ABSTRACT
The effect of including palm oil sludge in cattle diet (silage) was conducted in vitro in order to ascertain the suppressing effect on ruminal methanogenesis. The silage used for the study was Panicum maximum, Centrocema pubscens, Bracharia decumbens and Andropogon gayanus. The silage were cut into an average size of 3 to 5 cm and ensiled for 21 days. Four treatment diets were formulated with the silage by including palm oil sludge at 0, 10, 20 and 30%, respectively. Treatments were subjected to anaerobic digestion in 10 L bio-digesters. Total gas production, percentage methane production, volatile fatty acids and microbial population of the substrate were measured. Total gas production was significantly suppressed in the treatment having 30% palm oil sludge for about 25 days while a lag phase of 16 days was observed before a significant amount of methane was detected. Acetic acid production increased only in T1 on the first week while T2, T3 and T4 decreased after which their production remained irregular until the end of the experiment. Propionic acid production also increased in T1 and T4 on the first week while T2 and T3 decreased after which their production maintained an undefined pattern as the experiment progressed. Butyric acid production increased in T1, T2, and T4 on the first week while T2 decreased after which an undefined trend of production was established. Microbial population especially methanogens also reached its peak around the same period when there was maximum gas production. It was concluded that ruminal methanogenesis can be suppressed in-vitro by the inclusion of 30% palm oil sludge in the silage.
Key words: Bio-digesters, fermentation, in-vitro, methane, silage.
Abbreviation:
MCA, MacConkey agar; CRD, completely randomized design; NA, nutrient agar.
Methane emission from ruminant animals has become a serious issue that attracts global attention. Methane remains one of the greenhouse gases that cause climate change. Report shows that enteric methane is the most important greenhouse gas emitted (50 to 60%) at the farm scale in ruminant production systems (Ogino et al., 2007). Methane has the global warming potential of 25 and with longer residence time when compared to carbondioxide with higher global warming potential of 82 and shorter residence time (Wuebbles and Hayhoe, 2002; Forster et al., 2007). Human activities accounts for 70% of methane production and its rising concentration is strongly correlated with increasing population of ruminants (Moss et al., 2000; IPCC, 2007).
Murray et al. (1976) stated that about 90% enteric methane produced by ruminants has its origin in the rumen. Methane mitigation strategies in ruminants have been reviewed critically by several authors. Mitigation strategies notably on animal phenotype, biotechnologies and additives were discussed but nutrition strategies were described as the most developed and ready to be applied in the field. Smink et al. (2004), Van Zijderveld and Van Straalen (2004) and Van Laar and Van Straalen (2004) noted that several Rob-Agro studies have been performed and the effect of nutrition and feed additives on methane emission evaluated. Lin et al. (2013) had reported promising effect of different essential oils in modulating rumen function in vitro. According to Hristov et al. (2013) the inclusion of lipids in the diets of ruminants is an effective strategy for reducing enteric methane emission, but the applicability of this practice will depend on its cost effectiveness and its effects on feed intake, productivity and milk production. Hence, the inclusion of palm oil sludge in ruminant diets may have a great potential in reducing enteric methane emission since it contains more phyto-nutrients (vitamin E, vitamin K and alpha carotene, beta-carotene, lycopene and 20 other carotenes) than any other dietary oil. These nutrients are powerful antioxidants that protect the oil from oxidation. It is a waste and constitutes nuisance around households and palm oil processing factories. This study was therefore conducted to ascertain the efficacy and effective levels of palm oil sludge in reducing ruminal methanogenesis in vitro.
Experimental diets and design
A mixture of forage materials which comprises of Panicum maximum, Centrocema pubscens, Bracharia decumbens and Andropogon gayanus were mixed in equal proportion for the production of silage. The forage materials were cut to a size of between 3 to 5 cm and ensiled for 21 days. The silage were mixed with palm oil sludge at 0, 10, 20 and 30% inclusion levels to form four treatment diets with four replicate each. The treatment diets were subjected to anaerobic digestion in 10 L bio-digesters as the in vitro rumen simulation. 5.8 kg of water, 2 kg of treatment diets and 0.2 kg of rumen liquor were put in a 10 L biodigesters while the remaining space was left for gas accumulation. The treatments were replicated four times in a completely randomized design (CRD). Total gas production and percentage methane obtained were recorded using the Sperian gas analyzer; model number SN 66429, USA. Total gas volume was obtained through downward displacement of water in gasometers. The volume of water displaced therefore equals volume of gas produced. Sperian gas analyser recorded the percentage of methane in the total gas and that percentage was used to calculate the volume of methane fromtotal gas volume. Gas collection and analysis were done every 24 h for 90 days.
