ABSTRACT
To assay the efficiency of graphite nanoparticles (GtNPs) in sterilizing tissues and their role in enhancing genetic diversity, sweet potato is considered an important crop; hence its explants were used. In this experiment, GtNPs of 200, 400 and 800 ppm concentrations were used for sterilization of MS callus induction and regeneration media in Abees cultivar. The results showed that GtNPs had a good potential for removing bacterial contaminants without having side effects on the explant viability during the sterilization of sweet potato tissue in all their concentrations. Also, the percentage of callus induction increased from 98.67% in control to 100% in all GtNPs concentrations. The number of shoots per callus was enhanced at 400 ppm concentration. RAPD molecular markers and SDS-PAGE analysis were used to assess the genetic diversity of the sweet potato selected plants obtained from somaclonal variations in combination with GtNPs. Five decamer random amplified polymorphic DNA (RAPD) primers generated a total of 96 DNA fragments from the selected variants and their parent. Out of them, 82 polymorphic bands appeared with 85.42% polymorphism. The levels of DNA and protein patterns polymorphism within each treatment varied. RAPD and protein markers revealed that the concentration of 800 ppm showed the lowest similarity average among the ten selected variants and their parent. The obtained results indicated that somaclonal variation with GtNPs can be combined to increase the induced mutations frequency.
Key words: Graphite nanoparticles, somaclonal variation, random amplified polymorphic DNA (RAPD), sodium dodecyl sulfate polyacrylamide gel electrophoresis (SDS-PAGE).
Sweet potato [Ipomoea batatas (L.) Lam], belonging to Convolvulaceae family, is a very important crop in the world. It is a good source of proteins, minerals, vitamins and antioxidants (Pfeiffer and Mclafferty, 2007; Bovell-Benjamin, 2007; Tumwegamire et al., 2011). Due to its commercial importance, the genetic improvement of the plant is needed. The essential way to improve this important crop is through the induction of genetic variations, which can be done by biotechnological interventions such as tissue culture. Using plant tissue culture makes the production of secondary metabolite, genetically modified and disease-free plants possible (Murashige, 1974; Khosroushahi et al., 2006). In spite of the advantages of tissue culture technique, some methodological problems, such as microbial contamination of explants hinder its importance as an advanced technique for biotechnological research (Cassells, 1991).
In modern science, material nanoparticles (NPs) display completely new or enhanced properties based on their size, distribution and morphology. Scientists suggested positive and negative effects of NPs on plants’ growth and development. Many morphological and physiological changes can appear as a result of the interaction between nanoparticles and plants. The chemical composition, concentration, size and physical properties of NPs can determine their efficiency on plants (Ma et al., 2010; Khodakovskaya et al., 2012). Nanoparticles application led to the induction of microbe-free explants and demonstrated the positive role of NPs in callus induction, organogenesis, somatic embryo-genesis, somaclonal variation, genetic transformation and secondary metabolite production (Kim et al., 2017).
Genetic variation resulting from in vitro culture, somaclonal variation is considered to be very useful for developing transgenic plants with desirable agronomic traits (Gaafar and Saker, 2006). Detecting genetic variation of transgenic plants is one of the purposes and criteria for their safety assessment. Random Amplified Polymorphic DNA (RAPD) marker has been used successfully as a molecular marker to characterize, identify and determine variations in nuclear genome between sweet potato genotypes (Gichuki et al., 2003; He et al., 2006; Lin et al., 2009; Moulin et al., 2012; da Silva et al., 2014; Galal and El Gendy, 2017). Also, this technique proved to be able to detect variation among individuals and to estimate the genetic diversity of somaclonal variations in various plant species (Hernandez et al., 2007; Sheidai et al., 2008; Khan et al., 2011; Nasim et al., 2012) including sweet potato (Aboulila, 2016).
Among biochemical markers, Sodium Dodecyl Sulphate Polyacrylamide Gel Electrophoresis (SDS-PAGE) is a useful and inexpensive tool for describing genetic structure of several plant species (Oppong-Konadu et al., 2005; Salimi, 2013). Because of the importance of plant cell and tissue culture, and to avoid contamination, a dominant barrier in this technique, the aim of this work was to discover the effect of graphite nanoparticles (GtNPs) as antimicrobial agents and their role in enhancing somaclonal variations and diversity in sweet potato plants. Genetic diversity was assessed using RAPD molecular markers and SDS-PAGE analysis.
