ABSTRACT
Secondary metabolites may play a number of roles, among them, is the activation of plant defense mechanisms, such as phytoalexins and the synthesis of antioxidative enzymes. This study aimed to test the eliciting activity of phytoalexins of Lippia sidoides Cham. and six endophytic fungi associated with it and to verify the synthesis of the enzymes, as a response to the antioxidative and antifungal system. The assays were performed using spectrophotometric methods. Of the concentrations of essential oil used, 7.5 mg mL-1 was 66.48% higher than the Acorda® positive control for the induction of glyceollin. The endophytic fungus, Verticillium sp. showed the best result for 3-deoxythianocyanidine production. Excellent results were demonstrated by the LS-14 fungus for superoxide dismutase enzyme activity (130 U g-1 E-1 min-1); by L. sidoides for the activity of ascorbate peroxidase enzyme (3821 μmol ASA g-1 E-1 min-1); by L. sidoides for the activity of catalase enzyme (2429 μmol H2O2 g-1 E-1 min-1); by Fusarium sp. 2 for the activity of phenol peroxidase (87 μmol H2O2 g-1 E-1 min-1) and by Fusarium sp. 1 for the activity of chitinase (0.214 U min-1). Both the essential oil of L. sidoides and the extracts of the endophytic fungi had a positive response to the induction of phytoalexin and presence of enzymes related to antioxidative activity were verified and shown.
Key words: Catalase, superoxide dismutase, peroxidase phenols, ascorbate peroxidase, chitinase.
Abbreviation:
SOD, Superoxide dismutase; QUIT, chitinase; APX, ascorbate peroxidase; POX, phenol peroxidase; CAT, catalase; Ver, Verticillium sp.; Col, Colletotrichum sp.; Fus 1, Fusarium sp. 1; Fus 2, Fusarium sp. 2; Lip, Lippia sidoides; LS-6, code of the unidentified fungus; LS-14, code of the unidentified fungus; ROS, reactive oxygen species.
With increased productivity in agriculture and, consequently, increased use of agrochemicals, mainly related to the control of plant diseases, damage to the environment and living beings has appeared (Matiello and Bonaldo, 2013). However, the use of alternatives to conventional chemicals such as the use of plant extracts, fungi and essential oils for phytopathogenic control has been highlighted (Oliveira et al., 2016). Compounds such as kauralexins and zealexins in Zea mays and oryzalexin E in Oriza sativa have the potential to control phytopathogens, both by their direct fungitoxic action and by the ability to induce the accumulation of phytoalexins by activating plant defense metabolism (Venturoso et al., 2011). They are an interesting strategy in commercial agriculture, to develop biotechnological applications in the protection of plants (Ribera and Zuñiga, 2012). The tools could be used in the production of plants that express a greater amount of phytoalexins induced by both, phytoalexin elicitors or by genetic transformation (González-Lamothe et al., 2009). When plants come in contact with so-called elicitors, their defense system is activated, which induces resistance (Smith, 1996). The presence of these molecules, demonstrates antimicrobial activity with important actions in the protection of plants since they do not have an immune system like the vertebrates (Jenssen et al., 2006). They can also be called phytoalexins, which are synthesized in cytoplasmic inclusions near the site of attempted entry of the pathogen. In fungi, they disorganize the cellular content; the plasma membrane is ruptured and fungal enzymes are deactivated (Peiter-Beninca et al., 2008). Phytoalexin glyceollin in soybean (Glycine max L. Merr.) protects this legume. It is often reported in induction studies, for the use of its cotyledons, a tool used for studies involving the eliciting action because they are easily measured (Schwan-Estrada et al., 2000). Also, the use of etiolated mesocotyls of sorghum (Sorghum bicolor L. Moench21), inducing the production of phytoalexin 3-deoxythyanocyanidine promotes the increase in plant tissue resistance (Matiello and Bonaldo, 2013). It is also used in bioassays to test the elicitor effect of a treatment.Under stress conditions, the plants activate antioxidative defense mechanisms, such as resistance, in order to neutralize the cytotoxicity provoked by reactive oxygen species (ROS). In this system, the enzymes catalase (CAT), ascorbate peroxidase (APX), phenol peroxidase (POX) and superoxide dismutase (SOD) (Miller et al., 2010) are evidenced. Besides phytoalexins, these enzymes can have effect on plants resistance to pathogens.
Peroxidases catalyze the oxidation and eventual polymerization of hydroxycinnamic alcohol in the presence of hydrogen peroxide, which can lead to lignin. In addition, they participate in suberization, formation and cross-linking of cell wall components, auxin catabolism, senescence, protection against the attack of pathogens, insects and abiotic stressors (War et al., 2012). SODs remove superoxide radicals by preventing them from crossing the lipid bilayer of cell membranes (Hayakawa et al., 1984). Other types of enzymes, such as chitinases (QUIT), can also be produced by plants, animals, bacteria and fungi. They catalyze the hydrolysis of QUITin, a polysaccharide present in the cell wall of fungi, green algae and exoskeleton of crustaceans and insects. Thus, enzymatic lysis of the cell wall of phytopathogenic fungi through the action of extracellular chitinases becomes part of a biocontrol mechanism (Zarei et al., 2011). Among the elicitors, medicinal plant such as Lippia sidoides Cham., have antimicrobial properties and can be studied for their elicitor potential (Da Silva et al., 2013). Both antioxidative enzymes and chitinases, besides being produced by plants, can be synthesized by a wide variety of endophytic fungi, such as Beauveria, Lecanicillium, Metarhizium, Trichoderma (ST Leger and Wang, 2010; Sandhu et al., 2017), Nigrospora and Fusarium exerting multiple effects on host plants (Yang et al., 2016). Extracts of L. sidoides and their endophytic fungi may be used as a commercial product to reduce pest-related diseases. In this way, to identify the presence of enzymes that help in pest control, as well as the potential of phytoalexins induction is of extreme importance for the reduction of pesticides applied in organic plantations, mainly. If the extracts have this ability aforementioned, they probably can help crops of agricultural interest in their defense mechanisms against phytopathogens. Phytoalexins are considered important for plant resistance against pathogens. Plants are constantly attacked by many potential pathogens and respond by the activation of defense genes, the formation of ROS and the production of antimicrobial compounds. In this work, fresh leaves of L. sidoides and six endophytic fungi isolated from this plant were analyzed for the phytoalexin induction function, as well as the presence of enzymatic activity of SOD, APX, POX, CAT and QUIT in all extracts.
