ABSTRACT
Sweet potato (Ipomoea batatas L. Lam) has become one of the staple crops in Africa in the last 20 years. In Ethiopia, sweet potato is the second most widely grown root crop and is the first regarding the production per hectare. Thus, there is a great demand of planting material throughout the country. Currently, planting material is usually obtained from own previous season harvest, local markets or from the neighboring fields since no certified clean planting material production scheme has been established in Ethiopia yet. Unfortunately, this practice has contributed to the spread of viral diseases throughout the country. Elimination of viruses from infected plants is a tedious job, which requires efficient methods to eliminate the virus and also to verify that the plants are indeed virus-free. In the case of sweet potato, it was observed that heat treatment, combined with meristem tip culture is an efficient method for virus elimination. Previous findings indicate that reverse transcription (RT) PCR is more efficient than ELISA to verify the efficiency of virus elimination. In this study, the use of next generation sequencing (NGS) was explored as a verification method and compared with RT-PCR. The results show that NGS seems to be more efficient than RT-PCR, although also prone to inconclusive results.
Key words: Viruses, next generation sequencing (NGS), sweet potato, reverse transcription (RT) PCR, badnavirus.
Sweet potato [(Ipomoea batatas L. Lam)] is the seventh most economically important crops in the world with total annual production of 12.3 million tones (FAO, 2017). China leads the production followed by Nigeria, Tanzania, and Uganda and Ethiopia. Sweet potato is grown and consumed mainly by small-scale farmers in
Ethiopia. Nevertheless, sweet potato demand, as a food source and as planting material has increased substantially over the last 10 years. In Ethiopia, the Southern Nations Nationalities Peoples Region (SNNPR) is the dominant sweet potato production area. Unfortunately, this area is also where sweet potato viral diseases cause severe reduction of crop yields (Tesfaye et al., 2011, 2013).
In order to increase the production of sweet potato, it is necessary to devise a strategy to produce clean planting material that can supply the market. An initial step for the establishment of a certified clean planting material production scheme is to identify the most widely used varieties in the country, identify the virus that are infecting these varieties, and device methods by which these varieties can be “cleaned” from the infecting viruses. During the last five years, all of these goals were accomplished. Nevertheless, true development also requires the incorporation of novel techniques, which would contribute to the scientific development of the country.
During the last 10 years, next generation sequencing (NGS) has proven to be a reliable robust method for screening for viruses in plants (Kreuze et al., 2009; Kashif et al., 2012; Jones et al., 2017). This method allows the detection of viruses infecting a given host without prior knowledge on the existence of the virus, provides sequences data that can be used to classify the viruses into taxonomical categories and more sensitive than RT-PCR in detecting viruses in symptomless plants (Maliogka et al., 2018). NGS has been used to screen for viruses in sweet potato, revealing the presence of many viral species in this crop (Kashif et al., 2012; Mbanzibwa et al., 2014; Kreuze et al., 2017; Nhlapo et al., 2018). Nevertheless, NGS has not been previously used for virus screening in Ethiopian varieties of sweet potato.
Previous findings indicate that reverse transcription (RT) PCR is more efficient than ELISA to verify the efficiency of virus elimination in sweet potato varieties. Thus, in this paper, the efficiency of using reverse transcription PCR (RT-PCR) was compared with NGS as a method to verify efficient elimination of viruses utilizing heat treatment combined with meristem tip culture in the five most widely used sweet potato varieties in Ethiopia. Results showed that NGS seems to be more reliable than RT-PCR, although it can also lead to unexpected results. In addition, by using NGS as a detection method, the occurrence of two sweet potato viruses was also identified previously not known to be present in Ethiopia.
Plant materials
Five sweet potato varieties (‘Hawassa-83’, ‘Berkume’, ‘Tola’, ‘Kulfo’ and ‘Guntute B’) were obtained from research and academic institutions in Ethiopia. Four varieties (‘Hawassa-83’, ‘Berkume’, ‘Tola’, and ‘Kulfo’) were symptomless, whereas one (‘Guntute B’) showed severe virus symptoms. Shoot tips of each variety were planted in two sets. One set was subjected to heat treatment followed by meristem culture for virus elimination and the other set was used as the control initial plants. Following heat treatment, the in vitro plants were regenerated from meristem cultures.