The diets were analyzed for crude protein, ash, fats, moisture and fibre contents before and after the fermentation process according to AOAC (2000). Changes in the concentration of volatile fatty acids (acetic, propionic and butyric) were also determined biweekly during the fermentation period for 90 days using Spectrophotometer (BB Bran England model 7804 C) in a diluted volumes of 0.1 ml in 10 ml samples. Changes in total microbial population of the anaerobic fermentation medium were determined through laboratory methods in food microbiology by Harrigan and McCance P.25-28.
Preparation of diluent and media
Diluent (peptone water) and media (Nutrient agar) were prepared according to manufacturer’s specification.
Microbiological analysis of samples
One milliliter (1 ml) of each sample was serially transferred into 9 ml of the sterile diluent (peptone water) with a sterile pipette and shaken vigorously. Serial dilution was continued until 106 dilution was obtained.
Aliquot portion (0.1 ml) of the 106 and was inoculated onto freshly prepared, surface-dried nutrient agar (NA). The inoculi were spread with a sterile (hockey stick-like) glass spreader to obtain even distribution of isolates after incubation. Nutrient agar and MacConkey agar plates were incubated for 24 to 48 h at 37°C. Anaerogen was used during the incubation process.
Enumeration of microbial population
Total plate counts for the nutrient and MacConkey Agar were done by counting colonies at the reverse side of the culture plates. Total colony count was expressed in colony forming units per millilitre (cfu/ml) (Harrigan and McCance, 1990).
The proximate composition of the treatment diets that were subjected to anaerobic fermentation is presented in Table 1. Figure 1 shows the methane production pattern during the experiment. Methane production rate from T4 decreased significantly at the beginning of the curve probably as a result of the inhibitory effect of palm oil sludge. This inhibitory effect was maintained for aboutnine days before it declined. Though, the rate of methane production from T4 was still relatively lower until after 72 days before it started rising above others and reached its peak around 87th day. This was similar to the ones reported by Broughton et al. (1988), Salminen et al. (2000) and Cirne et al. (2007).
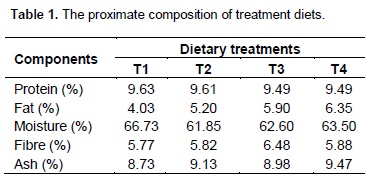
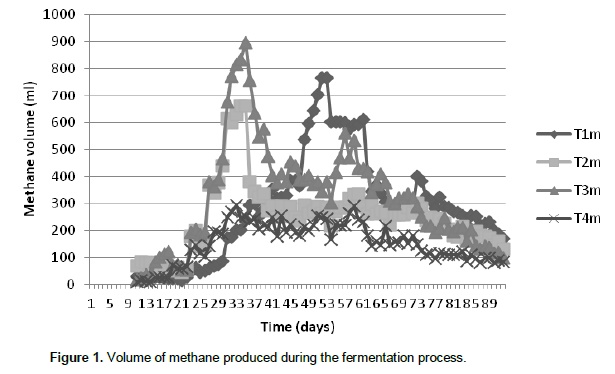
The initial lag phase in methane production as was observed may be attributed to the variation in the concentration of palm oil sludge. Significant methane production was first detected on the 16th day of the digestion process. The volume of methane produced after the lag phase was lowest in treatment 4 (30% palm oil sludge) than in the other treatments. The lowest level of methane production in T4 was maintained for almost 9 days, after which the activities of the methanogens increased and methane volume started to rise just as it was observed in other treatments. The periodic volume of methane production was almost the same in T1, T2 and T3. The variability in methane production was attributed to the different levels of palm oil sludge used in the study. This tends to show that palm oil sludge is capable of suppressing methane production in vitro. Kramer et al. (2013) reported that numerically longer retention time of grass silage-based rations (46.9 ±â€…5.68 h) compared with corn silage-based rations (36.9 ±â€…4.28 h) was in accordance with Lund (2002). The lag phase of 16 days implies that the inhibitory effect of the treatments might also be high if applied in vivo. Treatment 4 (30% palm oil sludge) produced the highest volume of methane toward the end of the fermentation period when the level of palm oil sludge in diet 4 could no longer suppress methane production since the bacteria have acclamatized themselves and can no longer be suppressed by oil. The highest volume of methane produced towards the end of the fermentation may not affect the applicability of the research result since it is assumed to be the period when the silage must have been digested in the animal and will be in form of manure if it were to be in vivo. Therefore, the inclusion of palm oil sludge in cattle diet can help to suppress methane production in vitro. Peak gas production was observed between 3 to 4 weeks of fermentation for all the treatments and this was also the period when microbial population reached its peak as shown in Figure 2.