Plant material, steam distillation and sample preparation
L. sidoides originating from Ceará was collected in Gurupi (11°44’48” latitude S, 49°02’55” longitude W), Tocantins, Brazil. Taxonomic identification was confirmed by experts at the herbarium (Federal University of São João Del Rei, Brazil), where samples were deposited with reference number 8303. As described by Guimarães et al. (2008), L. sidoides essential oil was extracted from the leaves by steam distillation method in a Clevenger-modified apparatus, as described by Guimarães et al. (2008) and stored at 4°C until further analysis. For the elicitor potential tests, essential oil concentrations of 0.625 to 7.5 mg mL-1 were prepared by adding Tween 80 (0.03%) and sterilized distilled water for solubilization.
Preparation of endophytic fungi extracts
The fungi Cladosporium sp., Verticilium sp., LS-6 (unidentified), Colletotrichum sp., Fusarium sp. 1, Fusarium sp. 2 and LS-14 (unidentified) were isolated from L. sidoides leaves. The mycelia of the endophytes were inoculated in 200 mL of pre-fermentative medium (Jackson et al., 1993). The cultures were incubated initially for 48 h under constant stirring (120 rpm) at 30 ± 1°C. Then, the mycelial masses were aseptically collected by vacuum filtration and reinoculated in 400 mL of the Czapek fermentation medium (Alviano et al., 1992). After 6 days of incubation under constant stirring (120 rpm) at 30 ± 1°C, the culture fluids were separated from the mycelia masses by vacuum filtration and the extract was obtained according to the methodology of Dhankhar et al. (2012). The extractions were carried out using ethyl acetate in order to obtain the highest number of active antimicrobial substances. To obtain the positive control, the commercial systemic resistance activator, Biozyme® at 1.25 mg mL-1, was used and as the negative control, distilled water + Tween 80 was used.
Test for phytoalexin induction in soybean cotyledons
Soybean seeds (G. max), cultivar Monsoy 8644-IPRO (Intacta®), were disinfected for 10 min in 1% sodium hypochlorite and washed in distilled water. Subsequently, they were sown in two trays containing autoclaved sand (121°C and 1 atm for 20 min). The trays were left in a greenhouse for 10 days and the cotyledons were then highlighted for the trials (Stangarlin et al., 2010). The cotyledons were placed in 120 mm diameter Petri dishes, where each plate contained 3 cotyledons and 2 sheets of sterile filter paper and were moistened with sterile distilled water. Each cotyledon was cut into small fragments, which were treated with 100 μL of the samples to be tested. Each endophytic fungus extract was tested, being 16.3; 15.3; 82.3; 13.0; 13.4 and 14.0 mg mL-1 for the fungi Cladosporum sp., Verticilium sp., LS-6, Colletotrichum sp., Fusarium sp. 1, Fusarium sp. 2 and LS-14, respectively. In addition, five concentrations of the essential oil of L. sidoides were tested. The plates were incubated at 25 ± 1°C in the dark for 20 h. Then, the cotyledons were weighed and placed in Erlenmeyers containing 10 mL of sterile distilled water, which were left under orbital shaking (150 rpm) for 1 h for extraction of the pigments (Meinerz et al., 2008; Stangarlin et al., 2010). Finally, the cotyledons were removed from the Erlenmeyers and the absorbance of the supernatant was read in a spectrophotometer (BioSpectro model SP-220) at 285 nm (Meinerz et al., 2008). Sterilized distilled water together with Tween 80 were used as a negative control and the commercial resistance activator Acorda® (JUMA AGRO) was taken as positive control (Stangarlin et al., 2010).
Phytoalexin induction in sorghum mesocotyls
Sorghum (S. bicolor) seeds, Buster (Atlantic Seeds®), were placed in 1% sodium hypochlorite for 15 min and then washed in sterile distilled water. Then, the seeds were wrapped in moistened germination paper sheets and the leaves placed in upright bins, incubated in the dark at 28 ± 1°C within 4 days for germination to occur (Stangarlin et al., 2010). After this period, the seedlings formed were first exposed to light for 4 h so that the mesocotyl elongation would stop. In the sequence, the seedlings were sprayed with 2 mL of the samples to be tested (the same as the previous item). Sterilized distilled water + Tween 80 were used as the negative control, while the commercial resistance activator Biozyme® (Arysta Life Science) was the positive control. The seedlings were kept under fluorescent light at 25 ± 1°C for 60 h. Subsequently, the mesocotyls were removed, excised, weighed and placed in microcentrifuge tubes containing 1.4 mL of acidified 80% methanol (0.1% HCL; v/v). These were kept cooled to 4 ± 1°C per 96 h for the extraction of the pigments (Bonaldo, 2005). The absorbance was read at 480 nm (BioSpectro model SP-220) (Nicholson et al., 1988; Stangarlin et al., 2010).