Heat therapy
Heat-therapy treatment, followed by meristem tip culture was applied according to the method described for sweet potato (Dennien et al., 2013), with some modifications described as follows. Five vine cuttings from each variety were potted and grown in a growth chamber at a daily temperature cycle of 25/18°C under a 12/12 h photoperiod with a light intensity of 150 μmol m-2s-1 provided by cool white fluorescent tubes (Osram L 58W/840 Lumilux). After four weeks, the potted plants were divided into two sets each consisting of three potted plants of each variety. One set continued to be grown under same conditions and used as a meristem donor for meristem culture alone (non-heat treated control). The other set was transferred to a different growth room where it was subjected to heat therapy for 8 weeks at daily temperatures cycle of 39 ± 1°C / 25 ± 1°C day/night, 12/12 h of photoperiod and light intensity of 150 μmolm-2s-1 provided by cool white fluorescent tubes (Osram L 58W/840 Lumilux).
Meristem culture and plant regeneration
Meristems were excised from both experimental conditions (non-heat treated and the heat-treated donor plants) and cultured in-vitro on nutrient media optimized for the sweet potatoes (Dereje and Hvoslef-Eide, 2020). The cultures were placed in the dark for a week at 25 ± 1°C and then moved to a growth condition of more intensity of light (28 μmolm-2s-1) provided by cool white fluorescent tubes (Osram L 58W/840 Lumilux). After five weeks, surviving meristems were counted and sub-cultured into test tubes containing a fresh medium of the same composition, for further shoot initiations. After initiation, plantlets were elongated and multiplied on plant growth regulators-free 1/2 basal MS media for subsequent virus indexing using combinations of methods described in Dereje et al. (submitted). The virus elimination efficiency of meristem culture alone and combined heat therapy were compared based on the percentages of plantlets that were cured of infecting viruses by each method.
Virus testing
All plants were tested by RT-PCR and NGS before and after heat treatment-meristem tip culture. RT-PCR was used to test for SPVC, SPVG, SPV2, SPFMV, SPCSV and SPMMV as follows. Briefly, total RNA was extracted from 0.1 g fresh leaf samples. These samples were grounded to a fine powder in liquid nitrogen using mortar and pestle. RNA extraction was performed using Trizol reagents (Invitrogen, Life Technologies) following the manufacturer’s procedures. Total RNA was quantified and the quality verified using Nanodrop (Thermo Fisher Scientiï¬c, USA), Agilent 2100 Bio-analyzer (Agilent Technologies, USA) and with a 1% agarose gel electrophoresis stained with ethidium bromide.
First strand cDNA was synthesized using 2.5 μg total RNA template, random primer (Invitrogen) and Super Script™ II Reverse Transcriptase (Invitrogen) and all other reaction components and reaction conditions were according to the manufacturer’s recommendations (Invitrogen, California ©2010 Life Technologies).
PCR reactions were carried out using the first strand cDNA as a template, virus-specific primers to SPVC, SPVG, SPV2 (Li et al., 2012) and SPFMV, SPCSV, and SPMMV (Kathurima et al., 2011) and TIF DNA polymerase (Invitrogen) following the procedures of the manufacturers and the appropriate controls. Amplification was performed under the reaction conditions of 94°C for 2 min, 35 cycles of 94°C for 20 s, 52°C (for SPVC, SPVG, SPMMV and SPCSV) or 53°C (for SPFMV) for 20 s, and 72°C for 30 s. A final elongation was carried out at 72°C for 5 min.
For NGS, total RNA was extracted from 100 mg leaf tissue using Trizol (Invitrogen, CA, USA). Extracted RNA samples were purified using PureLink RNA Mini purification kits and DNase treated using the TURBO DNA-free kit (Invitrogen by thermofisher scientific) according to the manufacturer’s instructions. The RNA samples quantity and purity were evaluated using a Nanodrop (NanoDropTechnologies, Wilmington, DE, USA). Furthermore, the RNA integrity was confirmed using Agilent 2100 Bioanalyzer (Agilent Technologies, Santa Clara, CA, USA). Total RNA (3 µg) was sent to Fasteris genome sequencing services center (Geneva, Switzerland) where the sample were sequenced.