This study was conducted at the Laboratories of Genetics Department, Faculty of Agriculture, Kafrelsheikh University, Egypt.
Plant material
Greenhouse-grown plants of sweet potato, Abees cultivar, were considered as the experimental materials in vitro using nodal cutting system.
Nano material
Graphite nanoparticles (purity 99.9%) and particle size of 1 to 2 nm) were applied in the present study. GtNPs were diluted in double distilled water at concentrations of 200, 400 and 800 ppm and suspended by sonication for 30 min before use.
Preparation and characterization of GtNPs
GtNPs were prepared using the expanded graphite (EGt) method as described by Yu and Qiang (2012). One gram of EGt was immersed in 1000 mL aqueous solution of 75% alcohol and suspended by sonication for 12 h. GtNPs were purified using a filtration process. They were washed with distilled water and then allowed to dry in a thermo-static vacuum oven at 100°C. Physical characterization and diameters of nanoparticles were noticed and measured by a transmission electron microscopy (TEM). The result of the TEM image of GtNPs (Figure 1) showed that the particle sizes are in the range of nano.
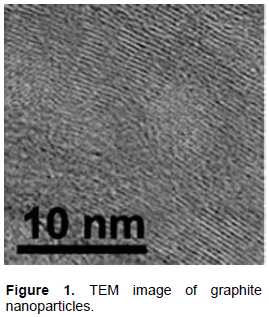
GtNPs treatments, callus induction and regeneration system
To study the effect of GtNPs on callogenic response and antimicrobial effects, stem segments without buds were surface disinfected with 70% EtOH for 30 s, 2.5% NaOCl for 5 min and 0, 200, 400 and 800 ppm GtNPs for 15 min before they were used as explant materials. Explants were cultured on MS (Murashige and Skoog, 1962) medium provided with sucrose (30 g/L), BAP (8 mg/l), myo-inositol (100 mg/l) and three different concentrations of GtNPs (200, 400 and 800 ppm) besides the control. The final pH value was adjusted to 5.8 and the media were solidified with 2 gm/l phytagel. Each treatment consisted of three replicates (five Petri dishes with five explants for each replicate). The explants were incubated at 25±2°C in darkness for 6 weeks with two sub-culturing. Six days after culturing, callugenesis was started. Explants were checked daily for any possible contamination. At the end of callus induction period, microbial contaminants were recorded. Moreover, callus induction percentage was estimated as the percentage of explants that produce callus.
Calli obtained from each treatment were subsequently transferred to shoot induction medium comprising complete MS medium with 100 mg/l myo-inistol and 6.0 mg/l BAP (containing GtNPs graded levels). The observations were recorded after two weeks of incubation for microbial contaminants, percentages of shoot induction (based on number of calli forming shoots) and the number of shoots/callus.
Hardening of in vitro plantlets
Regenerated shoots (3-5 cm in length) were excised from the embryogenic callus, transformed and cultured on half strength MS medium; their pH was adjusted to 5.8. Cultures incubation was done in growth room and maintained under conditions previously mentioned. For acclimatization, the obtained plantlets were hardened as described by Aboulila (2016).
Molecular analysis
Total genomic DNA was isolated from fresh leaves of the parent plant and ten selected somaclonal variants of each of the control and the three GtNPs treatments using the method of CTAB-chloroform as described by Saghai-Maroof et al. (1984). Random amplified polymorphic DNA analysis using five oligonucleotide decamer primers was applied; OPA-20, OPB-01, OPB-05, OPB-07 and OPB-17 (Bio Basic Inc, Canada). The PCR reaction mixture consisted of 0.75 μl of genomic DNA (40 ng), 0.75 μl of 20 μM primer, 5 μL of 2X PCR Master mix Solution (i-TaqTM, iNtRON's Biotechnology) and 3.5 μL of sterile distilled water in a final volume of 10 μl. Amplification condition was performed according to Galal and El Gendy (2017). Amplification products were separated by electrophoresis and bands were detected on Benchtop UV-transilluminator and photographed using Doc- ItTM Imaging System. A known 50 bp DNA Ladder ready-to-use (Cat-no: 300003, GeneON) was run against the PCR products.
Biochemical analysis
Total soluble proteins were obtained from 0.5 g fresh leaves of all selected somaclones with their parent. Sodium dodecyl sulphate polyacrylamide gel electrophoresis (SDS-PAGE) for total soluble protein was done using 12.5% polyacrylamide gel as described by Laemmli (1970). Molecular weights (MW) for all obtained bands were determined by using pre-stained high molecular weight standard marker (PINK Prestained Protein Marker, Cat. No. MWP02), with molecular weights ranging from 15 to 175 kDa.