Enzymatic activity
Determination of the enzymatic activity was performed both in the fresh leaves of L. sidoides and in the supernatant of the fermented medium of the six endophytic fungi associated with this plant which showed better results in the antifungal effect of Curvularia lunata (Wakker) (data not shown). Samples of L. sidoides were prepared by weighing 200 mg of the fresh leaves macerated in liquid nitrogen with 20% polyvinylpolyrolrolidone (PVPP). Upon formation of the powder, 1.5 mL of extraction buffer (100 mM potassium phosphate buffer, pH 7.0 added to 1 mM EDTA and 1 mM ascorbate to the volume of buffer used) was added. It was macerated for an additional 3 min and centrifuged at 14,000 g for 25 min at 4 ± 1°C. The supernatant was collected (protein extract) and stored at -20°C for further analysis. On the other hand, samples of the fungal extracts, that is, the liquid fermented by the fungi, were separated from the mycelia and conditioned directly in tubes for microcentrifuge at -20°C until the analyses were performed.
Superoxide dismutase (SOD; EC 1.15.1.1)
SOD activity was determined by inhibition of blue formazan production by nitroblue tetrazolium (NBT) photoreduction. The SOD was measured by adding 0.1 mL of protein extract in test tubes: 0.1 mL of 50 mM potassium phosphate buffer, pH 7.8; 0.02 mL of 0.1 mM EDTA; 0.4 mL of 70 mM L-methionine and 0.2 ml of 1 mM NBT. The reaction was started by adding 2 mM riboflavin and rapidly transferring the tubes, without light protection, to a 30 W lamp-lit chamber (30 μmol of photons m-2s-1) for 5 min. The absorbance was measured at 540 nm (BioSpectro model SP-220) (Giannopolotis and Ries, 1977). A unit of SOD activity was defined as the amount of enzyme required to inhibit 50% of NBT reduction and activity was expressed in unit per gram per extract per minute (U g-1 E-1 min-1), according to Beauchamp and Fridovich (1971).
Peroxidase phenols (POX; EC 1.11.1.7)
POX activity, according to the method proposed by Amako et al. (1994) was measured by adding 0.05 mL of the protein extract to a test tube; 2.9 mL of solution with 0.5 mL of 0.2 M guaiacol and 0.5 mL of 0.38 M hydrogen peroxide and 2 mL of 0.02 M sodium acetate buffer; pH 5.0. The readings were performed at 470 nm (BioSpectro model SP-220), intercalating 15 s for 3 min (12 readings) for each reaction. To reset the device, a blank was made by adding distilled water to the sample. The POX activity was expressed in micromole of hydrogen peroxide per gram per extract per minute (μmol H2O2 g-1 E-1 min-1).
Catalase (CAT; EC 1.11.1.6)
To determine CAT activity, 0.05 mL of protein extract was added; 2.95 mL of 50 mM potassium phosphate buffer, pH 7.8 with 20 mM hydrogen peroxide was added. The readings were recorded according to the decay of the absorbances. The activity was measured at 240 nm (BioSpectro model SP-220) for 300 s with readings performed every 30 s and calculated based on the molar extinction coefficient of 35 M-1 cm-1 (240 nm) and expressed as micromol hydrogen peroxide per gram per extract per minute (μmol H2O2 g-1 E-1 min-1) (Havir and Mchale, 1987).
Ascorbate peroxidase (APX; EC 1.11.1.11)
APX activity enzyme was measured by mixing 0.1 mL of the protein extract, 2.7 mL of 0.5 mM ascorbate (ASA) buffer and 0.2 mL of 30 mM hydrogen peroxide. The absorbance was measured at 290 nm (BioSpectro model SP-220) at 25°C by the degradation of hydrogen peroxide. APX activity was expressed as micromol of ascorbate per gram per extract per minute (μmol ASA g-1 E-1 min-1) (Asada and Takahashi, 1987).
Chitinase (QUIT; EC 3.2.1.14)
To determine QUIT activity, the release of soluble fragments of "CM-QUITin-RBV®" (Sigma-Aldrich) was observed by means of carboxymethylated QUITin labeled with bright violet remazol. For the reaction, 0.2 mL of the protein extract, 0.6 mL of 0.1 M sodium acetate buffer pH 5.0 and 0.2 mL of "CM-QUITin-RBV®" was added to 2.0 mg mL-1. Subsequently, it was incubated at 40°C for 20 min. The reaction was quenched with the addition of 0.2 mL of 1 M HCl, cooled on ice and centrifuged at 10,000 g for 5 min. The absorbance was measured at 550 nm (BioSpectro model SP-220). The activity of the QUIT enzyme was expressed in units of absorbance min-1 (U min-1).
Statistical analysis
The data were analyzed by ANOVA and, when significant, the averages were compared by the Tukey test for the qualitative factor and regression analysis for the quantitative factor, 5% error probability, with the aid of statistical analysis software ASSISTAT and SIGMAPLOT 12.0. The graph in the heatmap was performed on the software Gitools 2.3.1.