Data analysis
Raw RNA sequence data sets obtained from Fasteris were downloaded and analyzed using the Virus Detect software (Zheng et al., 2017). For the virus detect analysis, reads in the size range of 10 to 50 nucleotides were used.
Next generation sequencing
Small RNAs reads were generated using small RNA (sRNA) molecules of less than 30 nucleotides in size. sRNA deep sequenced data of the 5 varieties before and after virus elimination treatments were analyzed by denovo assembly, using the Virus Detect software package. Between 8.26 and 10.35 million sRNAs (1 to 50 bp) reads were generated per sample (Table 1). On average, 9.35 million reads were generated per sample and 79.4% of the sRNAs reads generated are between the size ranges of 18 to 26 bp (Table 1). The size distribution of the sRNAs read of the 10 samples libraries had slight differences. For all the libraries, reads with size of 21 and 24 bp accounted for the majority of sRNAs reads.
NGS reveals the existence of sweet potato viruses previously unknown to be present in Ethiopia
Table 2 shows viruses known to infect sweet potato detected in the samples before and after heat treatment-meristem tip culture. The following sweet potato viruses were detected: SPFMV, SPVC, SPCSV, SPVG, Sweet potato symptomless mastrevirus-1 (SPSMV-1) and three sweet potato badnaviruses (SPBV-A, SPBV-B, SPBV-C) collective called Sweet potato papakuy virus (SPPV). Among these SPFMV, SPVC, SPCSV and SPVG have previously been detected in Ethiopia. However, and to our knowledge this is the first report of SPSMV-1 and SPPV in Ethiopia.
SPPV (family Caulimoviridae; genus Badinavirus) is the most commonly detected virus in sweet potato (Mbanzibwa et al., 2014; Kreuze et al., 2017) and has been reported to occur in many accession belonging to the sweet collection at International Center for Potato (CIP) (Kreuze et al., 2017). SPPV was initially identified in sweet potato plants showing no visible symptoms (Kreuze et al., 2009). In this study, SPPV was detected in 5/5 of the initial plants samples and 3/5 of plants regenerated after heat-treated and meristem culture. Thus, heat therapy and meristem tip culture have 50% chance of eliminating the virus. Whether SPPV is endemic to Ethiopia or was introduced into the country through infected material used for breeding purposes, is unknown. Perhaps the wide use of CIP accessions in breeding programs has contributed to the spread of this virus in Ethiopia.
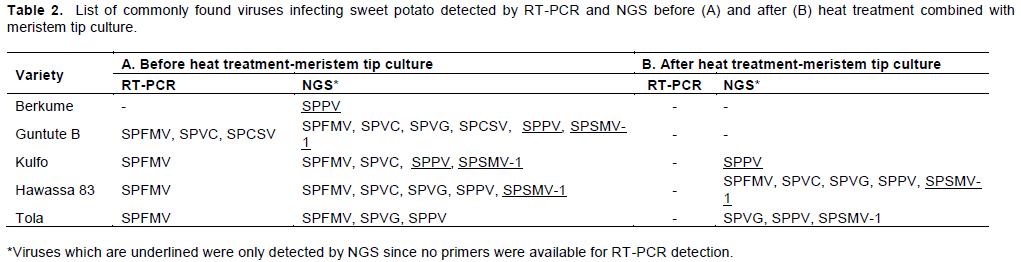
Viruses, which occur in in apparently healthy-looking plants have a higher chance of dissemination between farms when planting materials, are exchanged. Although Badinaviruses do not cause visible damage in sweet potato, they are serious pathogens of tropical horticultural crops: Banana, black pepper, cocoa, citrus, sugarcane, taro and yam (Bhat et al., 2016; Bömer et al., 2018). Thus, NGS can be a potential detection tool in rigorous certification schemes in nations where susceptible crops to these viruses exist.