Data analyses
Data of recorded traits were analyzed statistically as complete randomized design in three replicates (n=5); the mean values obtained from the treatments were compared by the least significant differences (LSD) test at significance level of P≤ 0.05 using the SXW program.
Molecular and biochemical data were introduced to SPSS package program as: Binary value of 1 for visible band and 0 for absent band; genetic similarity was estimated using Jaccard's similarity coefficient (Jaccard, 1901).
Effect of GtNPs on tissue culture
The influence of different concentrations of GtNPs (0, 200, 400 and 800 ppm) was evaluated by adding these concentrations to callus induction and shoot induction media. Results in Table 1 and Figure 2 show that regeneration capacity (shoot induction % and number of shoots/callus) was affected by the concentration of GtNPs. Callus induction percentage did not differ significantly in all treatments, which varied from 98.67% in control to 100% in all GtNPs concentrations. Also, in control treatment all of the calli were regenerable (100%) followed by 400 ppm concentration which regenerated 96.33% from all cultured calli on regeneration medium. Plant regeneration and production of multiple shoots from callus were obtained after four to five weeks from callus initiation. While 400 ppm GtNPs recorded the highest number of shoots per callus (13.73) in regeneration medium, the other treatments recorded regeneration capacities ranging from 6.67 to 8.67. These results agree with those of Lahiani et al. (2016) who used carbon-based nanomaterials (CBNs) on tobacco cell culture to increase growth (22-46%) by addition of 50 μg/l.
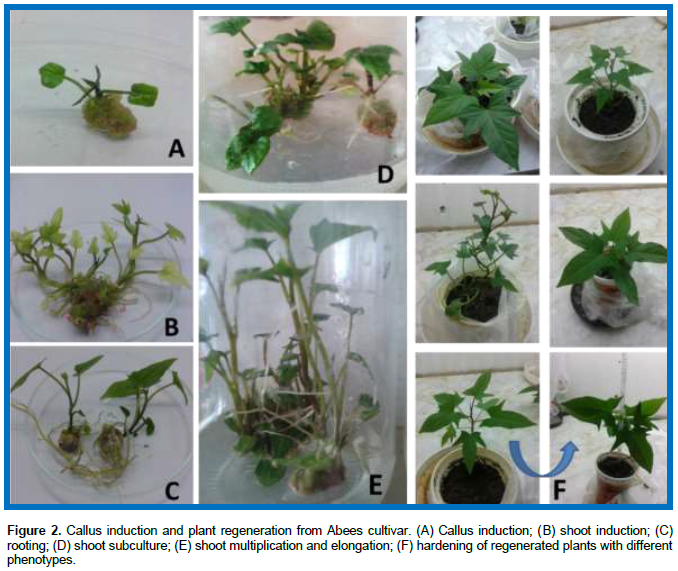
Moreover, Khodakovskaya et al. (2012) reported that the incorporation of 100 mg/l multi-walled carbon nanotubes into a medium containing 1 mg/l 2,4-D increased callus growth of tobacco explants (64% increase over control). The treatment with carbon nanotubes enhanced callus growth by upregulation of cell division genes (CycB) and water transport (NtPIP1). However, carbon nanotube treatment (10-600 mg/l) decreased cell viability and dry weight in Arabidopsis (Lin et al., 2009). Hence, from all of these reports it can be summarized that the addition of NPs to a plant tissue culture medium affects callus proliferation, shoot multiplication, somatic embryogenesis and rooting by altering antioxidant enzyme activities, gene expression and production of ROS.
Changes observed in developed organs and plantlets are termed somaclonal variation. In this study, some of the obtained regenerated plants after adaptation showed morphological differences compared to their parent, Abees cultivar (Figure 2F). These morphological differences are usually associated with changes in chromosome number, chromosome structure, DNA sequence, DNA methylation and mitotic crossing over (Bairu et al., 2011; Sivanesan and Jeong, 2012).
Positive effects of nanoparticles appeared on callus induction, shoot regeneration and growth in several studies. Aghdaei et al. (2012) found that shoot induction percentage, number of shoots and callus formation increased when culturing the stem explants from Tecomella undulata (Roxb) on MS media containing 10 mg/l AgNPs. NPs treatments affect the mitotic index and DNA integrity, and alter the protein and DNA expression in plants (Atha et al., 2012; Landa et al., 2015; Tripathi et al., 2017).