The assays with L. sidoides essential oil had a dose-dependent effect, with adjustment of the 1st-degree equation and R2 of 0.8006 for the production of phytoalexin glyceollin in soybean cotyledons (Figure 1A). All the concentrations tested (0.625, 1.25, 2.5, 5.0 and 7.5 mg mL-1) provided a synthesis of the phytoalexin with the highest induction value at the concentration of 7.5 mg mL-1. In addition, this concentration induced 66.48% more phytoalexin than the Acorda® positive control (Figure 1A). Phytoalexin 3-deoxythyanocyanidine induction was observed in sorghum mesocotyls (Figure 1B) production at all tested concentrations (0.625, 1.25, 2.5, 5.0 and 7.5 mg mL-1). In the regression analysis, it was possible to obtain the R2 value of 0.89 with a 3rd-degree equation having no dose-dependent effect (Figure 1B). The maximum value obtained was obtained in the concentration of 2.5 mg mL-1, with result of 28.25% higher than the Biozyme® positive control. Among endophytic fungi extracts associated with L. sidoides tested in this work, the one that presented greater result was the Fusarium sp. 1. This fungus induced 25.29% more phytoalexins than the positive control and 29.79% more than the Verticillium sp. fungus, which showed the lowest value (Figure 2A). The endophytic fungus, Verticillium sp. was the one that showed the best result regarding the production of 3-deoxythianocyanidine, and it induced 93.83% more phytoalexins than the fungus Fusarium sp. 2, which showed lower result, and 66.64% more than the negative control (Figure 2B).
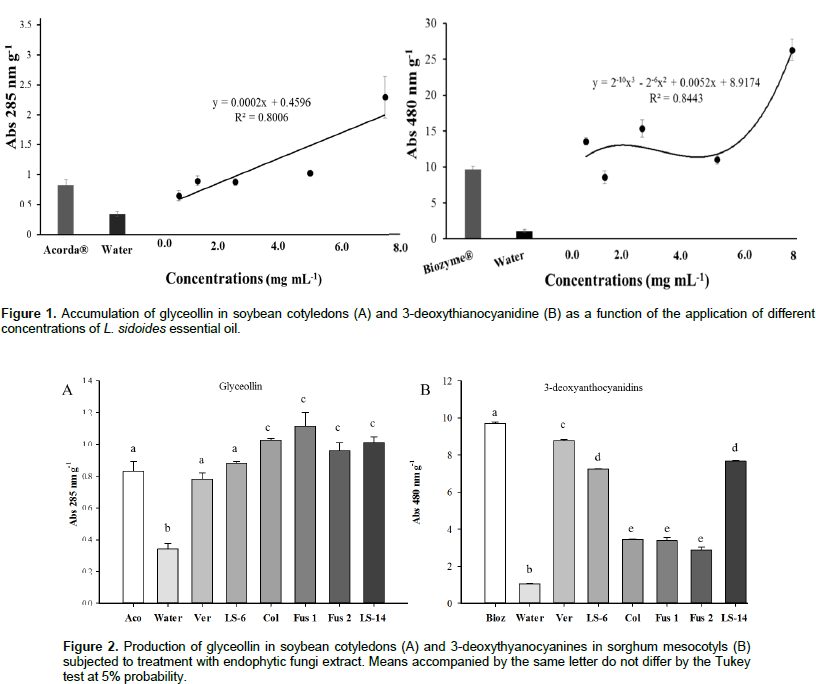
The results in Figure 3A show that there was activity of the SOD enzyme, where the highest value, 130 U g-1 E-1 min-1, was presented by the fungus LS-14. This value is higher than that of the L. sidoides plant, 64 U g-1 E-1 min-1. Regarding the activity of the QUIT enzyme, the highest value of 0.214 U min-1 was obtained for the Fusarium 1 fungus. It is also noted that, both the L. sidoides plant and the fungus Verticillium sp. did not synthesize the enzyme chitinase since no activity was detected (Figure 3B). For APX activity, the highest value was 3821 μmol ASA g-1 E-1 min-1 for L. sidoides (Figure 4A). For the activity of CAT, the featured was also for L. sidoides with 2429 μmol H2O2 g-1 E-1 min-1 of catalytic activity determination, however, no significant value of activity was observed for the fungus Colletotrichum sp. (Figure 4B). In relation to the POX activity, it can be observed that there was a contrast since the lowest value was for L. sidoides (2 μmol H2O2 g-1 E-1 min-1) and higher value for the fungus Fusarium sp. 2 was 87 μmol H2O2 g-1 E-1 min-1 (Figure 4C). Figure 5 shows the data of the enzymatic activity in heatmap graph format. It is a way of condensing information, where the columns correspond to the enzymes and the lines correspond to the extracts of both the plant and the six endophytic fungi studied. The colored tiles indicate the intensity of the enzymatic activity. It is shown in Figure 5 that the highest enzymatic activity was obtained with respect to the APX enzyme for L. sidoides extract and the smallest enzymatic activities are represented by QUIT.