SPSMV-1 (family Geminiviridae, genus Mastrevirus) has previously been reported to occur in sweet potato plants in Peru, Tanzania, Central America, China and Korea (Kreuze et al., 2009; Kwak et al., 2014; Mbanzibwa et al., 2014; Wang et al., 2015; Bhat et al., 2016; Kreuze et al., 2017; Bömer et al., 2018). The virus usually occurs as a symptomless infection and does not result in a severe disease. SPSMV-1 was detected in three varieties (‘Guntute B’, ‘Kulfo’, and ‘Hawassa-83’) before virus removal treatments. Based on the NGS results from the treated plants, SPSMV-1 was efficiently removed from ‘Guntute B’ and ‘Kulfo’, but not from ‘Hawassa-83’. However, surprisingly SPSMV-1 was also detected in ‘Tola’ (which was negative to SPSMV-1 before treatment).
Next generation sequencing (NGS) screening is more sensitive than RT-PCR
Throughout this study, two independent methods (NGS and RT-PCR) were employed to test viruses in the plant material. All plant material was tested by RT-PCR and NGS before and after heat treatment-meristem tip culture. Thus, by using these two independent methods, it is believe that our results are more reliable.
RT-PCR was initially used to test for SPVC, SPVG, SPV2, SPFMV, SPCSV, and SPMMV in all plants before undergoing virus elimination treatment. Of the six viruses tested only SPFMV, SPVC and SPCSV were detected. The results showed that 4 out of the 5 varieties were infected with SPFMV, and these results were verified by NGS. Furthermore, RT-PCR results indicated that the only one plant (‘Guntute B’) was infected with SPCSV, and these results were verified by NGS. However, RT-PCR results also indicated that only one plant (‘Guntute B’) was infected by SPVC whereas NGS indicated that this virus also infected two other varieties ‘Kulfo’ and ‘Hawassa-83’. Moreover, RT-PCR did not detect SPVG in any of the plants, whereas NGS revealed that three varieties (‘Guntute B’, ‘Hawassa 83’ and ‘Tola’) were infected with SPVG.
RT-PCR was also used to test for SPVC, SPVG, SPV2, SPFMV, SPCSV and SPMMV in all plants after undergoing virus elimination treatment. None of the viruses tested for were detected in any of the plants.
Thus, based only on RT-PCR results, the virus elimination treatment was successful in all varieties; efficiently eliminating the viruses (SPFMV, SPVC, SPCSV and SPVG) previously found infecting the untreated material. However, NGS results revealed that none of the viruses found infecting ‘Hawassa-83’ (SPFMV, SPVC and SPVG) was eliminated, whereas only SPFMV had been eliminated from ‘Tola’, which was still infected with SPVG. Surprisingly, NGS results also revealed that ‘Tola’ was also infected with SPSMV-1, even though this virus had not been detected in the plant before virus elimination treatment. More experiments are required to verify that the initial material was indeed free of SPSMV-1 or that the viral concentrations are below the detection threshold of NGS.
Abundances of homology based assembled vsiRNAs contigs differs between non-treated and treated plants and correlated with virus elimination treatments
Viral-derived siRNA (vsiRNAs) contigs are contigs which are assembled from siRNA molecules that belong to viral sequences. Thus, in theory, in the absence of viral infection, no vsiRNA contigs should be obtained. In this study, the number of viral-derived siRNA (vsiRNAs) contigs assembled from non-treated plants was higher in most of the plants (with the exception of one plant) than that of the treated plants (Figure 1). Heat treatment followed by meristem tip culture is an efficient method for eliminating viruses. Thus, a lower number of vsiRNAs derived contigs in the plants after undergoing heat treatment-meristem tip culture, might be due to the lower viral population present in the plant after the treatment. Nevertheless, and surprisingly, one variety (‘Hawassa-83’) showed a higher number vsiRNA derived contigs in the plant that had gone through heat treatment-meristem tip culture. The initial, non-treated “Hawassa-83” plant was infected with SMFMV, SPVC, SPPV, SPVG and SPSV-1 as determined by NGS. However, none of these viruses where eliminated from the plant by heat-treatment followed by meristem tip culture. Among the viruses found infecting ‘Hawassa-83’, SPPV belong to the genus Badnavirus.