Identification of anti-microbial effect of GtNPs
The efficiency of GtNPs in decontaminating diverse kinds of explants was evaluated. So, we used different concentrations of GtNPs to find the best sterilization treatment. Sweet potato explants were cultured on GtNPs and GtNPs-free media for callus induction and regeneration to study the elimination of microorganisms in all procedures of tissue culture after four weeks. The results showed that, treating sweet potato stem segments with 200, 400 and 800 mg/l GtNPs for 15 min, there were no contaminants with no effects on organogenesis. Moreover, contaminations were observed only in control treatment (GtNPs-free media) during the different stages in culture media, while microbial contaminants were absent in all GtNPs concentrations. These results showed that GtNPs were effective in the suppression of microbial contaminants in all concentrations.
When GtNPs of 200, 400 and 800 ppm concentrations were applied on sweet potato explant segments, they could function as antimicrobial agents leaving no harmful effect on explants and their viability, and were all able to produce callus. It has been illustrated that the toxicity of GtNPs to microbial cells is apparent even at the range of 200 to 800 ppm concentrations. Several types of NPs such as silver (Ag), aluminum oxide (Al2O3), copper oxide (CuO), iron oxide (Fe3O4), gold (Au), magnesium oxide (MgO), nickel (Ni), silicon (Si), silicon dioxide (SiO2), titanium dioxide (TiO2), GtNPs and zinc oxide (ZnO) have been reported to possess antimicrobial activities against various microorganisms (Liu et al., 2011; Gouran et al., 2014; Beyth et al., 2015).
Genetic diversity in sweet potato using RAPD and protein markers
Genetic polymorphism using RAPD analysis
Five oligonucleotide random primers were employed to study the genomic stability in sweet potato parental cultivar (Abees) along with its somaclonal variants obtained from tissue culture combined with GtNPs (Figure 3). Among the primers used, OPB-17 generated the highest number of total bands (27 bands), while primer OPB-05 produced the lowest (13 bands). All primers generated a total of 96 DNA bands. Fourteen bands were monomorphic and consistent among all selected variants for all treatments; however, 82 bands were observed to be polymorphic with 85.42% polymorphism. The highest level of polymorphism (96.30%) was recorded in primer OPB-17 while the lowest level of polymorphism was 72.22% in primer OPB-07 (Table 2).
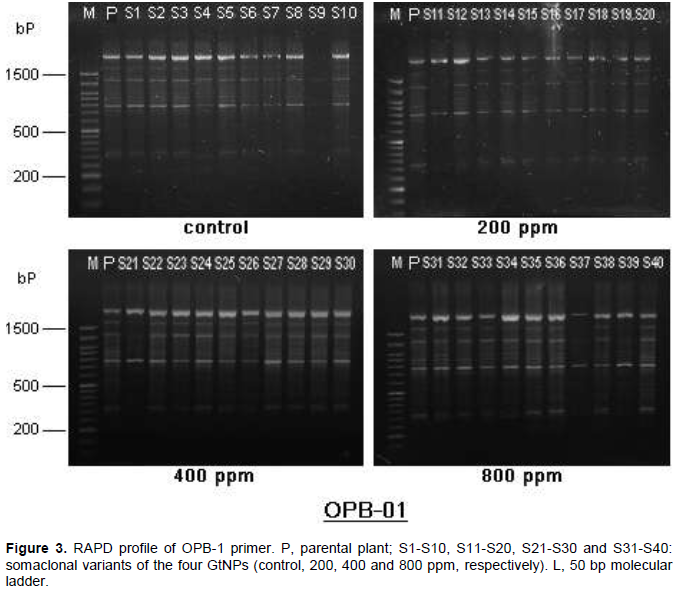
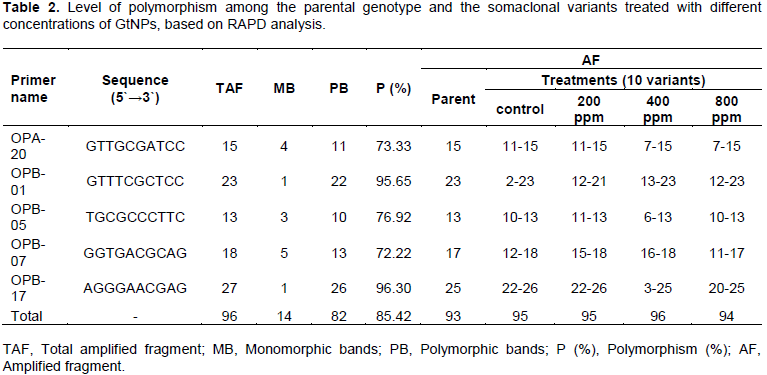
On the other hand, the five primers revealed a total of 93 bands in the parental genotype. Out of them, 52, 58, 35 and 47 bands were common in the parental genotype and the somaclonal variants obtained from the four treatments; control, 200, 400 and 800 ppm, respectively (Table 3). The level of polymorphism among the somaclonal variants and the parental genotype varied. The highest number of polymorphic bands (61 bands) was recorded in 400 ppm concentration with 63.54% polymorphism, while the lowest number (37 bands) was noticed in 200 ppm with 38.95% polymorphism. The genetic polymorphism increased in the regenerated plants at 400 and 800 ppm, while it decreased at 200 ppm compared to the control.