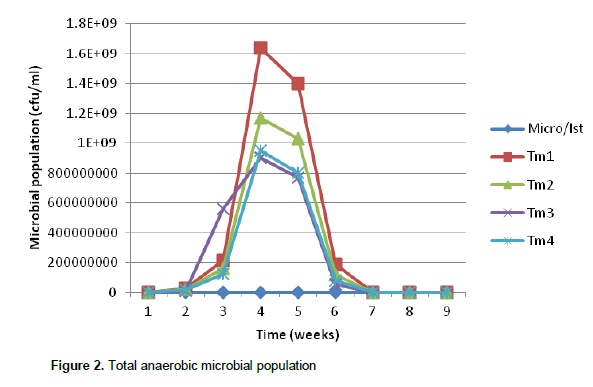
This shows that the volume of gas produced depends on the microbial load present. The variation in microbial population present in the different substrates also showed that the palm oil sludge had an effect on the preponderance of the microbes since the highest population was recorded in treatment 1(0% palm oil sludge) and lowest in treatment 4 (30% palm oil sludge). It is clear that oil coating can make a barrier to microbial attachment to feed particles in the rumen thereby reducing their activities (Narimani-Rad et al., 2012). Methanogens co-exist with several anaerobes to produce methane from carbon dioxide and hydrogen. Hence, the major part of the hydrogen formed in the rumen is converted into methane (Mills et al., 2001). Murphy et al. (1982), Argyle and Baldwin (1988), Pitt et al. (1996), Friggens et al. (1998) and Bannink et al. (2006a) observed that different types of fermented carbohydrate give different profiles of volatile fatty acid production and hence methane yield. Figures 3, 4 and 5 show the production of volatile fatty acids during the fermentation process. The acetic acid concentration followed the same trend except in treatment 1(0% palm oil sludge) that contain no palm oil sludge. As shown by Figure 3, treatment 4 had the highest concentration of acetic acid as compared to the three treatments that contain palm oil sludge. However, the concentration dropped around 50th day of the fermentation process.