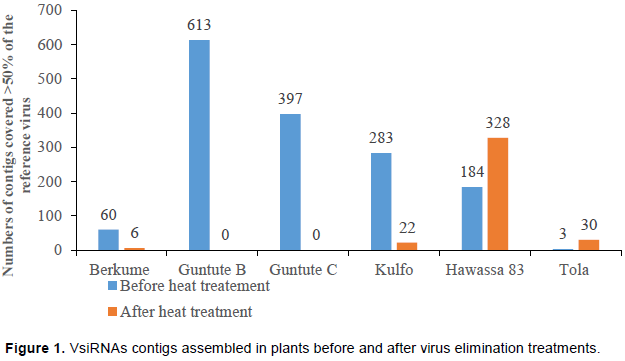
Within the genus Badnavirus, Banana streak virus (BSV) occurs in two stages (endogenous and episomal). BSV which is present as an integrated sequence in the host’s (banana) genome is named endogenous eBSV. On the other hand, when the virus is present as an episomal agent, capable of producing viral particle, it is named BSV. The functional episomal stage of BSV is triggered when the plant goes through some kind of stress, such as meristem tip culture (Gayral et al., 2010). Indeed, it is widely known that meristem tip culture in banana results in an increase of BSV in this host. Therefore, it is tempting to suggest that higher number of vsiRNA-derived contigs found in ‘Hawassa-83’ after the treatment corresponds to an increase in SPPV caused by the activation of the episomal stage of the virus due to the stress induce by procedure of meristem tip culture. Nevertheless, SPPV was also found in varieties ‘Kulfo’ and ‘Tola’ after heat-treatment followed by meristem tip culture. In both of these varieties the number of vsiRNAs derived contig was lower than that of the untreated plants. Thus, further experiments are required to determine if these results are indeed correct or an experimental mistake.
In this study the inclusion of NGS as means to screen viruses in plants before and after virus elimination treatment (heat treatment and meristem culture) helped verify the efficiency of this method. This study is the first to use deep sequencing to characterize siRNAs derived from viruses infecting sweet potato from Ethiopia. Using NGS the presences of previously reported viruses: SPCSV, SPFMV, SPVC and SPVG in sweet potato plants from Ethiopia were confirmed. Moreover, this study reports new, but less important sweet potato viruses: SPPV and SPSMV-1 for the first time. This study demonstrated using the more recent molecular methods (NGS) enables the detection of previously unidentified viruses, without the need of utilizing virus-specific primers or antibodies. Moreover, this study shows that NGS is more sensitive than RT-PCR, although it can also give unpredictable results. Thus, standardizing the method is required before it is used in large scale or in rigorous certification schemes. Although the cost for NGS are still very expensive for routine testing in developing countries (such as Ethiopia), it was envision that in the near future NGS will be cheap enough to become the standard testing utilized in certification schemes.
The authors have not declared any conflict of interests.
The assistance rendered to the authors by the technicians Astrid Helene Sivertsen (meristem cuttings to clean viruses, media preparation) and Gry Skjeseth (heat treatment, as well as caretaking of plants in the green house) are duly appreciated.
This work was supported by the NORAD funded project ‘‘Controlling disease in sweet potato and enset in South Sudan and Ethiopia to improve productivity and livelihoods under changing climatic conditions using modern technologies’’ under the NORHED program (agreement no ETH-13/0017, 2013).
REFERENCES
Bhat AI, Hohn T, Selvarajan R (2016). Badnaviruses: The current global scenario. Viruses 8(6):177.
Crossref
|
|
Bömer M, Rathnayake AI, Visendi P, Silva G, Seal SE (2018). Complete genome sequence of a new member of the genus Badnavirus, Dioscorea bacilliform RT virus 3, reveals the first evidence of recombination in yam badnaviruses. Archives of Virology 163(2):533-538.
Crossref
|
|
|
Dennien S, Homare D, Hughes M, Lovatt J, Coleman E, Jackson G (2013). Growing healthy sweet potato: Best practices for producing planting material. ACIAR.
View
|
|
|
Dereje HB, Hvoslef-Eide AK (2020). Optimization of plant growth regulators for meristem initiation and subsequent multiplication of five virus tested elite sweet potato varieties from Ethiopia. African Journal of Biotechnology 19(6):332-343.
Crossref
|
|
|
FAO (2017). FAOSTAT database food and agricultural commodities production/commodities by country, Ethiopia. Food and Agriculture Organization of the United Nations, Rome. [accessed 2018 05, 30].