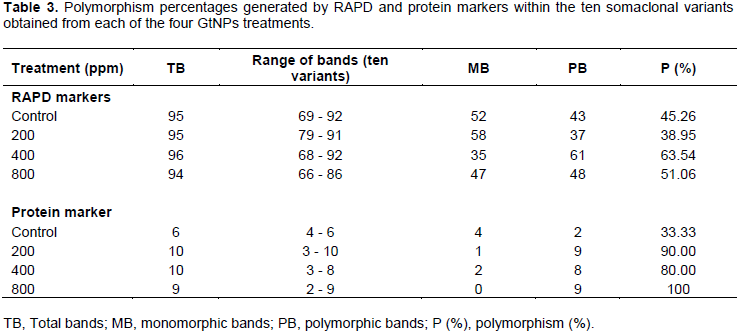
Variations observed in the total number of RAPD bands among the parental genotype and plants generated from tissue culture combined with different GtNPs concentrations indicate genetic differences of the variants because of somaclonal variation induced from tissue culture as seen in control treatment, plus the genetic variation induced by GtNPs treatments. This is in agreement with Sheidai et al. (2008) who found that some bands appeared in the parental plants and got lost in regenerated plants because of somaclonal variation. These results proved that RAPD markers were effective in detecting polymorphism which occurs due to insertion, deletions and base substitution that affect the primer-binding site and reflect as the presence or loss of bands. These findings are in agreement with earlier studies using RAPD analysis in describing genetic polymorphism among somaclonal variants in various plant species. Khan et al. (2011) used this technique to determine the genetic variations among micropropagated banana plants.
Genetic polymorphism based on SDS-PAGE analysis
Protein banding patterns were used to detect the genetic variations among the ten selected variants within the four studied treatments (Figure 4). The electrophorotic patterns of SDS-protein revealed marked polymorphism within each GtNPs treatment as shown in Table 3. Polymorphism percentage within the four treatments ranged from 33.33 to 100%. Control treatment revealed the lowest polymorphic percentage (33.33%) indicating that level of polymorphism differed a little within the control treatment. Also, 800 ppm treatment gave the highest polymorphic percentage (100%); a total of nine bands ranging from 2 to 9 were detected and all of them were polymorphic. For the other two treatments (200 and 400 ppm), the profile of variants and their parent exhibited the highest number of protein bands (10 bands for each) which showed 90 and 80% polymorphism, respectively.
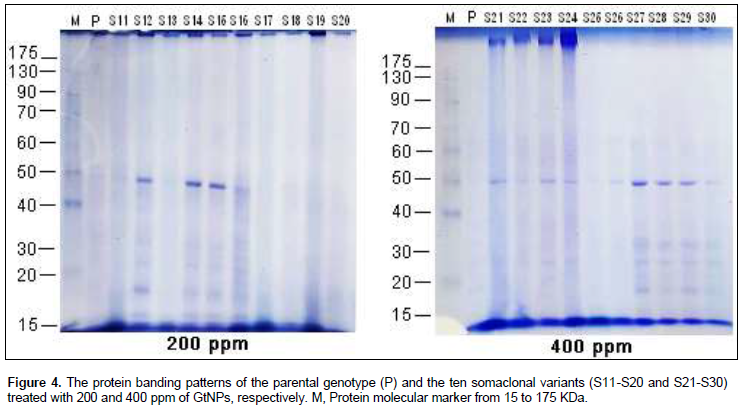
Results obtained from many studies have shown that much of the genetic variability generated from plant tissue culture may be the result of gene mutation (D’Amato, 1985; Ngezahayo et al., 2007) or epigenetic variation (Kaeppler et al., 2000; Guo et al., 2006; Smulders and de Klerk, 2011). It is likely that these variations are based on the differences in GtNPs concentrations, media used for culture or their combinations. This is in contrast with the results of Afrasiab and Iqbal (2010), who noted that the recovery of somaclones can be increased by combining micro-propagation with induced mutagenesis in vitro.