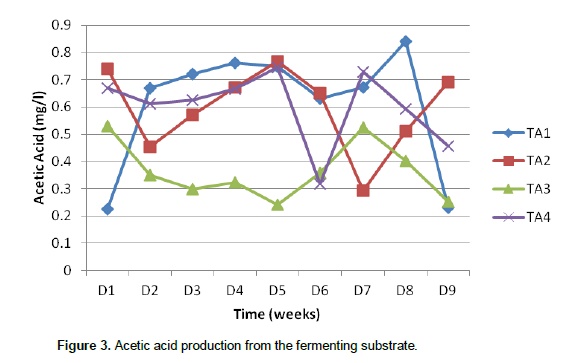
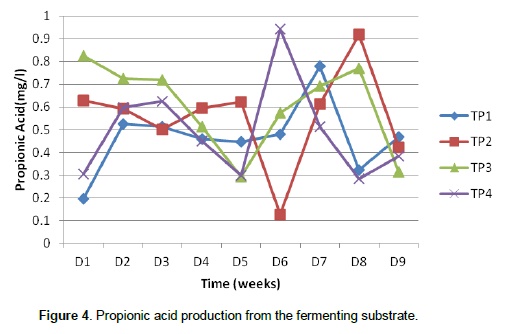
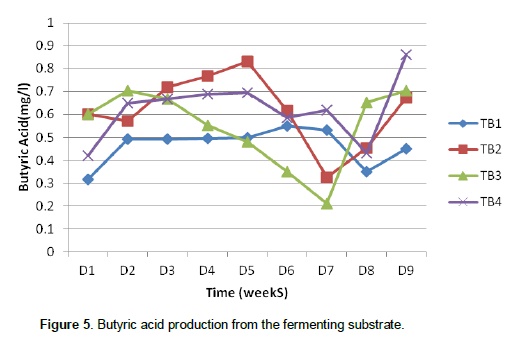
Kohn and Boston (2000) and Offner and Sauvant (2006) noted that during acetogenesis, acetate rather than methane is formed from CO2 and H2. Therefore, the removal of H2 by acetogenesis reduces methane yield since H2 and CO2 can be converted to acetate by acetogens which are also present in an anaerobic environment (Johnson and Johnson, 1995; Demeyer and Fievez, 2000; Moss et al., 2000). The production pattern of propionic acid was slightly different from that of acetic acid since their production from different treatments did not behave alike by either increasing or decreasing at the same time. Propionic acid concentration in T1 and T4 followed the same trend of production by increasing and decreasing at the same time while the trend in concentration of propionic acid in T2 and T3 were the same. However, butyric acid concentration followed the same trend. There was relatively enhanced propionic acid production in the first 2 weeks of production and a concurrent reduction in the concentration of acetic acid. This is in line with the earlier assertion that enhanced propionic acid concentration reduces methane production and at the same time reduces acetic and butyric acid concentration.
An in vitro rumen simulation technique was conducted to determine the effect of including palm oil sludge on methane production in the diet (silage) of Cattle. The results of the experiment have shown that inclusion of 30% palm oil sludge in silage is capable of suppressing methanogenesis in vitro. The increase in the concen-tration of acetic, propionic acid and butyric acid on the first week shows that palm oil sludge in the diet of cattle affect the concentration of volatile fatty acids. The reduction in methane production for a period of 9 days at 30% inclusion of palm oil sludge as was observed in this experiment also supports the applicability of this result since there is an average retention period of 45 h in animals.
The author(s) did not declare any conflict of interest.
MCA, MacConkey agar; CRD, completely randomized design; NA, nutrient agar.
REFERENCES
Argyle JL, Baldwin RL (1988). Modeling of rumen water kinetics and effects of rumen pH changes. J. Dairy Sci. 71:1178-1188.
Crossref |
|
Bannink A, Kogut J, Dijkstra J, Kebreab E, France J, Tamminga S, Van Vuuren AM, (2006a). Estimation of the stoichiometry of volatile fatty acid production in the rumen of lactating cows. J. Theor. Biol. 238: 36-51
Crossref |
|
Broughton MJ, Thiele JH, Birch EJ, Cohen A (1998). Anaerobic batch digestion of sheep tallow. Water Res. 32(5):1423-1428.
Crossref |
|
Cirne DG, Paloumet X, Bjo¨ rnsson L, Alves M M, Mattiasson B (2007). Anaerobic digestion of lipid-rich waste-Effects of lipid concentration. Renew. Energy 32 (6): 965–975.
Crossref |
|
Demeyer D, Fievez V (2000). Ruminants et environment: la méthanogenèse. Ann. Zootech 49:95-112
Crossref |
|
Friggens NC, Oldham JD, Dewhurst RJ, Horgan G (1998). Proportions of volatile fatty acids in relation to the chemical composition of feeds based on grass silage. J. Dairy Sci. 81:1331-1344.