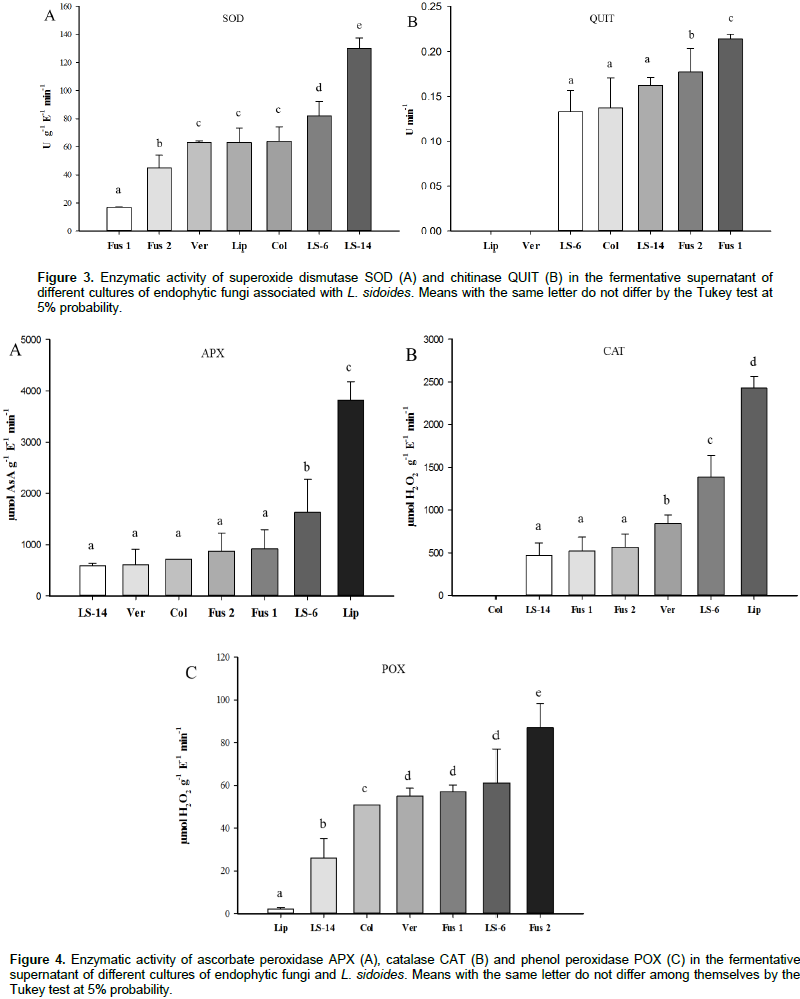
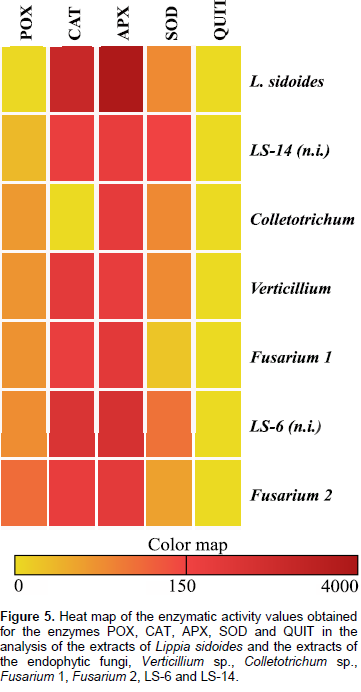
Among the defense activators of the tested plants, Acorda® induced the highest production of glyceollin in soy cotyledons, and it was used as a positive control (data not shown). For the sorghum tests, it was found that the Biozyme® activator generated better results for the induction of flavonoid 3-deoxythyanocyanidine (data not shown). Due to this bioactive and beneficial capacity for plants and other organisms, phytoalexin synthesis tests induced by endophytic fungal extracts were similarly performed. Previously, these extracts were screened against the phytopathogenic fungus C. lunata. The six extracts that inhibited in vitro mycelial growth of phytopathogen (Ferreira et al., 2017) were separated for the phytoalexin production tests. Therefore, all the endophytic fungi extracts associated with L. sidoides tested in this work demonstrated similar or greater induction of glyceollin than the positive control. Bonaldo et al. (2007) observed that the essential oil of Eucalyptus citriodora at the tested concentrations of 10-2, 10-4, 10-6, 10-8 and 10-10 did not promote the synthesis of phytoalexins in sorghum in contrast to the present work. However, the crude extract of the same plant induced accumulation of 3-deoxythianocyanidine. They believe that the negative result is because the solutions are quite diluted.
Other studies with leaf extracts of Ocimum gratissimum L. (alfavaca) induced the production of 3-deoxythianocyanidine when the 40% w/v concentration was used. Also, the same extract induced 6-fold more glyceollin synthesis than the control (distilled water) at 25% w/v concentration. In this research, the production of the two phytoalexins increased initially and then, with increase of the extract concentration, there was a decrease in the production. The authors indicated that phytoalexins were inactivated, possibly by irreversible binding to cell debris, which cannot be removed or detected (Colpas et al., 2009). This may also have occurred with the production of glyceollin from the concentrations of 5.0 and 7.5 mg mL-1 of L. sidoides essential oil in the present study. Glyceollin, phytoalexin derived from soybeans, exists as three mixed isomers. It is released at high concentrations during plant growth in response to a number of stress factors, such as injury, freezing, exposure to ultraviolet light, chemicals and exposure to microorganisms (Feng et al., 2007). Several studies have shown that its biological activities included antitumor, antiestrogenic, antibacterial and antifungal effects (Liu et al., 2014). Therefore, the analysis of its effects and its production are necessary to obtain an alternative type of control with the use of essential oils.
No similar results were found in relation to endophytic fungi extracts. However, Arruda et al. (2012) used an aqueous extract of mushrooms such as Agaricus blazei at 10% concentration and observed the induction of glyceollins. The accumulation from the concentration of 1 and 2% for extracts of Lentinula edodes was also evidenced; the induction was observed for the extracts of Pycnoporus sanguineus. Piccinin et al. (2000) used filtration of L. edodes basidiocarp autoclaved, filtered mycelial growth, filtered macerated mycelium and observed the induction of 3-deoxyanthiocyanins in mesocotyls of sorghum and glyceollins in soybean cotyledons. A small production of phytoalexins was observed in the negative control in which distilled water was used (Figures 1A, B, 2A and B). This small synthesis may be due to the mechanical lesions in the cotyledons to perform the tests. As phytoalexins appear after infection of the pathogen, it may be suggested that endophytic fungi synthesized molecules, releasing them from their hyphae into the fermentative medium (Hammerschmidt, 1999), which could be washed away with the solvent, ethyl acetate. These substances, when in higher concentrations, make the perception of signals derived from the elicitor more efficient, causing alterations in the cellular metabolism, such as activation of G proteins, increase in the ion flow through the plasma membrane, activity of kinases and phosphatases, activation of metabolic routes, among them, the synthesis of phytoalexins (Mazaro et al., 2008).