Genetic similarity
The ranges and averages of similarity values for the ten somaclonal variants and their parent within each of the GtNPs treatments (200, 400 and 800) and control based on RAPD and protein markers are listed in Table 4. RAPD and protein markers revealed that the concentration of 800 ppm showed the lowest similarity average among the ten selected variants and their parent. In the case of RAPD marker, all treatments revealed high similarity averages ranging from 0.816 (800 ppm) to 0.867 (200 ppm), with a mean value of 0.843, indicating high homogeneity within the tested treatments. While, the genetic similarity decreased with GtNPs increase from 200 to 800 ppm, indicating that genetic variations induced in the regenerated plants increase with the concentrations of GtNPs. Concerning protein marker, the similarity mean value of 0.608 was obtained from all tested treatments. The highest genetic similarity average was found within control treatment (0.850), while the lowest one was observed within 800 ppm concentration (0.416). However, 200 and 400 ppm treatments showed genetic similarity averages with 0.563 and 0.605, respectively.
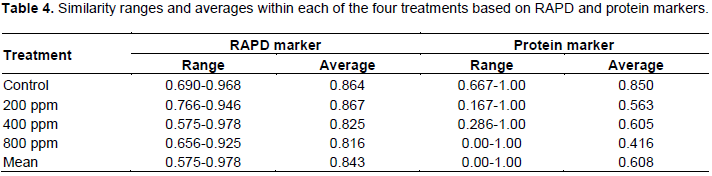
Therefore, analyses of RAPD and SDS-PAGE appeared to be effective for assessing genetic similarity of sweet potato somaclonal variants and their parent within each GtNPs treatment. These results are in line with those of Metry et al. (2002) who used RAPD markers and SDS-PAGE analysis to identify genetic similarity among transgenic potato cultures.
In conclusion, results showed that genetic variations occurred due to the differences generated from somaclonal variation during tissue culture combined with that generated from GtNPs treatments. Somaclonal variations and GtNPs can be combined to increase induced mutations frequency. Mutation, which changes one or few specific traits of a cultivar, can be utilized for selection of desired traits in sweet potato to crop improvement. The obtained variants could be used for more evaluation to test their commercial biosafety.
The authors have not declared any conflict of interests.
REFERENCES
Aboulila AA (2016). Molecular genetic diversity and efficient plant regeneration system via somatic embryogenesis in sweet potato (Ipomoea batatas (L.) Lam.). Egyptian Journal of Genetics and Cytology 45:347-365.
|
|
Afrasiab H, Iqbal J (2010). In vitro techniques and mutagenesis for the genetic improvement of potato cvs. Desiree and Diamant. Pakistan Journal of Botany 42:1629-1637.
|
|
|
Aghdaei M, Salehi H, Sarmast MK (2012). Effects of silver nanoparticles on Tecomella undulata (Roxb) Seem, micropropagation. Advances in Horticultural Science 26:21-24.
|
|
|
Atha DH, Wang H, Petersen EJ, Cleveland D, Holbrook RD, Jaruga P, Dizdaroglu M, Xing B, Nelson BC (2012). Copper oxide nanoparticle mediated DNA damage in terrestrial plant models. Environmental Science and Technology 46:1819-1827.
Crossref
|
|
|
Bairu MW, Aremu AO, Van Staden J (2011). Somaclonal variation in plants: causes and detection methods. Plant Growth Regulation 63:147-173.
Crossref
|
|
|
Beyth N, Houri-Haddad Y, Domb A, Khan W, Hazan R (2015). Alternative Antimicrobial Approach: Nano-Antimicrobial Materials. Evidence-based complementary and alternative medicine 246012.
Crossref
|
|
|
Bovell-Benjamin A (2007). Sweet potato: a review of its past, present and future role in human nutrition. Advances in Food and Nutrition Research 52:1-59.
Crossref
|
|
|
Cassells AC (1991). Problems in tissue culture: Culture contamination. In: Micropropagation: technology and application (Eds. Debergh, P.C. and Zimmerman, R.H.), Springer.