Crossref |
|
Hristov AN, Oh J, Lee C, Meinen R, Montes F, Ott R, Firkins J, Rotz A, Dell C, Adesogan A, Yang W, Tricarico J, Kebreab E, Waghorn G, Dijkstra J, Oosting S, Gerber PJ, Henderson B, Makkar H (2013). Mitigation of Greenhouse Gas Emissions in Livestock Production: A Review of Technical Options for Non-CO2 Emissions. FAO, Rome, Italy (in press). |
|
IPCC (Intergovernmental Panel on Climate Change) (2007). Climate Change 2007: Mitigation of Climate Change. Contribution of Working Group III to the Fourth Assessment. Report of the Intergovernmental Panel on Climate Change, eds Metz B, Davidson OR, Bosch PR, Dave R, Meyer LA (Cambridge University Press, Cambridge, United Kingdom and New York, NY, USA). |
|
Johnson KA, Johnson DE (1995). Methane emissions from cattle. J. Anim. Sci. 73:2483-2492. |
|
Kohn RA, Boston RC (2000). The role of thermodynamics in controlling rumen metabolism. In: Modelling Nutrient Utilization in Farm Animals. Eds. J.P. McNamara, J. France & D.E. Beever. CAB International, Wallingford, UK. pp. 11-24.
Crossref |
|
Kramer M, Lund P, Weisbjerg MR (2013). Rumen Passage Kinetics of Forage and Concentrate Derived Fiber in Dairy Cows. J. Dairy Sci. 96(5):3163-3176.
Crossref |
|
Lin B, Wang JH, Lu Y, Liang Q, Liu JX (2013). In vitro rumen fermentation and methane production are influenced by active component of essential oils combined with fumarate. J. Anim. Physiol. Anim. Nutr. 97(1): 1-9.
Crossref |
|
Mills JAN, Dijkstra J, Bannink A, Cammell SB, Kebreab E, France J (2001). A mechanistic model of whole-tract digestion and methanogenesis in the lactating dairy cow: Model development, evaluation, and application. J. Anim. Sci. 79:1584-1597. |
|
Moss Angela R, Jean-Pierre J, Newbold J (2000). Methane production by ruminants: Its contribution to global warming (Review article). Ann Zootechn. 49:231-253.
Crossref |
|
Murphy MR, Baldwin RL, Koong LJ (1982). Estimation of stoichiometric parameters for rumen fermentation of roughage and concentrate diets. J. Anim. Sci. 55:411-421. |
|
Murray RM, Bryant AM, Leng RA (1976). Rates of production of methane in the rumen and large intestine of sheep. Br. J. Nutr. 36:1-14.
Crossref |
|
Narimani-Rad M, Habib AS, Mortaza KN, Alireza L (2012). Effect of sunflower oil supplementation on in in vitro fermentation patterns of forage based diets for ruminants. Bull. Environ. Pharmacol. Life Sci. 1(12): 73-77. |
|
Offner A, Sauvant D (2006). Thermodynamic modelling of ruminal fermentations. Anim. Res. 55:1-23.
Crossref |
|
Ogino A, Orito H, Shimadad K, Hirooka H (2007). Evaluating environmental impacts of the Japanese beef cow–calf system by the life cycle assessment method. Anim. Sci. J. 78:4240-432.
Crossref |
|
Pitt RE, Van Kessel JS, Fox DG, Pell AN, Barry MC, Van Soest PJ (1996). Prediction of ruminal volatile fatty acids and pH within the net carbohydrate and protein system. J. Anim. Sci. 74:226-244. |
|
Salminen E, Rintala J, Lokshina LYA, Vavilin VA (2000). Anaerobic batch degradation of solid poultry slaughterhouse waste. Water Sci. Technol. 41:33-41. |
|
Smink W, Pellikaan WF, Van der Kolk LJ, Van de, Hoek KW (2004). Methane production as a result from rumen fermentation in cattle calculated by using the IPCC-GPG Tier 2 method. FIS report, FS 04 12 E. |
|
Van Laar H, van Straalen WM (2004). Ontwikkeling van een rantsoen voor melkvee dat de methaanproductie reduceert. Schothorst Feed Reseatrch. |
|
Van Zijderveld S, van Straalen WM (2004). Validatie van de IPCC methaan conversiefactor vooromstandigheden waaronder Nederlands melkvee wordt gehouden. Schothorst Feed Research proefverslag BET. 2004-29. |