp. in biotechnology: A wealth of catalytic activity. Food Technology Biotechnology 45(3):250-268.
|
|
|
Penninckx M, Jiménez GA (1996). Transformación Microbiológica de la Biomasa. Curso teórico – práctico. Univsersité Libre de Bruxelles and Universidad de Antioquia. Medellín, Abril 22-27.
|
|
|
Peralta ZP, Esposito E, Pelegrini R, Groto R, Reyes J, Durán N (1998). Effluent treatment of pulp and paper, and textile industries using immobilized horseradish peroxidasa. Environmental Technology 19:55-63.
Crossref
|
|
|
Pozdnyakova NN, Balandina SA, Dubrovskaya EV, Golubev CN, Turkovskaya OV (2018). Ligninolytic basidiomycetes as promising organisms for the mycoremediation of PAH-contaminated Environments. IOP Conf. Earth Environmental Science 107:012071.
|
|
|
Raimbault M (1998). General and microbiological aspects of solid substrates fermentation. Electronic Journal of Biotechnology 1(3):1-17.
Crossref
|
|
|
Rothschild N, Levkowitz A, Hadar Y, Dosoretz C (1999). Manganese deficiency can replace high oxygen levels needed for lignin peroxidasa formation by Phanerochaette chrysosporium. Applied Environmental Microbiology 65(2):483-488.
|
|
|
Rubio GC (2005). Aislamiento de un consorcio bacteriano en un quimiostato alimentado con ácido 2,4-diclorofenoxiacético (2,4-D) y evaluación cinética de sus integrantes sobre diversos compuestos organoclorados. Tesis de Maestría. Escuela Nacional de Ciencias Biológicas. Instituto Politécnico Nacional. México.
|
|
|
Ruiying G, Jianlong W (2007). Effects of pH and temperature on isotherm parameters of chlorophenols biosorption to anaerobic granular sludge. Journal of Hazardous Materials 145(3):398-403.
Crossref
|
|
|
Sedarati MR, Kesshavarz T, Leontievsky AA, Evans CS (2003). Transformation of high concentrations of chlorophenols by the white-rot basidiomycete Trametes versicolor immobilized on nylon mesh. Electronic Journal of Biotechnology 6(2):104-114.
|
|
|
Siddique T, Okeke BC, Arshhad M, Frankenberger WT Jr (2003). Enrichment and isolation of endosulfan-degrading microorganisms. Journal of Environmental Quality 3:47-54.
Crossref
|
|
|
Solis PJ, Santana M, Aguilar UMG, Cavazos GA, Serrano NJ, Gómez H, Aguilar UB (2015). Ability of Phanerochaete chrysosporium and Trametes versicolor to remove Zn2+, Cr3+, Pb2+ metal ions. Terra Latinoamericana 33:189-198.
|
|
|
Srivastava VC, Prasad B, Mishra IM, Mall D, Swamy MM (2008). Prediction of Breakthrough Curves for Sorptive Removal of Phenol Bagasse Fly Ash Packed Bed. Industrial Engineering Chemistry Research 47(5):1603-1613.
Crossref
|
|
|
Stamatiu SK, Alarcón A, Ferrera CR, Nava DC, Sánchez EJ, Cruz SJS, Castillo M (2015). Tolerancia de hongos filamentosos a endosulfán, clorpirifós y clrotoalonil en condiciones in vitro. Revista internacional de contaminación ambiental 31(1):23-37.
|
|
|
Swamy J, Ramsay JA (1999). Effects of Mn2+ and NH4+ concentrations on laccase and manganese peroxidasa production and Amarath decoloration by Trametes versicolor. Applied Microbiology and Biotechnology 51(3):391-396.
Crossref
|
|
|
Szklarz GD, Antibus RK, Sinsabaugh RL, Linkins AE (1989). Production of phenol oxidases and peroxidases by wood-rotting fungi. Mycologia 81(2):234-240.
Crossref
|
|
|
Tien M, Kirk TK (1983). Lignin degrading enzyme from the hymenomycete Phanerochaette chrysosporium Burds. Science 221:661-663.
Crossref
|
|
|
Tortella GR, Rubilar O, Gianfreda L, Valenzuela E, Diez MC (2008). Enzymatic characterization of Chilean native wood-rotting fungi for potential use in biotechnology applications. World Journal Microbiology Biotechnology (Submitted, WIBI 3338).
Crossref
|
|
|
Ullah MA, Kadhim H, Rastall RA, Evans CS (2000). Evaluation of solid substrates for enzymes production by Coryolus versicolor, for use in bioremediation of chlorophenols in aqueos effluents. Applied Microbiology Biotechnology 54:832-837.
Crossref
|
|
|
Wu J, Yu Q (2006). Biosorption of phenol and chlorophenols from aqueous solutions by fungal mycelia. Process Biochemistry 41(1):44-49.
Crossref
|
|