Crossref
|
|
|
D'Amato F (1985). Cytogenetics of plant cell and tissue cultures and their regenerants. Critical Reviews in Plant Sciences 3:73-112.
Crossref
|
|
|
da Silva AVC, Andrade LNT, Rabbani ARC, Nunes MUC, Pinheiro LR (2014). Genetic diversity of sweet potatoes collection from North-eastern Brazil. African Journal of Biotechnology 13:1109-1116.
Crossref
|
|
|
Gaafar RM, Saker MM (2006). Monitoring of cultivars identity and genetic stability in strawberry varieties grown in Egypt. World Journal of Agricultural Sciences 2:29-36.
|
|
|
Galal OA, El Gendy ASA (2017). Genetic characterization of three Egyptian sweet potato genotypes based on morpho-agronomic and molecular markers. Egyptian Journal of Genetics and Cytology 46:283-296.
|
|
|
Gichuki ST, Berenyi M, Zhang D, Hermann M, Schmidt J, Glössl J, Burg K (2003). Genetic diversity in sweet potato [Ipomoea batatas (L.) Lam.] in relationship to geographic sources as assessed with RAPD markers. Genetic Resources Crop Evolution 50:429-437.
Crossref
|
|
|
Gouran A, Jirani M, Mozafari AA, Saba MK, Ghaderi N, Zaheri S (2014). Effect of silver nanoparticles on grapevine leaf explants sterilization at in vitro conditions, 2nd National Conference on Nanotechnology from Theory to Application, Isfahan, Iran pp. 1-6.
|
|
|
Guo W, Gong L, Ding Z, Li Y, Li F, Zhao S, Liu B (2006). Genomic instability in phenotypically normal regenerants of medicinal plant Codonopsislanceolata Benth. et Hook. f., as revealed by ISSR and RAPD markers. Plant Cell Reports 25:896-906.
Crossref
|
|
|
He XQ, Liu QC, Ishiki K, Zhai H, Wang YP (2006). Genetic diversity and genetic relationships among Chinese sweetpotato landraces revealed by RAPD and AFLP markers. Breeding Science 56:201-207.
Crossref
|
|
|
Jaccard P (1901). Étude comparative de la distribuition florale dans une portion des Alpes et des Jura. Bulletin de la Société vaudoise des sciences naturelles 37:547-579.
|
|
|
Kaeppler SM, Kaeppler HF, Rhee Y (2000). Epigenetic aspects of somaclonal variation in plants. Plant Molecular Biology 43:179-188.
Crossref
|
|
|
Khan S, Saeed B, Kauser N (2011). Establishment of genetic fidelity of in vitro raised banana plantlets. Pakistan Journal of Botany 43:233-242.
|
|
|
Khodakovskaya MV, de Silva K, Biris AS, Dervishi E, Villagarcia H (2012). Carbon nanotubes induce growth enhancement of tobacco cells. ACS Nano 6(3):2128-2135.
Crossref
|
|
|
Khosroushahi AY, Valizadeh M, Ghasempour A, Khosrowshahli M, Naghdibadi H, Dadpour MR, Omidi Y (2006). Improved Taxol production by combination of inducing factors in suspension cell culture of Taxusbaccata. Cell Biology International 30:262-269.
Crossref
|
|
|
Kim DH, Gopal J, Sivanesan I (2017). Nanomaterials in plant tissue culture: the disclosed and undisclosed. The Royal Society of Chemistry (RSC) Advances 7:36492-36505.
|
|
|
Laemmli UK (1970). Clavage of structural protein during assembly of head bacteriophage T4. Nature 227:680-685.
Crossref
|
|
|
Lahiani MH, Dervishi E, Ivanov I, Chen J, Khodakovskaya M (2016). Comparative study of plant responses to carbon-based nanomaterials with different morphologies. Nanotechnology 27(26):265102.
Crossref
|
|
|
Landa P, Prerostova S, Petrova S, Knirsch V, Vankova R, Vanek T (2015). The transcriptomic response of arabidopsis thaliana to zinc oxide: A comparison of the impact of nanoparticles, bulk, and ionic zinc. Environmental Science and Technology 49:14537-14545.
Crossref
|
|
|
Lin C, Fugetsu B, Su Y, Watari F (2009). Studies on toxicity of multi-walled carbon nanotubes on Arabidopsis T87 suspension cells. Journal of Hazardous Materials 170:578-583.