Typically, 2 to 5% of O2 is reduced univalent, a process in which a molecule receives only one electron, which will occupy one of the outer orbitals while the other remains unpaired. The consequence of this is the production of highly reactive intermediates, called reactive oxygen species (ROS), free radicals. Thus, the first reactive toxic species of oxygen, superoxide (O2•-), is formed. This radical can be disrupted in hydrogen peroxide (H2O2) or even through catalytic action by the action of the enzyme SOD (Halliwell and Gutteridge, 1989). Thus, by identifying the presence of enzymatic activity of SOD in the fermentative products of endophytic fungi associated with L. sidoides, it is possible to establish a real, mutual and synergistic relationship of these organisms. The plant favors the growth of these fungi in its interior as the comfortable habitat for the life cycle of these microorganisms, and also, the fungi also protect it by helping in the elimination of these ROS. Therefore, through the enzymatic activities performed in the present study, this was observed. All fungal samples contained enzyme activity. Nunes et al. (2006), observed an increase in the mean values of the enzymatic activity of SOD when the combination of the phytohormones 6-benzylaminopurine acid (BAP) and naphthaleneacetic acid (ANA) was used, which led to the accumulation of ROS due to oxidative stress. There are also studies on endophytic fungi of wine grape, which were reinoculated to show the antioxidative activities. It includes the enzymatic activity of SOD for a possible application of endophytic fungi as metabolic regulators in winemaking (Yang et al., 2016).
There are no reports on chitinase activity in L. sidoides leaves in the literature. Souza et al. (2001) found endochitinases produced by the fungus, Colletotrichum gloeosporioides. The Fusarium fungus has no history of chitinase production. Brzezinska and Jankiewicz (2012) reported on the use of chitinases against phytopathogenic Fusarium culmorum and Fusarium solani. It is important that an organism synthesizes chitinases since it has been demonstrated that the overexpression of chitinase genes in plants increases resistance to pathogens. This enzyme acts on the hydrolysis of QUIT in polymers, whose substances are part of the fungal cell walls (Van Loon et al., 2006). Therefore, it would be a great antimicrobial. The activity of APX, CAT and POX was also performed for both the L. sidoides plant and the associated endophytic fungi. These tests were also done in order to detect the presence of these enzymes and to infer that endophytic fungi really help, protect and live in synergy with the host plant. The enzymes APX, CAT, POX and SOD are among the enzymes considered antioxidative. They can be found in virtually all cell sites and plants, and participate in the hydrogen peroxide detoxification system (Asada, 1992). Determination of the activity of these enzymes plays an important role in elucidating the main functions of the endophytic fungi inside their host plants. They contribute to the combined actions of the plant and fungus. In addition, ROS lead to the oxidation of biomolecules with consequent loss of their biological functions and/or homeostatic imbalance, causing damage to plant cells and tissues such as fungi (Halliwell and Whiteman, 2004). All fungal samples contained enzyme activity.
In the work of Pimenta et al. (2013), these enzymes were detected in the plant, Lippia filifolia, where they verified the effect of the inhibition of ethylene synthesis on oxidative stress. The expression of the genes encoding these enzymes is dependent on several types of abiotic and biotic stresses emphasizing the importance and complexity of understanding the mechanisms involved and their complexity. Both enzymes and antioxidative metabolites may be able to prevent the accumulation of ROS and oxidative stress (Saher et al., 2004), serving as the signal to prevent abnormalities (Nunes et al., 2015). Further study will be carried out to verify the antimicrobial activity of the phytoalexins extracted from the extracts. There is also a need to establish other methodologies for extracting phytoalexins in corn crop, for example. By means of real-time PCR analysis, synthesis of the POX, CAT, APX, SOD and QUIT enzymes can be quantitatively verified by the endophytic fungi and by the plant, as well as to verify the induction of the synthesis of these enzymes in cultures of agricultural interest before and after the application of these extracts. Research related to the induction of defense and/or resistance substances that are natural are important and necessary for use in agriculture. They can be cheaper, more efficient, biodegradable and do not cause harm to the environment.
The essential oil of L. sidoides and all extracts of the endophytic fungi associated with it can be considered elicitors of phytoalexins by the presence of molecules that indicate triggering of the biosynthesis of the same ones. In addition, the presence of enzymes related to antioxidative activity such as SOD, APX, POX and CAT and related to the hydrolysis of QUIT in polymers, such as in both leaves of the plant and the fermented liquid of the endophytic fungi, were verified and proved. In this way, it has important and potential commercial products for activating plant defense and promoting its resistance in cultivars of agricultural interest, but mainly organic farming.
The authors have not declared any conflict of interests.
REFERENCES
Alviano CS, Farbiarz SR, Travassos LR, Angluster J, Souza W (1992). Effect of environmental factors on Fonsecaea pedrosoi morphogenesis with em phasis on sclerotic cells induced by propranolol. Mycopathologia. 119(1):17-23. Amako K, Chen GX, Asada K (1994). Separate assays specific for ascorbate peroxidase and guaiacol peroxidase and for the chloroplastic and cytosolic isozymes of ascorbate peroxidase in plants. Plant Cell Physiol. 35(3):497-504.
|
|
Arruda RS, Mesquini RM, Schwan-Estrada KRF, Nascimento JF (2012). Efeito de extratos de cogumelos na indução de fitoalexinas e no controle de oídio da soja em casa de vegetação. Biosci. J. 28(2):164-172.
|
|
|
Asada K, Takahashi M (1987). Production and scavenging of active oxygen in photosynthesis, In: Kyle, D.J., Osmond, B., Arntzen, C.J., (Eds.), Photoinhibition. Amsterdam, Elsevier, pp. 227-287.
|
|
|
Asada K (1992). Ascorbate peroxidase - A hydrogen peroxide-scavenging enzyme in plants. Physiol. Plant. 85(2):235-241.