View
|
|
|
Gayral P, Blondin L, Guidolin O, Carreel F, Hippolyte I, Perrier X, Iskra-Caruana M-L (2010). Evolution of endogenous sequences of banana streak virus: What can we learn from banana (Musa sp.) Evolution? Journal of Virology 84(14):7346-7359.
Crossref
|
|
|
Jones S, Baizan-Edge A, MacFarlane S, Torrance L (2017). Viral diagnostics in plants using next generation sequencing: Computational analysis in practice. Frontiers in Plant Science 8:1770.
Crossref
|
|
|
Kathurima T, Bett B, Miano D, Kim D (2011). Diagnostics of viruses infecting local farmer preferred sweet potato cultivars in Kenya. African Journal of Agricultural Research 6(16):3718-3724.
|
|
|
Kashif M, Pietilä S, Artola K, Jones R, Tugume A, Mäkinen V, Valkonen J (2012). Detection of viruses in sweetpotato from Honduras and Guatemala augmented by deep-sequencing of small-RNAs. Plant Disease 96(10):1430-1437.
Crossref
|
|
|
Kreuze J, Perez A, Galvez M, Cuellar WJ (2017). Badnaviruses of sweetpotato: Symptomless co-inhabitants on a global scale. bioRxiv: 140517.
Crossref
|
|
|
Kreuze JF, Perez A, Untiveros M, Quispe D, Fuentes S, Barker I, Simon R (2009). Complete viral genome sequence and discovery of novel viruses by deep sequencing of small RNAs: A generic method for diagnosis, discovery and sequencing of viruses. Virology 388(1):1-7.
Crossref
|
|
|
Kwak H-R, Kim M-K, Shin J-C, Lee Y-J, Seo J-K, Lee H-U, Jung M-N, Kim S-H, Choi H-S (2014). The current incidence of viral disease in Korean sweet potatoes and development of multiplex RT-PCR assays for simultaneous detection of eight sweet potato viruses. Plant Pathology Journal 30(4):416.
Crossref
|
|
|
Li F, Zuo R, Abad J, Xu D, Bao G, Li R (2012). Simultaneous detection and differentiation of four closely related sweet potato potyviruses by a multiplex one-step RT-PCR. Journal of Virological Methods 186(1-2):161-166.
Crossref
|
|
|
Maliogka VI, Minafra A, Saldarelli P, Ruiz-García AB, Glasa M, Katis N, Olmos A (2018). Recent advances on detection and characterization of fruit tree viruses using high-throughput sequencing technologies. Viruses 10(8):436.
Crossref
|
|
|
Mbanzibwa D, Tugume A, Chiunga E, Mark D, Tairo F (2014). Small RNA deep sequencingâ€based detection and further evidence of DNA viruses infecting sweetpotato plants in Tanzania. Annals of Applied Biology 165(3): 329-339.
Crossref
|
|
|
Nhlapo T, Mulabisana J, Odeny D, Rey M, Rees D (2018). First Report of Sweet potato badnavirus A and Sweet potato badnavirus B in South Africa. Plant Disease 102(9):1865-1865.
Crossref
|
|
|
Tesfaye T, Feyissa T, Abraham A (2011). Survey and serological detection of sweet potato (Ipomoea batatas (L.) Lam) viruses in Ethiopia. Journal of Applied Biosciences 41:2746-2756.
|
|
|
Tesfaye T, Fikre H, Gemu M (2013). Prevalence, incidence and distribution of sweet potato vrus: Its effect on the yield of sweet potato in southern Region of Ethiopia. International Journal of Science and Research 2(1):591-595.
|
|
|
Wang Y-J, Zhang D-S, Zhang Z-C, Wang S, Qiao Q, Qin Y-H, Tian Y-T (2015). First report on sweetpotato symptomless virus 1 (genus Mastrevirus, family Geminiviridae) in sweetpotato in China. Plant Disease 99(7):1042-1042.
Crossref
|
|
|
Zheng Y, Gao S, Padmanabhan C, Li R, Galvez M, Gutierrez D, Fuentes S, Ling K-S, Kreuze J, Fei Z (2017). VirusDetect: An automated pipeline for efficient virus discovery using deep sequencing of small RNAs. Virology 500:130-138.
Crossref
|
|