Crossref
|
|
|
Liu SB, Zeng TH, Hofmann M, Burcombe E, Wei J, Jiang R, Kong J, Chen Y (2011). Antibacterial activity of graphite, graphite oxide, graphene oxide, and reduced graphene oxide: membrane and oxidative stress. ACS Nano 5(9):6971-6980.
Crossref
|
|
|
Ma X, Geiser-Lee J, Deng Y, Kolmakov A (2010). Interactions between engineered nanoparticles (ENPs) and plants: phytotoxicity, uptake and accumulation. Science of the Total Environment 408(16):3053-3061.
Crossref
|
|
|
Metry E, Enan M, Gad El-Karim GH, Nasr El-Din T, Madkour M (2002). Production of genetically modified potato plants for fungal resistance. Arab Journal of Biotechnology 5:11-18.
|
|
|
Moulin MM, Rodrigues R, Gonçalves LSA, Sudré CP, Pereira MG (2012). A comparison of RAPD and ISSR markers reveals genetic diversity among sweet potato landraces (Ipomoea batatas (L.) Lam.). Acta Scientiarum Agronomy 34:139-147.
Crossref
|
|
|
Murashige T (1974). Plant propagation through tissue cultures. Annual review of plant physiology 25:135-166.
Crossref
|
|
|
Murashige T, Skoog F (1962). A revised medium for rapid growth and bioassays with tobacco tissue cultures. Physiologia Plantarum 15:473-497.
Crossref
|
|
|
Nasim G, Khan S, Khokhar I (2012). Molecular polymorphism and phylogenetic relationship of some alternaria alternata isolates. Pakistan Journal of Botany 44(4):1267-1270.
|
|
|
Ngezahayo F, Dong Y, Liu B (2007). Somaclonal variation at the nucleotide sequence level in rice (Oryza sativa L.) as revealed by RAPD and ISSR markers, and by pairwise sequence analysis. Journal of Applied Genetics 48:329-336.
Crossref
|
|
|
Oppong-Konadu EYR, Akromah RK, Adu-Dapaah H, Kai EO (2005). Genetic diversity within Ghanaian cowpea germ-plasm based on SDS-PAGE of seed proteins. African Crop Science Journal 3:117-123.
|
|
|
Pfeiffer WH, Mclafferty B (2007). Harvest-Plus: Breeding crops for better nutrition. Crop Science 47:88-105.
Crossref
|
|
|
Saghai-Maroof MA, Soliman KM, Jorgensen RA, Allard RW (1984). Ribosomal DNA spacer-length polymorphisms in barley: Mendelian inheritance, chromosomal location, and population dynamics. Proceedings of the National Academy of Sciences 81(24):8014-8018.
Crossref
|
|
|
Salimi S (2013). Relationships of some soybean genotypes based on morphological characters and biochemical marker. International Journal of Agronomy and Plant Production 4:2237-2243.
|
|
|
Sheidai M, Aminpoor H, Noormohammadi Z, Farahani F (2008). RAPD analysis of somaclonal variation in banana (Musa acuminate L.) cultivar Valery. Acta Biologica Szegediensis 52(2):307-311.
|
|
|
Sivanesan I, Jeong BR (2012). Identification of somaclonal variants in proliferating shoot cultures of Senecio cruentus cv. Tokyo Daruma. Plant Cell, Tissue Organ Culture 111:247-253.
Crossref
|
|
|
Smulders M, de Klerk G (2011). Epigenetics in plant tissue culture. Plant Growth Regulation 63:137-146.
Crossref
|
|
|
Tripathi DK, Shweta S, Singh S, Pandey R, Singh VP, Sharma NC, Prasad SM, Dubey NK, Chauhan DK (2017). An overview on manufactured nanoparticles in plants: Uptake, translocation, accumulation and phytotoxicity. Plant Physiology and Biochemistry 110:2-12.
Crossref
|
|
|
Tumwegamire S, Rubaihayo PR, Labonte DR, Diaz F, Kapinga R, Mwanga ROM, Grüneberg WJ (2011). Genetic diversity in white- and orange-fleshed sweetpotato farmer varieties from East Africa evaluated by simple sequence repeat markers. Crop Science 51:1132-1142.
Crossref
|
|
|
Yu X, Qiang L (2012). Preparation for graphite materials and study on electrochemical degradation of phenol by graphite cathodes. Advances in Materials Physics and Chemistry 2:63-68.
Crossref
|
|