Crossref
|
|
|
Beauchamp C, Fridovich I (1971). Superoxide dismutase: improved assay applicable to acrylamide gels. Anal. Biochem. 44(1):2762-2787.
Crossref
|
|
|
Bonaldo S (2005). Efeito de Saccharomyces cerevisiae na síntese de fitoalexinas em sorgo, na germinação e formação de apressórios por fungos fitopatogênicos e na proteção de pepino a Colletotrichum lagenarium e sorgo a Colletotrichum sublineolum. 166 f. Tese de Doutorado (Agronomia) - Universidade de São Paulo, Piracicaba.
Crossref
|
|
|
Bonaldo SM, Schwan-Estrada KR, Stangarlin JR, Cruz ME, Fiori-tutida AC (2007). Contribuição ao estudo das atividades antifúngica e elicitora de fitoalexinas em sorgo e soja por eucalipto (Eucalyptus citriodora). Summa Phytopathologica. 33(4):383-387.
Crossref
|
|
|
Brzezinska MS, Jankiewicz U (2012). Production of antifungal chitinase by Aspergillus niger LOCK 62 and its potential role in the biological control. Curr. Microbiol. 65(6):666-672.
Crossref
|
|
|
Colpas FT, Schwan-Estrada KRF, Stangarlin JR, Ferrarese ML, Scapim CA, Bonaldo SM (2009). Induction of plant defense responses by Ocimum gratissimum L. (Lamiaceae) leaf extracts. Summa Phytopathol. 35(3):191-195.
Crossref
|
|
|
Da Silva TF, Vollú RE, Jurelevicius D, Alviano DS, Alviano CS, Blank AF, Seldin L (2013). Does the essential oil of Lippia sidoides Cham. (pepper-rosmarin) affect its endophytic microbial community? BMC Microbiol. 13(1):29.
Crossref
|
|
|
Dhankhar S, Kumar S, Dhankhar S, Yadav JP (2012). Antioxidant activity of fungal endophites isolated from Salvadora oleoides decne. Int. J. Pharm. Pharm. Sci. 4(2):380-385.
|
|
|
Devi PS, Saravanakumar M, Mohandas S (2011). Identification of 3- deoxyanthocyanins from red sorghum (Sorghum bicolor) bran and its biological properties. Afr. J. Pure Appl. Chem. 5(7):181-193.
|
|
|
Feng S, Saw CL, Lee YK, Huang D (2007). Fungal-stressed germination of black soybeans leads to generation of oxooctadecadienoic acids in addition to glyceollins. J. Agric. Food Chem. 55(21):8589-8595.
Crossref
|
|
|
Ferreira TPS, Santos GR, Soares IM, Ascêncio SD, Alvim TC, Siqueira CA, Aguiar RWSA (2017). Secondary metabolites from endophytic fungus from Lippia sidoides Cham. J. Med. Plant Res. 11(16):296-306.
Crossref
|
|
|
Giannopolotis CN, Ries SK (1977). Superoxide dismutases: I. occurrence in higher plants. Plant Physiol. 59:309-314.
Crossref
|
|
|
Guimarães LGL, Cardoso MG, Zacaroni LM, Lima RK (2008). Influência da luz e da temperatura sobre a oxidação do óleo essencial de capim-limão Cymbopogon citratus (D.C.) Stapf). Quim. Nova. 31(6):1476-1480.
Crossref
|
|
|
González-Lamothe R, Mitchell G, Gattuso M, Diarra MS, Malouin F, Bouarab K (2009). Plant antimicrobial agents and their effects on plant and human pathogens. Int. J. Mol. Sci. 10:3400-3419.
Crossref
|
|
|
Halliwell B, Gutteridge JMC (1989). Free radicals in Biology and Medicine. Oxford: Clarendon Press 543 p.
|
|
|
Halliwell B, Whiteman M (2004). Measuring reactive species and oxidative damage in vivo and in cell culture: how should you do it and what do the results mean? Br. J. Pharmacol. 42(2):231-55.
Crossref
|
|
|
Hammerschmidt R (1999). Phytoalexins: What Have We Learned After 60 Years? Annu. Rev. Phytopathol. 37:285-306.
Crossref
|
|
|
Havir EA, Machale NA (1987). Biochemical and developmental characterization of multiple forms of catalase in tobacco-leaves. Plant Physiol. 84(2):450-455.
Crossref
|
|
|
Jackson M, Karwoswski JP, Humphrey PE, Kohl WL, Barlow GJ, Tanaka SK (1993). Calbistrins, novel antifungal agents produced by Penicillium restrictum. J. Antibiot. 46:34-38.
Crossref
|
|
|
Liu Y, Wu Z, Feng S, Yang X, Huang D (2014). Hormesis of glyceollin I, an induced phytoalexin from soybean, on budding yeast chronological lifespan extension. Molecules 19:568-580.
Crossref
|
|
|
Lo CL, Weiergang I, Bonham C, Hipskind J, Wood K, Nicholson RL (1996). Phytoalexin accumulation in sorghum: identification of methyl ether of lutheolinidin. Physiol. Mol. Plant Pathol. 49:21-31.
Crossref
|
|
|
Matiello J, Bonaldo SM (2013). Atividade elicitora de fitoalexinas em soja e sorgo por extratos e tinturas de espécies medicinais. Rev. Bras. Plantas Med. 15(4):541-550.
Crossref
|
|
|
Mazaro SM, Fogolari H, Wagner Júnior A, Citadin I, Santos I (20130. Potencial de extratos à base de Calendula officinalis L. na indução da síntese de fitoalexinas e no efeito fungistático sobre Botrytis cinerea, in vitro. Rev. Bras. Plantas Med. 15(2):208-216.
|
|
|
Meinerz CC, Formighieri AP, Schwan-Estrada KRF, Dieterich C, Franzener G, Stangarlin JR (2008). Atividade elicitora de fitoalexinas em sorgo e soja por derivados de avenca (Adiantum capillus-veneris L.). Rev. Bras. Plantas Med. 10(2):26-31.
|
|
|
Miller G, Suzuki H, Ciftci-Yilmaz S, Mittler R (2010). Reactive oxygen species homeostasis and signaling during drought and salinity stresses. Plant Cell Environ. 33:453-467.
Crossref
|
|
|
Nicholson RL, Jamil FF, Snyder BA, Lue WL, Hipskind J (1988). Phytoalexin synthesis in the juvenile sorghum leaf. Physiol. Mol. Plant Pathol. 33:271-278.
Crossref
|
|
|
Nunes RCA, Viana RS, Neto NBM (2015). Atividade enzimática da superóxido dismutase em resposta aos fitorreguladores em Gerbera jamensonii. Comunicata Scientiae. 6(1):83-89.
|
|
|
Oliveira JSB, Schwan-Estrada KRF, Bonato CM, Carneiro SMTPG (2016). Homeopatias de óleos essenciais sobre a germinação de esporos e indução de fitoalexinas. Rev. Ciênc. Agron. 48(1):208-215.
|
|
|
Peiter-Beninca C, Franzener G, Assi L, Iurkiv L, Eckstein B, Costa VC, Nogueira MA, Stangarlin JR, Schwan-Estrada KRF (2008). Indução de fitoalexinas e atividade de peroxidases em sorgo e soja tratados com extratos de basidiocarpos de Pycnoporus sanguineus. Arq. Inst. Biol. 75(3):285-292.
|
|
|
Piccinin E (2000). Potencial de preparações do cogumelo comestível "shiitake" (Lentinula edodes) no controle de fitopatógenos fúngicos, bacterianos e virais em sorgo, maracujá e fumo. 2000. 160f. Tese (Doutorado), Universidade de São Paulo, Piracicaba.
|
|
|
Pimenta MR, Ribeiro C, Soares CQG, Mendes GC, Braga VF, Reis LB, Otoni WC, Resende CF, Viccini LF, Peixoto OHP (2013). Ethilene syntesis inhibition effects on oxidative stress and in vitro conservation of Lippia filifolia (Verbenaceae). Braz. J. Biol. 73(3):617-621.
Crossref
|
|
|
Ribera AE, Zu-iga G (2012). Induced plant secondary metabolites for phytopatogenic fungi control: a review. J. Soil Sci. Plant Nutr. 12(4):893-911.
Crossref
|
|
|
Saher S, Piqueras A, Hellin E, Olmos E (2004). Hyperhydricity in micropropagated carnation shoots: the role of oxidative stress. Physiologia Plant. 120:152-161.
Crossref
|
|
|
Smith CJ (1996). Accumulation of phytoalexins: defense mechanisms and stimulus response system. New Phytol. 132:1-45.
Crossref
|
|
|
Schwan-Estrada, KRF, Stangarlin JR, Cruz MES (2000). Uso de extratos vegetais no controle de fungos fitopatogênicos. Floresta. 30(1-2):129-137.
Crossref
|
|
|
Souza RF, Gomes RG, Alviano GS, Ferreira MS, Coelho RRR, Soares RMA (2001). Purificação e caracterização de uma endoquitinase produzida pelo Colletotrichum gloeosporioides. Universidade do Rio de Janeiro, Departamento de Microbiologia geral. XXI Congresso Brasileiro de Microbiologia, P 40.
|
|
|
Stangarlin JR, Schulz DG, Franzener G, Assi L, Schwan-Estrada KRF, Kuhn OJ (2010). Indução de fitoalexinas em soja e sorgo por preparações de Saccharomyces boulardii. Arq. Inst. Biol. 77:91-98.
|
|
|
Van Loon LC, Rep M, Pieterse CMJ (2006). Significance of inducible defense-related proteins in infected plants. Annual Review of Phytopathology. 44:135-162.
Crossref
|
|
|
Venturoso LR, Bacchi LMA, Gavassoni WL, Conus LA, Pontim BCA, Bergamin AC (2011). Atividade antifúngica de extratos vegetais sobre o desenvolvimento de fitopatógenos. Summa Phytopathol. 37(1):18-23.
Crossref
|
|
|
War AR, Paulraj MG, Ahmad T, Buhroo AA, Hussain B, Ignacimuthu S, Sharma HC (2012). Mechanisms of plant defense against insect herbivores. Plant Signal. Behav. 7:1306-1320.
Crossref
|
|
|
Yang MZ, Ma MD, Yuan MQ, Huang ZY, Yang WX, Zhang HB, Huang LH, Ren AY, Shan H (2016). Fungal Endophytes as a Metabolic Fine-Tuning Regulator for Wine Grape. PLoS ONE. 11(9):1-17.
Crossref
|
|
|
Zarei M, Aminzadeh S, Zolgharnein H, Safahieh A, Daliri M, Noghabi KA, Ghoroghi A, Motallebi A (2011). Characterization of a chitinase with antifungal activity from a native Serratia marcescens B4A. Braz. J. Microbiol. 42(3):1017-1029.
Crossref
|
|